- STEM Ambassadors
- School trusts
- ITE and governors
- Invest in schools
- Student programmes
- Benefits and impact
- Our supporters
- Advertising and sponsorship
- Become a STEM Ambassador
- Request a STEM Ambassador
- Employer information
- Training and support
- STEM Ambassadors Partners
- Working with community groups
- Search icon
- Join the STEM Community

Homeostasis - kidneys
The kidneys and water balance is a nice topic to teach. Students have direct experience of the process and can observe changes depending on their own water intake. It is satisfying when students understand processes so directly related to their own physiology.
Part of the topic deals with kidney dialysis and transplants. Care should be taken in this area as some sensitive issues are touched upon. It is not beyond possibility that some class members may have direct experience through a family member or friend. Likewise, fatal accidents can often be the source of donor kidneys and this should be remembered.
Whilst this list provides a source of information and ideas for experimental work, it is important to note that recommendations can date very quickly. Do NOT follow suggestions which conflict with current advice from CLEAPSS, SSERC or recent safety guides. eLibrary users are responsible for ensuring that any activity, including practical work, which they carry out is consistent with current regulations related to Health and Safety and that they carry an appropriate risk assessment. Further information is provided in our Health and Safety guidance.
Homeostasis - kidneys and water balance
This is a web resource written specifically for 14-16 students. It can be used by students for self-study or revision. If used in the classroom, there are some useful animations that can be used with an interactive whiteboard.
Section 5 (How the kidney works) can be used to view the section on filtration and reabsorption.
Section 6 looks at ADH and the control of water balance and is a good way to illustrate how feedback loops control water in the body. The action of ADH can be somewhat confusing for students. It is counter-intuitive to some that the release of a hormone can actually make something happen less. That is, more ADH equals less urine produced. A description of more ADH causes more water to be conserved in the body may be helpful.
Section 8 (What is dialysis?) has a good explanation of the process. This, and section 9, can be used to produce a poster on the pros and cons of dialysis and transplants.
Let’s Dissect – the Kidney
Quality Assured Category: Science Publisher: University of Bristol
This animation and video show students the structure and function of the kidneys. It also describes dialysis and kidney transplants.
Students can use the animation as a self-study activity once the topic of the kidneys and their broad function has been introduced. Alternatively, it makes a good revision activity once the topic has been completed.
Depending on the detail required by your course specifications, students can be given a framework of headings so that they can focus onto the relevant sections of the animation. They could make their own notes on the topic, which will help them develop skills in interpreting and summarising information. Supply diagrams for annotation.
There is a good section on dialysis. Ask students to consider the dialysis solution and explain its composition.
A kidney dissection is readily carried out in the laboratory. The video shows how to carry out the procedure effectively, or alternatively it can be used in place of a dissection. It is worth viewing the video as it shows the blood vessels and ureter. These are normally missing kidneys obtained from a butcher.
With any dissection, it is beneficial to be sensitive to students’ feelings. Reassurance may be gained by informing students that the animal that the kidneys were taken from was not killed specifically for that purpose. Be aware of cultural objections to handling tissues from certain animals. It may be worth talking to students prior to the lesson so that any concerns can be raised and any permissions from parents granted.
If students genuinely do not want to be involved, they can have alternative activities arranged for them.

Kidneys *suitable for home teaching*
Quality Assured Category: Science Publisher: Gatsby Science Enhancement Programme
A Catalyst article which describes the structure and function of the kidneys. Students can read the whole article.
There is a good diagram which shows the structure of a kidney tubule and relates it to the processes of filtration and reabsorption. This is quite a tricky concept as students are told that kidneys ‘filter’ the blood. Intuitively, this is a one-way process. Reabsorption suggests something else.
Students can be challenged to write three stories that tell the routes through the kidney and beyond of: • a water molecule destined to be excreted from the body; • a molecule of urea (from excess protein); • a sugar molecule.
You can use these stories to check if the students have understood the process of filtration, followed by reabsorption.
One section of the article describes the control of urine production via ADH. Students could produce flow diagrams to illustrate the control pathways that happen when the body has too much, or too little water.
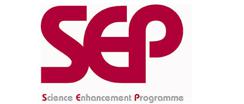
Interactive kidney animation
This animation allows students to see how different parts of the kidney work. The introduction and section on the nephron are at a suitable level. Sections on the Bowman’s capsule, loop of Henle, collecting duct and proximal tubule are a little too detailed but may be of interest to students as an extension activity.
Have students view the introduction.
The section on the nephron allows students to select different molecules and watch their progress through the nephron. Students can use their understanding of the kidney to predict where the different molecules will go. This could be as an individual activity, giving students 10 minutes to explore the animation.
As a whole class revision or summary activity, the animation could be displayed on an interactive whiteboard. Give students a choice of route options and have them vote to see which one they think is correct. This will help to gauge their level of understanding.
Kidney Failure
Quality Assured Category: Biology Publisher: Association for Science Education (ASE)
The activities in this resource take a closer look at renal dialysis, peritoneal dialysis and kidney transplants.
The first activities can be used to revise kidney structure and function. These activities need not be done they have recently been covered.
There is a good information sheet on dialysis and transplants. This can be used as the basis of a comprehension activity. Have students produce suitable questions and swap them amongst the class. These can then be peer marked. The answers can be discussed and misconceptions addressed.
A final activity looks at how transplant patients are chosen. This is a good activity to get students thinking about the broader implications of the procedure. How would they decide?
Remember to be sensitive to any class members, friends or family who may have kidney failure or indeed who may have been involved in the type of accident from which many donor tissues are obtained.
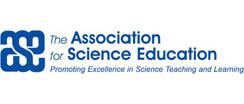
Keeping Healthy
Quality Assured Category: Science Publisher: University of York Science Education Group (UYSEG)
These materials contain a wide range of activities but the ones relating to the kidneys can be found in section 5, starting on page 60.
There are two good activities that help students to understand how the kidneys work and how dialysis works.
The first activity sees students modelling the filtration of the blood. Using a mesh or net, students see how large molecules and blood cells cannot be filtered whilst smaller solute molecules can be.
It is worth stressing where this process is happening in the kidney and also that the useful molecules are subsequently reabsorbed.
A second activity uses Visking tubing and a sample of made up ‘blood’ to model dialysis. This will help students to get a grasp of a process that they would otherwise be unable to view. It will help to make it less abstract. A subsequent activity focusses onto what happens in a renal dialysis machine.
Students’ understanding of dialysis can be tested at this point. In the experiment, glucose and salt enter the dialysis fluid. This would then be discarded. How does this compare to a real dialysis machine? What is the composition of the dialysis fluid? How is it adjusted to make sure that useful substances like glucose and salt are not taken out of the blood? After all, the body needs to keep these. It is just the urea that the dialysis machine is designed to remove. This leads to a good discussion about diffusion and concentration gradients.
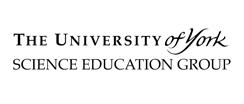
Kidney Function
- Cardiovascular System
Resource Type
Description.
This video explores how the kidneys filter blood to remove wastes and excess fluid from the body, maintaining a healthy balance of water and salts in the blood.
Physician Richard Lifton explains that as fluids (mostly water) from blood move through the kidney, almost all of the water, along with salts and nutrients the body needs, are reabsorbed by the blood. The remaining fluid and wastes become urine.
Lifton uses a fish tank being filled with water and salt to demonstrate how much liquid the kidney filters in a day: about 170 liters, of which only about 1 liter ends up in the urine. He also uses a 3D model to illustrate anatomical components of the kidney, including the nephron, the proximal tubule, the renal medulla, the loop of Henle, the distal convoluted tubule, and the cortical collecting duct.
This clip is from a 1998 Holiday Lecture Series, Of Hearts and Hypertension: Blazing Genetic Traits .
anatomy, circulation, demonstration, filtration, homeostasis, ion regulation, mineral, nephron, salt, urine
Terms of Use
Please see the Terms of Use for information on how this resource can be used.
Version History
Explore related content, other related resources.

Chapter 9: The Urinary System
The urinary system and homeostasis – water and electrolyte balance., water balance.
On a typical day, the average adult will take in about 2500 mL (almost 3 quarts) of aqueous fluids. Although most of the intake comes through the digestive tract, about 230 mL (8 ounces) per day is generated metabolically, in the last steps of aerobic respiration. Additionally, each day about the same volume (2500 mL) of water leaves the body by different routes; most of this lost water is removed as urine. The kidneys also can adjust blood volume though mechanisms that draw water out of the filtrate and urine. The kidneys can regulate water levels in the body; they conserve water if you are dehydrated, and they can make urine more dilute to expel excess water if necessary. Water is lost through the skin through evaporation from the skin surface without overt sweating and from air expelled from the lungs. This type of water loss is called insensible water loss because a person is usually unaware of it.
Regulation of Water Intake: Osmolality is the ratio of solutes in a solution to a volume of solvent in a solution. Plasma osmolality is thus the ratio of solutes to water in blood plasma. A person’s plasma osmolality value reflects his or her state of hydration. A healthy body maintains plasma osmolality within a narrow range, by employing several mechanisms that regulate both water intake and output.
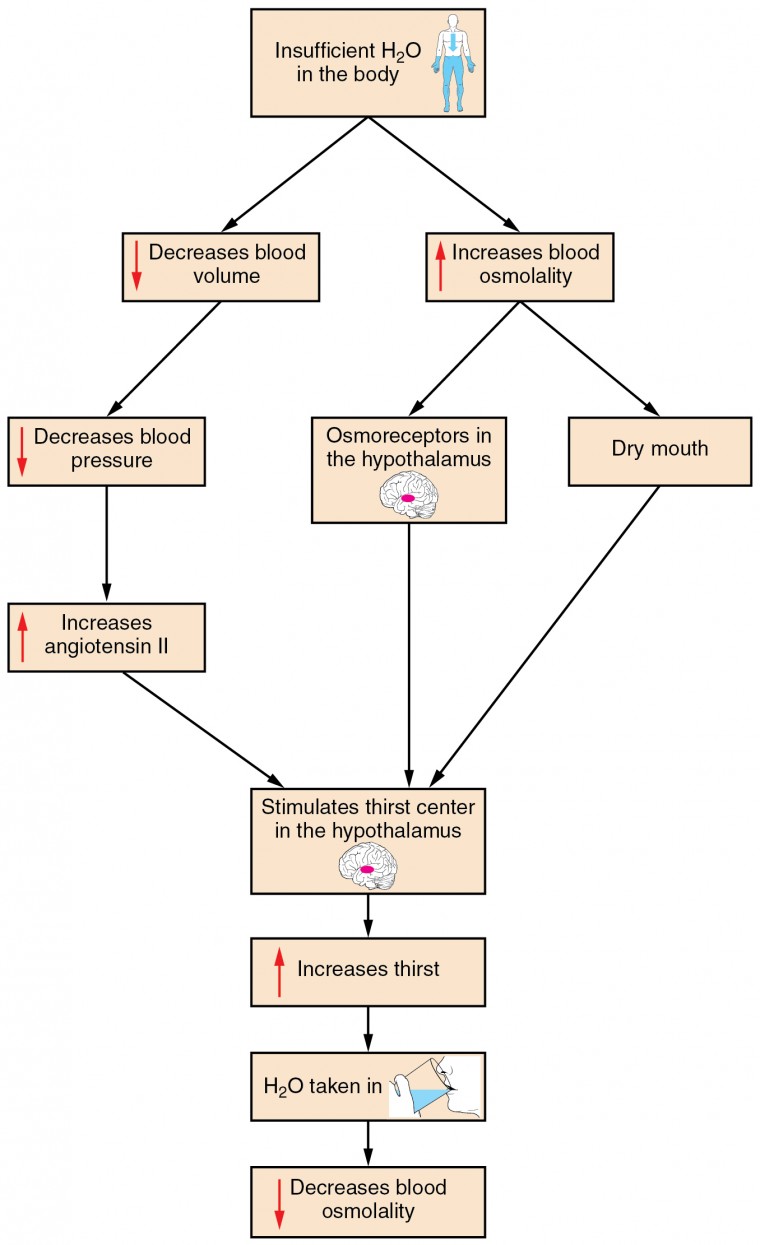
Figure 1. Click to view a larger image. The thirst response begins when osmoreceptors detect a decrease in water levels in the blood.
Drinking water is considered voluntary. So how is water intake regulated by the body? Consider someone who is experiencing dehydration , a net loss of water that results in insufficient water in blood and other tissues. The water that leaves the body, as exhaled air, sweat, or urine, is ultimately extracted from blood plasma. As the blood becomes more concentrated, the thirst response—a sequence of physiological processes—is triggered. Osmoreceptors are sensory receptors in the thirst center in the hypothalamus that monitor the concentration of solutes (osmolality) of the blood. If blood osmolality increases above its ideal value, the hypothalamus transmits signals that result in a conscious awareness of thirst. The person should (and normally does) respond by drinking water. The hypothalamus of a dehydrated person also releases antidiuretic hormone (ADH) through the posterior pituitary gland. ADH signals the kidneys to recover water from urine, effectively diluting the blood plasma. To conserve water, the hypothalamus of a dehydrated person also sends signals via the sympathetic nervous system to the salivary glands in the mouth. The signals result in a decrease in watery, serous output (and an increase in stickier, thicker mucus output). These changes in secretions result in a “dry mouth” and the sensation of thirst.
Decreased blood volume resulting from water loss has two additional effects. First, baroreceptors, blood-pressure receptors in the arch of the aorta and the carotid arteries in the neck, detect a decrease in blood pressure that results from decreased blood volume. The heart is ultimately signaled to increase its rate and/or strength of contractions to compensate for the lowered blood pressure.
Second, the kidneys have a renin-angiotensin hormonal system that increases the production of the active form of the hormone angiotensin II, which helps stimulate thirst, but also stimulates the release of the hormone aldosterone from the adrenal glands. Aldosterone increases the reabsorption of sodium in the distal tubules of the nephrons in the kidneys, and water follows this reabsorbed sodium back into the blood.
If adequate fluids are not consumed, dehydration results and a person’s body contains too little water to function correctly. A person who repeatedly vomits or who has diarrhea may become dehydrated, and infants, because their body mass is so low, can become dangerously dehydrated very quickly. Endurance athletes such as distance runners often become dehydrated during long races. Dehydration can be a medical emergency, and a dehydrated person may lose consciousness, become comatose, or die, if his or her body is not rehydrated quickly.
Regulation of Water Output
Water loss from the body occurs predominantly through the renal system. A person produces an average of 1.5 liters (1.6 quarts) of urine per day. Although the volume of urine varies in response to hydration levels, there is a minimum volume of urine production required for proper bodily functions. The kidney excretes 100 to 1200 milliosmoles of solutes per day to rid the body of a variety of excess salts and other water-soluble chemical wastes, most notably creatinine, urea, and uric acid. Failure to produce the minimum volume of urine means that metabolic wastes cannot be effectively removed from the body, a situation that can impair organ function. The minimum level of urine production necessary to maintain normal function is about 0.47 liters (0.5 quarts) per day. The kidneys also must make adjustments in the event of ingestion of too much fluid. Diuresis , which is the production of urine in excess of normal levels, begins about 30 minutes after drinking a large quantity of fluid. Diuresis reaches a peak after about 1 hour, and normal urine production is reestablished after about 3 hours.
Role of ADH
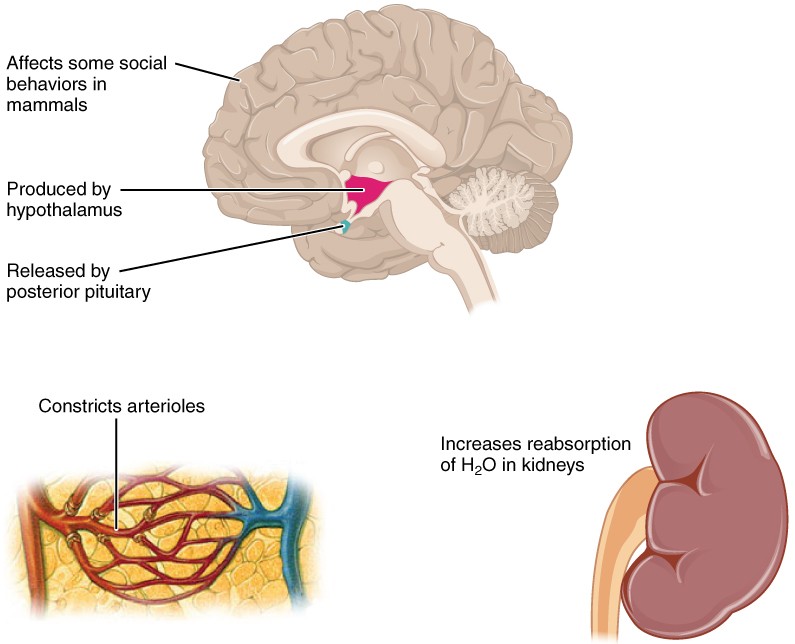
Figure 2. ADH is produced in the hypothalamus and released by the posterior pituitary gland. It causes the kidneys to retain water, constricts arterioles in the peripheral circulation, and affects some social behaviors in mammals.
Antidiuretic hormone (ADH) , also known as vasopressin, controls the amount of water reabsorbed from the collecting ducts and tubules in the kidney. This hormone is produced in the hypothalamus and is delivered to the posterior pituitary for storage and release (Figure 2.). When the osmoreceptors in the hypothalamus detect an increase in the concentration of blood plasma, the hypothalamus signals the release of ADH from the posterior pituitary into the blood.
ADH has two major effects. It constricts the arterioles in the peripheral circulation, which reduces the flow of blood to the extremities and thereby increases the blood supply to the core of the body. ADH also causes the epithelial cells that line the renal collecting tubules to move water channel proteins, called aquaporins, from the interior of the cells to the apical surface, where these proteins are inserted into the cell membrane. The result is an increase in the water permeability of these cells and, thus, a large increase in water passage from the urine through the walls of the collecting tubules, leading to more reabsorption of water into the bloodstream. When the blood plasma becomes less concentrated and the level of ADH decreases, aquaporins are removed from collecting tubule cell membranes, and the passage of water out of urine and into the blood decreases.
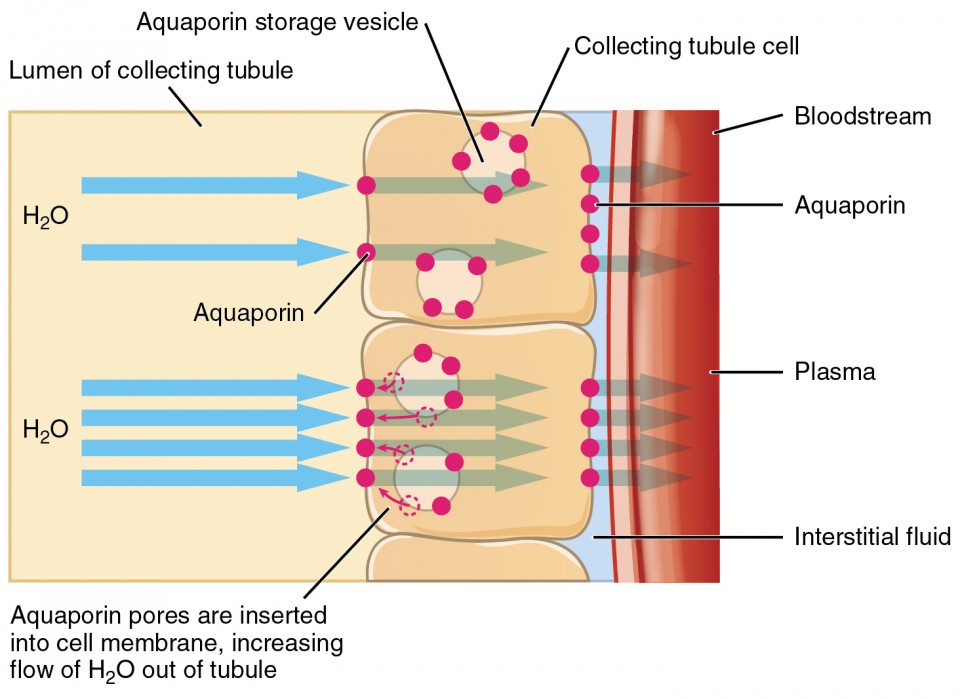
Figure 3. The binding of ADH to receptors on the cells of the collecting tubule results in aquaporins being inserted into the plasma membrane, shown in the lower cell. This dramatically increases the flow of water out of the tubule and into the bloodstream.
A diuretic is a compound that increases urine output and therefore decreases water conservation by the body. Diuretics are used to treat hypertension, congestive heart failure, and fluid retention associated with menstruation. Alcohol acts as a diuretic by inhibiting the release of ADH. Additionally, caffeine, when consumed in high concentrations, acts as a diuretic.
Electrolyte balance
The body contains a large variety of ions, or electrolytes, which perform a variety of functions. Some ions assist in the transmission of electrical impulses along cell membranes in neurons and muscles. Other ions help to stabilize protein structures in enzymes. Still others aid in releasing hormones from endocrine glands. All of the ions in plasma contribute to the osmotic balance that controls the movement of water between cells and their environment.
Electrolytes in living systems include sodium, potassium, chloride, bicarbonate, calcium, phosphate, magnesium, copper, zinc, iron, manganese, molybdenum, copper, and chromium. In terms of body functioning, six electrolytes are most important: sodium, potassium, chloride, bicarbonate, calcium, and phosphate. These six ions aid in nerve excitability, endocrine secretion, membrane permeability, buffering body fluids, and controlling the movement of fluids between compartments.
Excretion of ions occurs mainly through the kidneys, with lesser amounts lost in sweat and in feces. Excessive sweating may cause a significant loss, especially of sodium and chloride. Severe vomiting or diarrhea will cause a loss of chloride and bicarbonate ions. Adjustments in respiratory and renal functions allow the body to regulate the levels of these ions in the ECF.
The following table lists the reference values for blood plasma, cerebrospinal fluid (CSF), and urine for the six ions addressed in this section. In a clinical setting, sodium, potassium, and chloride are typically analyzed in a routine urine sample. In contrast, calcium and phosphate analysis requires a collection of urine across a 24-hour period, because the output of these ions can vary considerably over the course of a day. Urine values reflect the rates of excretion of these ions. Bicarbonate is the one ion that is not normally excreted in urine; instead, it is conserved by the kidneys for use in the body’s buffering systems.
Table 1. Electrolyte and Ion Reference Values | ||||
---|---|---|---|---|
Name | Chemical symbol | Plasma | CSF | Urine |
Sodium | Na | 136.00–146.00 (mM) | 138.00–150.00 (mM) | 40.00–220.00 (mM) |
Potassium | K | 3.50–5.00 (mM) | 0.35–3.5 (mM) | 25.00–125.00 (mM) |
Chloride | Cl | 98.00–107.00 (mM) | 118.00–132.00 (mM) | 110.00–250.00 (mM) |
Bicarbonate | HCO | 22.00–29.00 (mM) | —— | —— |
Calcium | Ca | 2.15–2.55 (mmol/day) | —— | Up to 7.49 (mmol/day) |
Phosphate | PO4 | 0.81–1.45 (mmol/day) | —— | 12.90–42.00 (mmol/day) |
Imbalances of these ions can result in various problems in the body, and their concentrations are tightly regulated. Aldosterone and angiotensin II control the exchange of sodium and potassium between the renal filtrate and the renal collecting tubule. Calcium and phosphate are regulated by PTH, calcitrol, and calcitonin.
Sodium is the major cation of the extracellular fluid. It is responsible for one-half of the osmotic pressure gradient that exists between the interior of cells and their surrounding environment. People eating a typical Western diet, which is very high in NaCl, routinely take in 130 to 160 mmol/day of sodium, but humans require only 1 to 2 mmol/day. This excess sodium appears to be a major factor in hypertension (high blood pressure) in some people. Excretion of sodium is accomplished primarily by the kidneys. Sodium is freely filtered through the glomerular capillaries of the kidneys, and although much of the filtered sodium is reabsorbed in the proximal convoluted tubule, some remains in the filtrate and urine, and is normally excreted.
Hyponatremia is a lower-than-normal concentration of sodium, usually associated with excess water accumulation in the body, which dilutes the sodium. An abnormal loss of sodium from the body can result from several conditions, including excessive sweating, vomiting, or diarrhea; the use of diuretics; excessive production of urine, which can occur in diabetes; and acidosis, either metabolic acidosis or diabetic ketoacidosis. At the cellular level, hyponatremia results in increased entry of water into cells by osmosis, because the concentration of solutes within the cell exceeds the concentration of solutes in the now-diluted ECF. The excess water causes swelling of the cells; the swelling of red blood cells—decreasing their oxygen-carrying efficiency and making them potentially too large to fit through capillaries—along with the swelling of neurons in the brain can result in brain damage or even death. Hypernatremia is an abnormal increase of blood sodium. It can result from water loss from the blood. Hormonal imbalances involving ADH and aldosterone may also result in higher-than-normal sodium values.
Potassium is the major intracellular cation. It helps establish the resting membrane potential in neurons and muscle fibers after membrane depolarization and action potentials. In contrast to sodium, potassium has very little effect on osmotic pressure.
Hypokalemia is an abnormally low potassium blood level. Similar to the situation with hyponatremia, hypokalemia can occur because of either an absolute reduction of potassium in the body or a relative reduction of potassium in the blood due to the redistribution of potassium. An absolute loss of potassium can arise from decreased intake, frequently related to starvation. It can also come about from vomiting, diarrhea, or alkalosis. Hyperkalemia , an elevated potassium blood level, also can impair the function of skeletal muscles, the nervous system, and the heart. Hyperkalemia can result from increased dietary intake of potassium. In such a situation, potassium from the blood ends up in the ECF in abnormally high concentrations. This can result in a partial depolarization (excitation) of the plasma membrane of skeletal muscle fibers, neurons, and cardiac cells of the heart, and can also lead to an inability of cells to repolarize. For the heart, this means that it won’t relax after a contraction, and will effectively “seize” and stop pumping blood, which is fatal within minutes. Because of such effects on the nervous system, a person with hyperkalemia may also exhibit mental confusion, numbness, and weakened respiratory muscles.
Chloride is the predominant extracellular anion. Chloride is a major contributor to the osmotic pressure gradient between the ICF and ECF, and plays an important role in maintaining proper hydration.
Hypochloremia , or lower-than-normal blood chloride levels, can occur because of defective renal tubular absorption. Vomiting, diarrhea, and metabolic acidosis can also lead to hypochloremia. Hyperchloremia , or higher-than-normal blood chloride levels, can occur due to dehydration, excessive intake of dietary salt (NaCl) or swallowing of sea water, aspirin intoxication, congestive heart failure, and the hereditary, chronic lung disease, cystic fibrosis. In people who have cystic fibrosis, chloride levels in sweat are two to five times those of normal levels, and analysis of sweat is often used in the diagnosis of the disease.
Bicarbonate
Bicarbonate is the second most abundant anion in the blood. Its principal function is to maintain your body’s acid-base balance by being part of buffer systems.
About two pounds of calcium in your body are bound up in bone, which provides hardness to the bone and serves as a mineral reserve for calcium and its salts for the rest of the tissues. Teeth also have a high concentration of calcium within them. A little more than one-half of blood calcium is bound to proteins, leaving the rest in its ionized form. Calcium ions, Ca 2+ , are necessary for muscle contraction, enzyme activity, and blood coagulation. In addition, calcium helps to stabilize cell membranes and is essential for the release of neurotransmitters from neurons and of hormones from endocrine glands. Calcium is absorbed through the intestines under the influence of activated vitamin D. A deficiency of vitamin D leads to a decrease in absorbed calcium and, eventually, a depletion of calcium stores from the skeletal system, potentially leading to rickets in children and osteomalacia in adults, contributing to osteoporosis.
Hypocalcemia , or abnormally low calcium blood levels, is seen in hypoparathyroidism, which may follow the removal of the thyroid gland, because the four nodules of the parathyroid gland are embedded in it. Hypercalcemia , or abnormally high calcium blood levels, is seen in primary hyperparathyroidism. Some malignancies may also result in hypercalcemia.
Bone and teeth bind up 85 percent of the body’s phosphate as part of calcium-phosphate salts. Phosphate is found in phospholipids, such as those that make up the cell membrane, and in ATP, nucleotides, and buffers.
Hypophosphatemia , or abnormally low phosphate blood levels, occurs with heavy use of antacids, during alcohol withdrawal, and during malnourishment. In the face of phosphate depletion, the kidneys usually conserve phosphate, but during starvation, this conservation is impaired greatly. Hyperphosphatemia , or abnormally increased levels of phosphates in the blood, occurs if there is decreased renal function or in cases of acute lymphocytic leukemia. Additionally, because phosphate is a major constituent of the ICF, any significant destruction of cells can result in dumping of phosphate into the ECF.
Regulation of Sodium and Potassium
Sodium is reabsorbed from the renal filtrate, and potassium is excreted into the filtrate in the renal collecting tubule. The control of this exchange is governed principally by two hormones—aldosterone and angiotensin II.
Aldosterone
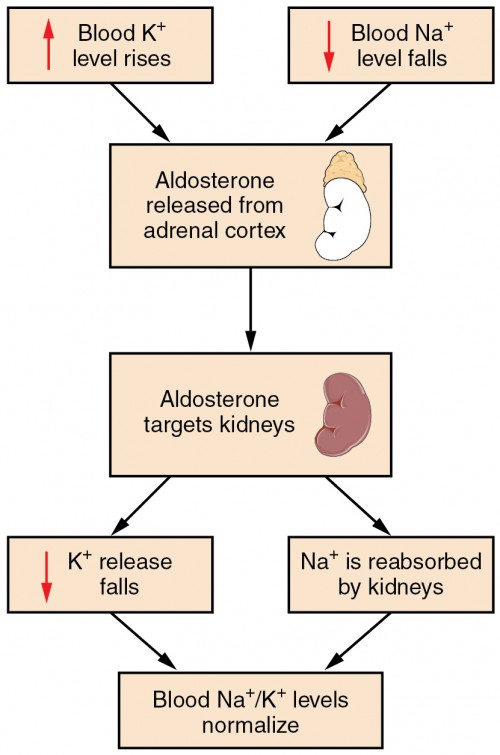
Figure 4. Aldosterone, which is released by the adrenal gland, facilitates reabsorption of Na + and thus the reabsorption of water.
Recall that aldosterone increases the excretion of potassium and the reabsorption of sodium in the distal tubule. Aldosterone is released if blood levels of potassium increase, if blood levels of sodium severely decrease, or if blood pressure decreases. Its net effect is to conserve and increase water levels in the plasma by reducing the excretion of sodium, and thus water, from the kidneys. In a negative feedback loop, increased osmolality of the ECF (which follows aldosterone-stimulated sodium absorption) inhibits the release of the hormone.
Angiotensin II
Angiotensin II causes vasoconstriction and an increase in systemic blood pressure. This action increases the glomerular filtration rate, resulting in more material filtered out of the glomerular capillaries and into Bowman’s capsule. Angiotensin II also signals an increase in the release of aldosterone from the adrenal cortex.
In the distal convoluted tubules and collecting ducts of the kidneys, aldosterone stimulates the synthesis and activation of the sodium-potassium pump. Sodium passes from the filtrate, into and through the cells of the tubules and ducts, into the ECF and then into capillaries. Water follows the sodium due to osmosis. Thus, aldosterone causes an increase in blood sodium levels and blood volume. Aldosterone’s effect on potassium is the reverse of that of sodium; under its influence, excess potassium is pumped into the renal filtrate for excretion from the body.
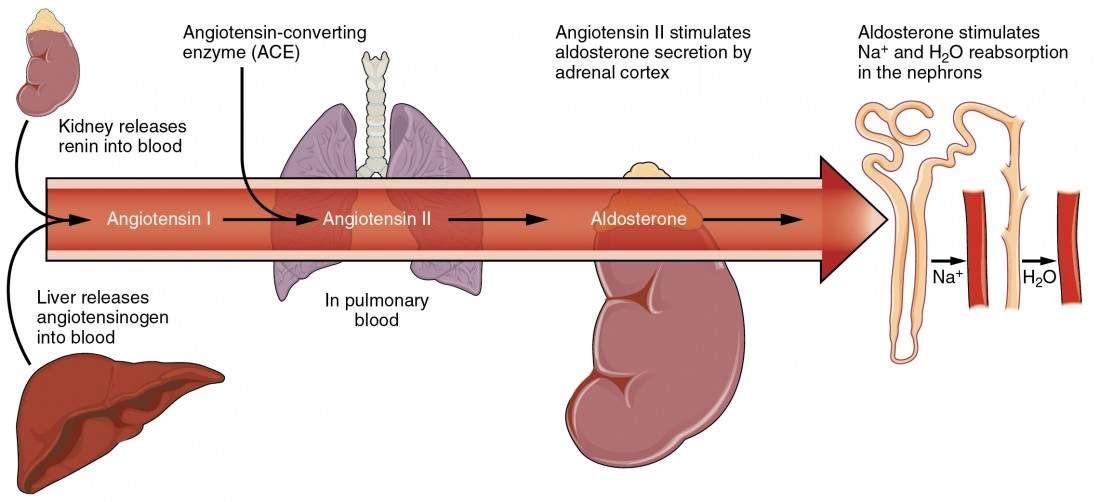
Figure 5. Angiotensin II stimulates the release of aldosterone from the adrenal cortex.
Regulation of Calcium and Phosphate
Calcium and phosphate are both regulated through the actions of three hormones: parathyroid hormone (PTH), dihydroxyvitamin D (calcitriol), and calcitonin . All three are released or synthesized in response to the blood levels of calcium.
PTH is released from the parathyroid gland in response to a decrease in the concentration of blood calcium. The hormone activates osteoclasts to break down bone matrix and release inorganic calcium-phosphate salts. PTH also increases the gastrointestinal absorption of dietary calcium by converting vitamin D into dihydroxyvitamin D (calcitriol), an active form of vitamin D that intestinal epithelial cells require to absorb calcium.
PTH raises blood calcium levels by inhibiting the loss of calcium through the kidneys. PTH also increases the loss of phosphate through the kidneys.
Calcitonin is released from the thyroid gland in response to elevated blood levels of calcium. The hormone increases the activity of osteoblasts, which remove calcium from the blood and incorporate calcium into the bony matrix.
Blood Pressure Regulation
Due to osmosis, water follows where Na + leads. Much of the water the kidneys recover from the forming urine follows the reabsorption of Na + . ADH stimulation of aquaporin channels allows for regulation of water recovery in the collecting ducts. Normally, all of the glucose is recovered, but loss of glucose control (diabetes mellitus) may result in an osmotic dieresis severe enough to produce severe dehydration and death. A loss of renal function means a loss of effective vascular volume control, leading to hypotension (low blood pressure) or hypertension (high blood pressure), which can lead to stroke, heart attack, and aneurysm formation.
Regulation of Osmolarity
Blood pressure and osmolarity are regulated in a similar fashion. Severe hypo-osmolarity can cause problems like lysis (rupture) of blood cells or widespread edema, which is due to a solute imbalance. Inadequate solute concentration (such as protein) in the plasma results in water moving toward an area of greater solute concentration, in this case, the interstitial space and cell cytoplasm. If the kidney glomeruli are damaged by an autoimmune illness, large quantities of protein may be lost in the urine. The resultant drop in serum osmolarity leads to widespread edema that, if severe, may lead to damaging or fatal brain swelling. Severe hypertonic conditions may arise with severe dehydration from lack of water intake, severe vomiting, or uncontrolled diarrhea. When the kidney is unable to recover sufficient water from the forming urine, the consequences may be severe (lethargy, confusion, muscle cramps, and finally, death) .
pH Regulation
Proper kidney function is essential for pH homeostasis.
Vitamin D Synthesis
In order for vitamin D to become active, it must undergo a hydroxylation reaction in the kidney, that is, an –OH group must be added to calcidiol to make calcitriol (1,25-dihydroxycholecalciferol). Activated vitamin D is important for absorption of Ca ++ in the digestive tract, its reabsorption in the kidney, and the maintenance of normal serum concentrations of Ca ++ and phosphate. Calcium is vitally important in bone health, muscle contraction, hormone secretion, and neurotransmitter release. Inadequate Ca ++ leads to disorders like osteoporosis and osteomalacia in adults and rickets in children. Deficits may also result in problems with cell proliferation, neuromuscular function, blood clotting, and the inflammatory response. Recent research has confirmed that vitamin D receptors are present in most, if not all, cells of the body, reflecting the systemic importance of vitamin D. Many scientists have suggested it be referred to as a hormone rather than a vitamin.
Erythropoiesis: control by EPO hormone.
EPO is a 193-amino acid protein that stimulates the formation of red blood cells in the bone marrow. The kidney produces 85 percent of circulating EPO; the liver, the remainder. If you move to a higher altitude, the partial pressure of oxygen is lower, meaning there is less pressure to push oxygen across the alveolar membrane and into the red blood cell. One way the body compensates is to manufacture more red blood cells by increasing EPO production. If you start an aerobic exercise program, your tissues will need more oxygen to cope, and the kidney will respond with more EPO. If erythrocytes are lost due to severe or prolonged bleeding, or under produced due to disease or severe malnutrition, the kidneys come to the rescue by producing more EPO. Renal failure (loss of EPO production) is associated with anemia, which makes it difficult for the body to cope with increased oxygen demands or to supply oxygen adequately even under normal conditions. Anemia diminishes performance and can be life threatening.
- Anatomy & Physiology. Provided by : OpenStax CNX. Located at : http://cnx.org/contents/[email protected] . License : CC BY: Attribution . License Terms : Download for free at http://cnx.org/contents/[email protected]
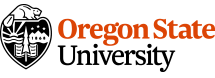
26.2 Water Balance
Learning objectives.
By the end of this section, you will be able to:
- Explain how water levels in the body influence the thirst cycle
- Identify the main route by which water leaves the body
- Describe the role of ADH and aldosterone and their effect on body water levels
- Define dehydration and identify common causes of dehydration
On a typical day, the average adult will take in about 2500 mL (almost 3 quarts) of aqueous fluids. Although most of the intake comes through the digestive tract, about 230 mL (8 ounces) per day is generated metabolically, in the last steps of aerobic respiration. Additionally, each day about the same volume (2500 mL) of water leaves the body by different routes; most of this lost water is removed as urine. The kidneys also can adjust blood volume though mechanisms that draw water out of the filtrate and urine. The kidneys can regulate water levels in the body; they conserve water if you are dehydrated, and they can make urine more dilute to expel excess water if necessary. Water is lost through the skin through evaporation from the skin surface without overt sweating and from air expelled from the lungs. This type of water loss is called insensible water loss because a person is usually unaware of it.
Regulation of Water Intake
Osmolality is the ratio of solutes in a solution to a volume of solvent in a solution. Plasma osmolality is thus the ratio of solutes to water in blood plasma. A person’s plasma osmolality value reflects his or her state of hydration. A healthy body maintains plasma osmolality within a narrow range, by employing several mechanisms that regulate both water intake and output.
Drinking water is considered voluntary. So how is water intake regulated by the body? Consider someone who is experiencing dehydration , a net loss of water that results in insufficient water in blood and other tissues. The water that leaves the body, as exhaled air, sweat, or urine, is ultimately extracted from blood plasma. As the blood becomes more concentrated, the thirst response—a sequence of physiological processes—is triggered ( Figure 26.2.1 ). Osmoreceptors are sensory receptors in the thirst center in the hypothalamus that monitor the concentration of solutes (osmolality) of the blood. If blood osmolality increases above its ideal value, the hypothalamus transmits signals that result in a conscious awareness of thirst. The person should (and normally does) respond by drinking water. The hypothalamus of a dehydrated person also releases antidiuretic hormone (ADH) through the posterior pituitary gland. ADH signals the kidneys to recover water from urine, effectively diluting the blood plasma. To conserve water, the hypothalamus of a dehydrated person also sends signals via the sympathetic nervous system to the salivary glands in the mouth. The signals result in a decrease in watery, serous output (and an increase in stickier, thicker mucus output). These changes in secretions result in a “dry mouth” and the sensation of thirst.
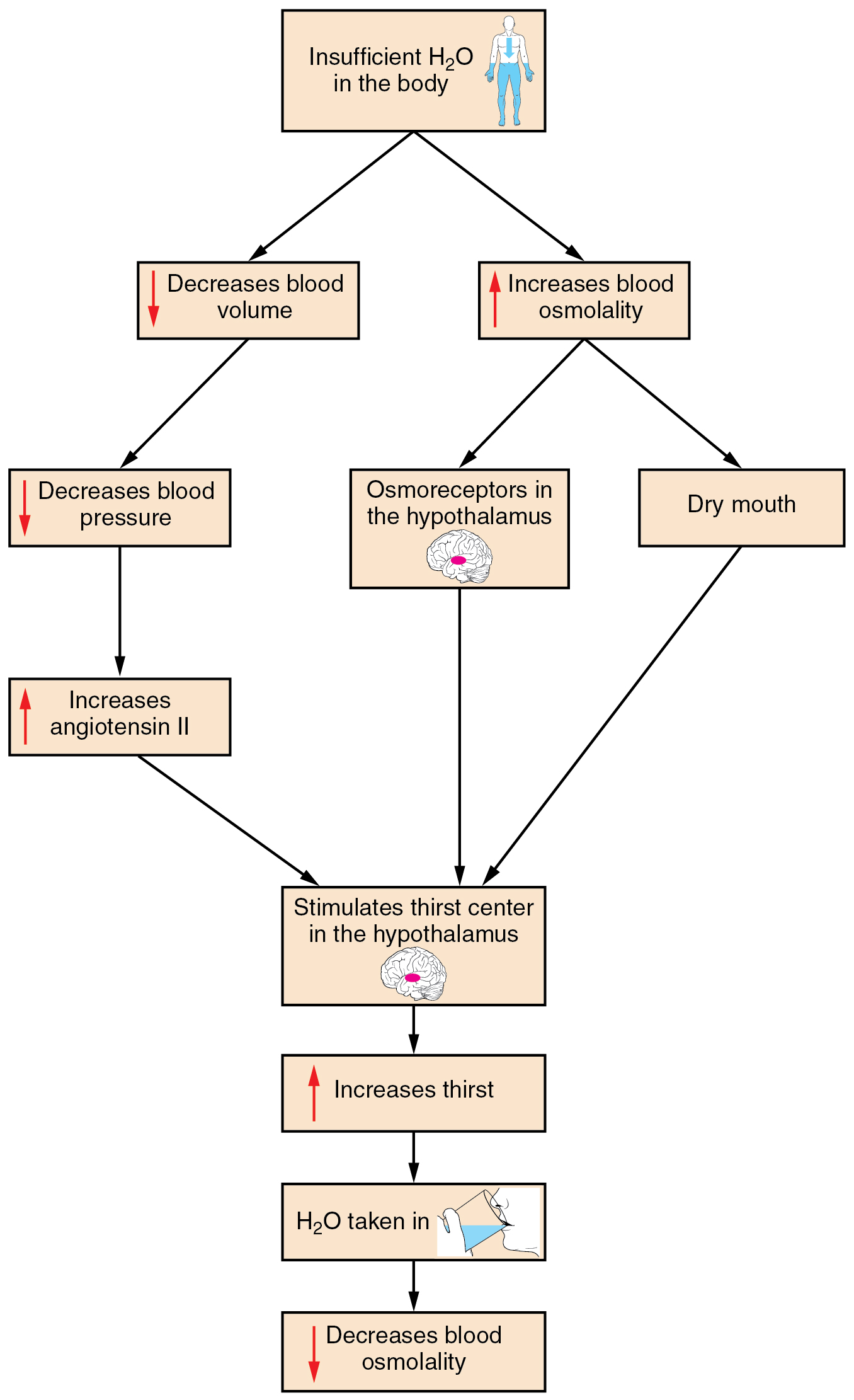
Decreased blood volume resulting from water loss has two additional effects. First, baroreceptors, blood-pressure receptors in the arch of the aorta and the carotid arteries in the neck, detect a decrease in blood pressure that results from decreased blood volume. The heart is ultimately signaled to increase its rate and/or strength of contractions to compensate for the lowered blood pressure.
Second, the kidneys have a renin-angiotensin hormonal system that increases the production of the active form of the hormone angiotensin II, which helps stimulate thirst, but also stimulates the release of the hormone aldosterone from the adrenal glands. Aldosterone increases the reabsorption of sodium in the distal tubules of the nephrons in the kidneys, and water follows this reabsorbed sodium back into the blood. Circulating angiotensin II can also stimulate the hypothalamus to release ADH.
If adequate fluids are not consumed, dehydration results and a person’s body contains too little water to function correctly. A person who repeatedly vomits or who has diarrhea may become dehydrated, and infants, because their body mass is so low, can become dangerously dehydrated very quickly. Endurance athletes such as distance runners often become dehydrated during long races. Dehydration can be a medical emergency, and a dehydrated person may lose consciousness, become comatose, or die, if his or her body is not rehydrated quickly.
Regulation of Water Output
Water loss from the body occurs predominantly through the renal system. A person produces an average of 1.5 liters (1.6 quarts) of urine per day. Although the volume of urine varies in response to hydration levels, there is a minimum volume of urine production required for proper bodily functions. The kidney excretes 100 to 1200 milliosmoles of solutes per day to rid the body of a variety of excess salts and other water-soluble chemical wastes, most notably creatinine, urea, and uric acid. Failure to produce the minimum volume of urine means that metabolic wastes cannot be effectively removed from the body, a situation that can impair organ function. The minimum level of urine production necessary to maintain normal function is about 0.47 liters (0.5 quarts) per day.
The kidneys also must make adjustments in the event of ingestion of too much fluid. Diuresis , which is the production of urine in excess of normal levels, begins about 30 minutes after drinking a large quantity of fluid. Diuresis reaches a peak after about 1 hour, and normal urine production is reestablished after about 3 hours.
Role of ADH
Antidiuretic hormone (ADH) , also known as vasopressin, controls the amount of water reabsorbed from the collecting ducts and tubules in the kidney. This hormone is produced in the hypothalamus and is delivered to the posterior pituitary for storage and release ( Figure 26.2.2 ). When the osmoreceptors in the hypothalamus detect an increase in the concentration of blood plasma, the hypothalamus signals the release of ADH from the posterior pituitary into the blood.
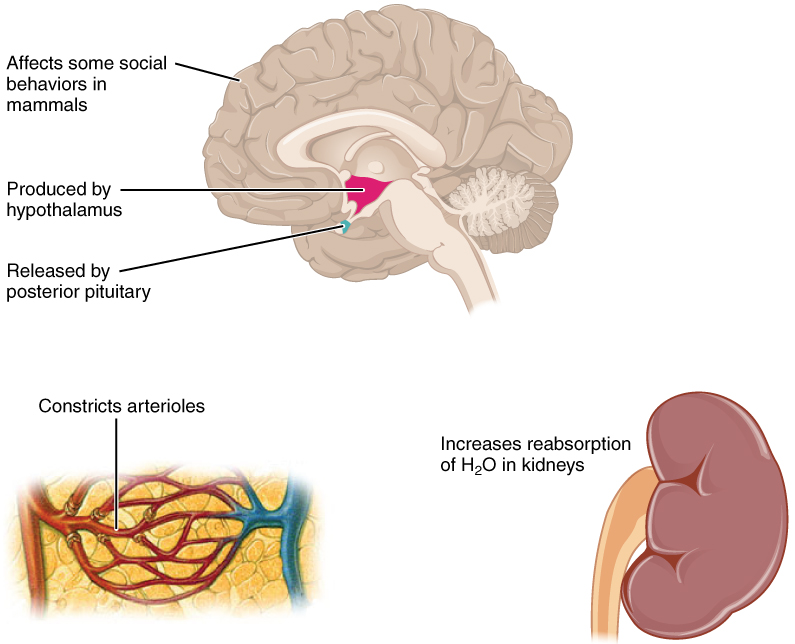
ADH has two major effects. It constricts the arterioles in the peripheral circulation, which reduces the flow of blood to the extremities and thereby increases the blood supply to the core of the body. ADH also causes the epithelial cells that line the renal collecting tubules to move water channel proteins, called aquaporins, from the interior of the cells to the apical surface, where these proteins are inserted into the cell membrane ( Figure 26.2.3 ). The result is an increase in the water permeability of these cells and, thus, a large increase in water passage from the urine through the walls of the collecting tubules, leading to more reabsorption of water into the bloodstream. When the blood plasma becomes less concentrated and the level of ADH decreases, aquaporins are removed from collecting tubule cell membranes, and the passage of water out of urine and into the blood decreases.
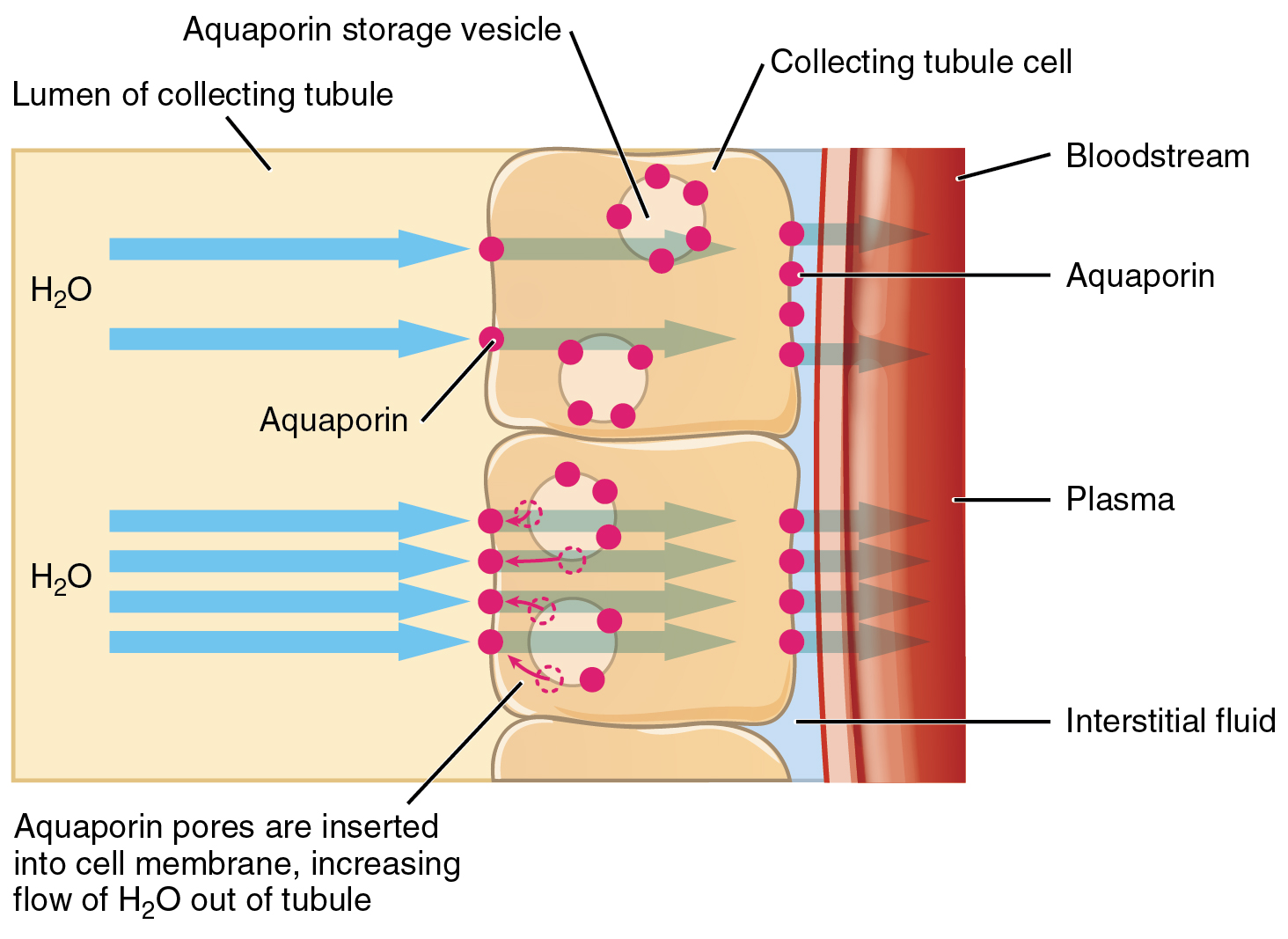
A diuretic is a compound that increases urine output and therefore decreases water conservation by the body. Diuretics are used to treat hypertension, congestive heart failure, and fluid retention associated with menstruation. Alcohol acts as a diuretic by inhibiting the release of ADH. Additionally, caffeine, when consumed in high concentrations, acts as a diuretic.
Chapter Review
Homeostasis requires that water intake and output be balanced. Most water intake comes through the digestive tract via liquids and food, but roughly 10 percent of water available to the body is generated at the end of aerobic respiration during cellular metabolism. Urine produced by the kidneys accounts for the largest amount of water leaving the body. The kidneys can adjust the concentration of the urine to reflect the body’s water needs, conserving water if the body is dehydrated or making urine more dilute to expel excess water when necessary. ADH is a hormone that helps the body to retain water by increasing water reabsorption by the kidneys.
Review Questions
Critical thinking questions.
1. Describe the effect of ADH on renal collecting tubules.
2. Why is it important for the amount of water intake to equal the amount of water output?
Answers for Critical Thinking Questions
- ADH constricts the arterioles in the peripheral circulation, limiting blood to the extremities and increasing the blood supply to the core of the body. ADH also causes the epithelial cells lining the renal collecting tubules to move water channel proteins called aquaporins from the sides of the cells to the apical surface. This greatly increases the passage of water from the renal filtrate through the wall of the collecting tubule as well as the reabsorption of water into the bloodstream.
- Any imbalance of water entering or leaving the body will create an osmotic imbalance that will adversely affect cell and tissue function.
This work, Anatomy & Physiology, is adapted from Anatomy & Physiology by OpenStax , licensed under CC BY . This edition, with revised content and artwork, is licensed under CC BY-SA except where otherwise noted.
Images, from Anatomy & Physiology by OpenStax , are licensed under CC BY except where otherwise noted.
Access the original for free at https://openstax.org/books/anatomy-and-physiology/pages/1-introduction .
Anatomy & Physiology Copyright © 2019 by Lindsay M. Biga, Staci Bronson, Sierra Dawson, Amy Harwell, Robin Hopkins, Joel Kaufmann, Mike LeMaster, Philip Matern, Katie Morrison-Graham, Kristen Oja, Devon Quick, Jon Runyeon, OSU OERU, and OpenStax is licensed under a Creative Commons Attribution-ShareAlike 4.0 International License , except where otherwise noted.
FREE K-12 standards-aligned STEM
curriculum for educators everywhere!
Find more at TeachEngineering.org .
- TeachEngineering
- Kidney Filtering
Hands-on Activity Kidney Filtering
Grade Level: 5 (3-5)
Time Required: 45 minutes
Expendable Cost/Group: US $3.00
Group Size: 4
Activity Dependency: None
Subject Areas: Biology
Curriculum in this Unit Units serve as guides to a particular content or subject area. Nested under units are lessons (in purple) and hands-on activities (in blue). Note that not all lessons and activities will exist under a unit, and instead may exist as "standalone" curriculum.
- Walk, Run, Jump!
- Muscles, Muscles Everywhere
- Fascinating Friction!
- Design Devices to Help Astronauts Eat: Lunch in Outer Space!
- Blood Cell Basics
- The Beat Goes On
- Do You Have the Strength?
- 20/20 Vision
- Engineering a Mountain Rescue Litter
- Endocrine Excitement!
- Creating Model Working Lungs: Just Breathe
Unit | Lesson | Activity |
TE Newsletter
Engineering connection, learning objectives, materials list, worksheets and attachments, more curriculum like this, introduction/motivation, vocabulary/definitions, troubleshooting tips, activity extensions, activity scaling, user comments & tips.
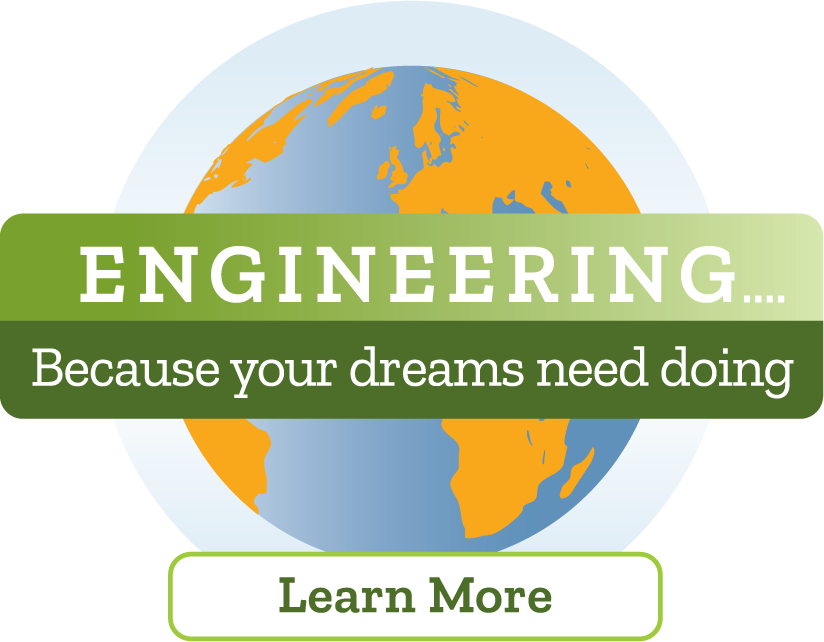
Engineers design many different filters that help people live healthier lives. Engineers design filters to clean water, and they also design special filters called dialysis machines. This equipment, essentially a special filter, cleans blood for people whose kidneys are not working correctly.
After this activity and accompanying worksheet, students should be able to:
- Explain the role of the kidney as a filtering system for blood.
- Describe the by-products of the excretory system.
- Model the filtering function of a kidney on a larger scale.
- Give examples of filters designed by engineers, such as dialysis machines.
Educational Standards Each TeachEngineering lesson or activity is correlated to one or more K-12 science, technology, engineering or math (STEM) educational standards. All 100,000+ K-12 STEM standards covered in TeachEngineering are collected, maintained and packaged by the Achievement Standards Network (ASN) , a project of D2L (www.achievementstandards.org). In the ASN, standards are hierarchically structured: first by source; e.g. , by state; within source by type; e.g. , science or mathematics; within type by subtype, then by grade, etc .
Ngss: next generation science standards - science.
View aligned curriculum
Do you agree with this alignment? Thanks for your feedback!
Common Core State Standards - Math
International technology and engineering educators association - technology, state standards, colorado - math, colorado - science.
Each group needs:
- 6-inch square pieces of each of the following:
- plastic window screening
- hardware cloth (½" mesh)
- hardware cloth (¼" mesh)
- hardware cloth (1/8" mesh)
- poultry netting (1" holes)
- 1-2 sheets of newspaper (to cover desk)
- 2 measuring cups or bowls (about 4 cups each)
- large funnel (large enough to have large pebble flow through the neck)
- ½ cup small pebbles in various sizes from 1/8" to >1"
- ½ cup water
- Filtering System Journal, 4 copies
- Filtering Worksheet, 4 copies
To bind the screens for safety:
Optional materials:
- (optional) round coffee filter
- (optional) 2 tbsp. flour (only needed if using a coffee filter)
To which human body system do the kidneys belong? The excretory ─ or urinary system! This is an important body system because it helps us remove any harmful substances from our body. The kidneys actually work as a filtering system for our blood. They take blood in from the bloodstream, remove waste products (such as salt, minerals and any toxins, or bad stuff) and combine them with water. Then the body gets rid of this water and waste combination, known as urine. The kidney, in a healthy adult, can process as much as 45 liters of water a day, releasing only 1-2 liters in the form of urine.
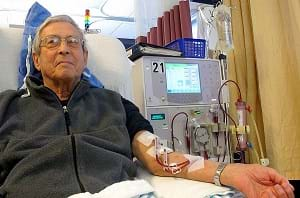
Engineers have designed many amazing filtering systems. They have designed systems to filter bad stuff out of water so that people can have clean water to drink, which helps us stay healthy. They have designed filters to remove harmful particles from the air, so we can have clean air to breathe. They even design filters to prevent unwanted noise from coming through on our phones. Engineers also design special filters for people in the hospital or who have medical problems. Many of the machines that you see in hospitals have been designed by engineers.
Sometimes people have kidneys that do not work well, so engineers have designed a special machine, called a dialysis machine , which filters their blood when their kidneys can not. A dialysis system removes a person's blood through a tube, runs it through filters to remove wastes and extra fluids, and then returns the clean blood to their body. It is a truly amazing system! The dialyzer consists of thousands of small fibers. The blood runs through the fibers, and a cleansing solution runs along the outside of the fibers. The solution acts like a sponge, and it soaks up the extra fluid and waste from the blood. The dirty solution is discarded, and the clean blood gets returned to the body.
Today we are going to test some filter materials and decide which ones work best for removing objects from water. Although these filter models are larger than an actual kidney, they help us investigate how kidneys filter waste from our blood. Also, the model allows us to understand how engineers can design filters to create dialysis machines for people whose kidneys are not working properly.
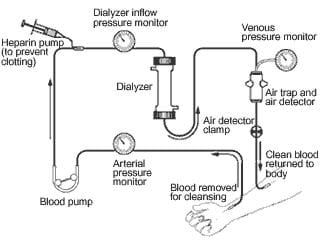
Before the Activity
- Cut screening, poultry netting, and hardware cloth to the proper size.
- Bind the poultry netting and hardware cloth with duct tape to cover sharp edges (see Figure 4).
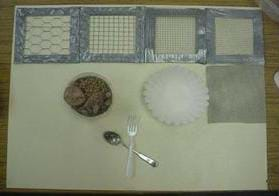
- Assemble all necessary materials.
- Prepare overhead of the Excretory System (attached).
With the Students
- Show students the Excretory System overhead. Ask students to help you identify the location of the kidneys, bladder and ureters. Have students write down these terms under the Vocabulary section of the Filtering System Journal.
- Remind students that the kidneys are a filtering system for the blood. Explain that engineers design dialysis machines for people whose kidneys are not working properly. Have students write a few sentences about how the kidney functions under "I've Learned" on their journal sheets.
- Explain that this activity is a model for how the kidney cleans the blood. Students should be clear that this is just a model and that the kidney does not actually filter solids, except for blood cells. The products filtered through the screens in the activity are meant to represent the waste products in the blood, which are excreted in urine. Urine contains glucose, sodium, potassium, bicarbonate, water, acid, blood cells, protein and urea (which is what makes urine yellow).
- Students should mix the sand, pebbles, flour (optional) and water in the first measuring cup or bowl, as shown in Figure 4.
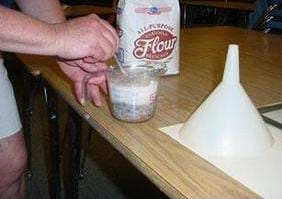
- The students should take turns filtering the water mixture through the funnel, poultry netting, different-sized hardware cloth, window screening and coffee filter (optional), from large-filter holes to small-filter holes (see Figure 6). The screening should be held over the second measuring cup/bowl. Students should then pour the mixture from the full measuring cup/bowl onto the screen over the empty container and then back again, using a different screen each time.
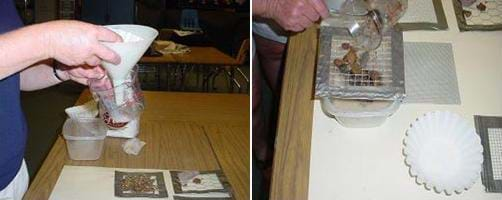
- Students should complete the Observations section of the Filtering System Journal.
- Discuss with the students what they have learned and have them work through the Filtering Worksheet. Ask the students how the filter model they created might be used by engineers in creating dialysis machines. Have students write down any other things they have learned in their Filtering System Journal under the "I've Learned" section, and answer any questions the students may have written in the "Questions I Have" section of their journal.
dialysis: The process of separating substances in solution by diffusion through semipermeable membranes.
dialysis machine: Equipment that filters blood when the kidneys cannot.
hemodialysis: The process that refers specifically to purifying blood by dialysis.
Pre-Activity Assessment
Vocabulary: Have students write down the terms kidneys, excretory system, ureters and bladder under the Vocabulary section of the Filtering System Journal. Have them discuss with a neighbor what these terms mean and write short definitions next to the terms.
Activity Embedded Assessment
Worksheet/ Observations: Explain to students that engineers and scientists record their observations while they are working with a model. Using the Filtering System Journal, ask them to write down anything they noticed during the activity in the "Observations" section of their journal, and any questions they might have in the "Questions I Have" section. Review their answers to gauge their mastery of the subject.
Post-Activity Assessment
Discussion: Lead a discussion out loud, asking students what they have learned about filters and engineering. Have students write down the things they have learned in their Filtering System Journal under the "What I've Learned" section. Make sure to answer any questions the students may have written in the "Questions I Have" section of their journal.
Math Extension: Have students work through the Filtering Worksheet. Remind students that there are numbers that engineers would need to know in order to size a dialysis machine properly.
Safety Issues
Be careful when handling the sharp edges of the mesh screening.
Use a large enough container to contain the poured water.
Have students research engineering systems that use filters (water treatment systems, waste water treatment systems, etc.) Remind students that many engineered systems actually are designed with knowledge that engineers have learned through science and nature.
Invite a medical technician to speak to the class about dialysis machines.
For upper grades, explain more of the details of how a dialysis machine works. Some students may have a friend or relative who is or has been on dialysis. Talk about what qualities are important to consider when designing a dialysis machine (sterility, work as similar to the body's function as possible, comfort level of the patient, ease of use for the patient and nurse/aide, etc.). Check out https://www.niddk.nih.gov/health-information/kidney-disease/kidney-failure/hemodialysis for more information on dialysis. Have students complete the challenge section of the math worksheet.
For lower grades, discuss why we use the filters in order of largest to smallest mesh size, and not the other way around. Talk with students about how in mathematics and in many engineering applications ─ (as well as in life!) ─ the order in which we do things is very important. Doing things in the correct order helps us achieve and understand the outcomes of a model. The math worksheet may not be appropriate for students under 3rd grade.

This lesson helps students explore the functions of the kidney and its place in the urinary system. Students learn how engineers design instruments to help people when kidneys are not functioning properly or when environmental conditions change, such as kidney function in space.

Using ordinary classroom materials, students act as biomedical engineering teams challenged to design prototype models that demonstrate semipermeability to help medical students learn about kidney dialysis. A model consists of two layers of a medium separated by material acting as the membrane. Grou...

U.S. Department of Health and Human Services, National Institutes of Health, National Institute of Diabetes and Digestive and Kidney Diseases, National Institutes of Health, National Kidney and Urologic Diseases Information Clearinghouse (NKUDIC), NIH Publication No. 06–4281, March 2006, "Prevent Diabetes Problems: Keep Your Kidneys Healthy," http://diabetes.niddk.nih.gov/dm/pubs/complications_kidneys/
U.S. Department of Health and Human Services, National Institutes of Health, National Institute of Diabetes and Digestive and Kidney Diseases, National Institutes of Health, National Kidney and Urologic Diseases Information Clearinghouse (NKUDIC), NIH Publication No. 03–4666, September 2003, "Treatment Methods for Kidney Failure: Hemodialysis," http://kidney.niddk.nih.gov/kudiseases/pubs/hemodialysis/
Contributors
Supporting program, acknowledgements.
The contents of this digital library curriculum were developed under grants from the Fund for the Improvement of Postsecondary Education (FIPSE), U.S. Department of Education and National Science Foundation (GK-12 grant no. 0338326). However, these contents do not necessarily represent the policies of the Department of Education or National Science Foundation, and you should not assume endorsement by the federal government.
Last modified: September 11, 2019
Kidneys and Regulation of Water and Inorganic Ions
Kidneys regulate water and inorganic ions
Table of Contents
Renal Functions
Kidneys remove/add substances from/to the plasma.
- Regulate water concentration, inorganic ion composition, and volume of the internal environment by controlling their excretion.
- Excrete metabolic wastes, including urea, uric acid, and creatinine into urine
- Excrete foreign chemicals in urine
- Synthesize glucose from amino acids and other precursors (gluconeogenesis).
- Secrete the hormones, erythropoietin, renin, and 1, 25-dihydroxyvitamin D3.
Structure of Kidneys and Urinary System
The two kidneys lie near the back of the abdominal wall. Urine flows from kidneys through ureters into the bladder, from which it is eliminated through the urethra. The outer part of the kidney is called the renal cortex and the inner part is called renal medulla. Each kidney is made up of subunits called nephrons and each nephron consists of (1) an initial filtering component called renal corpuscle located in the cortex and (2) a tubule located in the medulla that extends out from renal corpuscle. The renal corpuscle forms a filtrate that is free of cells and proteins, and as it flows through the tubule, substances are added to it or removed from it and the final exiting fluid is called urine.
Each renal corpuscle contains a tuft of capillary loops called glomerulus, which protrudes into a fluid-filled space called Bowman’s space. Blood enters the glomerulus by an afferent arteriole and leaves it by an efferent arteriole. Protein free fluid from glomerulus enters Bowman’s space and is drained by the proximal tubule which leads to the descending limb of the loop of Henle, from where the fluid goes to the ascending limb and then to the distal convoluted tubule. The fluid then flows into the collecting duct system, comprised of the connecting tubule, followed by the cortical collecting duct and the medullary collecting duct. Medullary collecting ducts from numerous nephrons merge and drain into the renal pelvis, which is continuous with the ureter. The tubules are also connected to another set of blood vessels called peritubular capillaries. The part of ascending limb passing between the afferent and efferent arterioles has a patch of cells called macula densa and wall of the afferent arteriole at this point has cells called juxtaglomerular (JG) cells, which secrete the hormone renin. These two types of cells comprise the juxtaglomerular apparatus (JGA).
Basic Renal Processes
Filtration of plasma from glomerular capillaries into Bowman’s space is called glomerular filtration and the filtrate is called glomerular filtrate. During its passage through tubules, substances move from tubules to peritubular capillaries, a process called tubular reabsorption and substances move from peritubular capillaries to tubules, a process called tubular secretion.
A E = A F + A S – A RA
A E = Amount excreted A F = Amount filtered A S = Amount secreted A RA = Amount reabsorbed
For a given substance, a particular combination of all these processes applies.
Glomerular filtration
Glomerular filtrate contains all plasma substances in the same concentrations as plasma except proteins and molecules bound to these proteins. It is a bulk flow process.
Net glomerular filtration pressure = P GC – P BS – π GC
P GC is glomerular capillary hydrostatic pressure favoring filtration from capillary to Bowman’s space P BS is Bowman’s space hydrostatic pressure favoring movement from Bowman’s space to capillaries π GC is osmotic pressure resulting from the presence of protein in glomerular capillary plasma and no protein in Bowman’s space
Normally, net filtration pressure is positive.
Glomerular Filtration Rate (GFR) – Volume of liquid filtered from glomeruli into the Bowman’s capsule per unit time. GFR is determined by net filtration pressure, the permeability of the corpuscular membranes and surface area available for filtration. GFR is subject to physiological regulation by neural and hormonal inputs to afferent and efferent arterioles. Constriction of afferent arterioles decreases PGC while constriction of efferent arterioles increases it. Mesangial cells, which are modified smooth muscle cells are involved in this constriction process.
The filtered load is the total amount of any nonprotein substance filtered into Bowman’s space. It is given by multiplying GFR with the plasma concentration of the substance. If the quantity of a substance excreted in urine is less than filtered load, tubular reabsorption has occurred, if it is more then tubular secretion has occurred.
Tubular reabsorption
Waste products are reabsorbed incompletely so that they are mostly excreted in the urine, while useful products are reabsorbed completely so that they are mostly not excreted.
Reabsorption by mediated transport is responsible for reabsorption of many substances, e.g., glucose molecules are coupled to the reabsorption of sodium. The limit to which these mediated transport systems can move materials per unit time is called transport maximum (Tm). This limit is a result of the saturation of binding sites on membrane transport proteins. In people with diabetes mellitus, plasma glucose concentration is so high that the filtered load of glucose exceeds the glucose Tm. and therefore, glucose appears in urine (glucosuria).
Tubular secretion
Tubular secretion is responsible for moving substances, e.g., H + and K + ions, from peritubular capillaries into tubular lumen by diffusion or transcellular mediated transport. Some of the movements are coupled to reabsorption of Na + ions.
Tubule cells can synthesize glucose, ammonia, etc. and add it to blood as needed. It can catabolize peptides etc. and remove them from the body.
Hormones and neurotransmitters regulate channels and transporters. In order to excrete waste products, GFR must be large resulting in large filtered loads of substances. The primary role of the proximal tubule and loop of Henle is to reabsorb large quantities of substances. Such extensive reabsorption ensures that distal segments receive small amounts of substances and that their quantities in urine can be fine-tuned and regulated. Most homeostatic controls are therefore exerted on distal segments.
Renal clearance
Renal clearance is the measure of the volume of plasma from which a substance is completely removed by kidneys per unit time.
Clearance of substance S = (Mass of S excreted per unit time)/Plasma concentration of S
C S = (U S V)/P S
Where: C S = clearance of S U S = urine concentration of S V = urine volume per unit time P S = plasma concentration of S C S of a substance equals GFR if it is filtered but not reabsorbed, secreted or metabolized.
Micturition
Urine flow through ureters to the bladder is propelled by contractions of ureter wall smooth muscle. Urine is stored in the bladder and ejected during urination or micturition. The bladder is a chamber with walls made of smooth muscle called detrusor muscle, contraction of which produces urination. Part of the muscle at the base of the bladder, where urethra begins, functions as a sphincter called the internal urethral sphincter. Below this sphincter is a ring of skeletal muscle called the external urethral sphincter, which surrounds the urethra.
The detrusor muscle receives parasympathetic input, while the internal sphincter receives sympathetic input and the external sphincter receives motor input. While the bladder is filling, there is little parasympathetic input to the detrusor muscle but there are strong sympathetic and motor inputs to the sphincters. While filling occurs, the detrusor muscle is relaxed, and sphincters are closed. As the bladder fills, stretch receptors stimulate the parasympathetic fibers, resulting in contraction of the detrusor muscle. Sympathetic and motor inputs to sphincters are inhibited and sphincters open to produce urination. There is voluntary control over the external sphincter.
Total Body Balance of Sodium and Water Water is gained from:
- Oxidation of organic nutrients.
Water is lost from
- Skin via sweat glands
- Respiratory passageways
- Gastrointestinal tract
- Urinary tract
Water and salt balance is primarily a result of regulation through urinary loss.
Basic renal processes for sodium and water
Sodium and water filter freely from glomerular capillaries to the Bowman’s space. They undergo considerable reabsorption in the proximal tubule but the major hormonal controls on reabsorption are exerted in the collecting ducts. They are not secreted into the tubules.
Primary Active Sodium Reabsorption. Sodium moves out of lumen into the epithelium by diffusion or by ion channels or by cotransport with glucose (which is also being reabsorbed) or countertransport with H + ions (which are being secreted). Na + /K + -ATPase transports sodium out of the epithelium into the interstitial fluid.
Coupling of Water Reabsorption. The removal of sodium lowers the osmolarity of the lumen and raises that of the interstitial fluid. This causes a net diffusion of water from the lumen into the interstitial fluid through the epithelium. Water permeability of the proximal tubule is high but only that of collecting ducts is under the control of vasopressin (ADH). ADH stimulates the insertion of aquaporin channels, increasing water permeability. Low ADH leads to water diuresis or diabetes insipidus. Increased urine flow due to increased solute excretion is called osmotic diuresis.
The Countercurrent Multiplier System
Fluid from proximal tubule has the same osmolarity as plasma since it absorbs sodium and water equally. In the ascending limb, sodium, but not water, is actively reabsorbed from the lumen, making the interstitial fluid of the medulla hyperosmotic. Due to this hyperosmocity, there is passive diffusion of water from the lumen into the interstitial fluid in the descending limb. Fluid in the distal tubule becomes progressively dilute as sodium is transported out and then in the cortical and medullary ducts, water diffuses out of the tubule into the hyperosmotic interstitial fluid and urine is concentrated.
Renal Sodium Regulation
Since sodium is the major extracellular solute, changes in total body sodium result in changes in the volume of extracellular fluid, changing plasma volume and therefore blood pressure, which is detected by the baroreceptors.
Sodium excreted = Sodium filtered – Sodium reabsorbed
Lower total body sodium can decrease GFR by vasoconstriction, resulting in lower pressure in renal arteries. The control of reabsorption is more important for long-term regulation. Aldosterone stimulates sodium reabsorption by cortical collecting ducts (and large intestine, sweat, and salivary glands). The secretion of aldosterone is controlled by angiotensin II, which is produced from angiotensinogen in a reaction, the rate-limiting step of which is controlled by renin from JG cells. These cells act as internal baroreceptors as well as receive sympathetic inputs from external baroreceptors. Angiotensin II is also a vasoconstrictor itself.
Renal Water Regulation
Water excreted = Water filtered – Water reabsorbed Water excretion is regulated mainly at the level of reabsorption by vasopressin.
- Baroreceptor control of vasopressin secretion. The secretion of vasopressin can be triggered by decreased extracellular volume but the baroreceptor reflex plays a relatively lesser role because it has a higher threshold.
- Osmoreceptor control of vasopressin secretion. Changes in total body water with which there is no change in total body sodium are regulated by reflexes that alter water excretion without altering sodium excretion. Receptors that control vasopressin secretion mainly due to water gain or loss are the osmoreceptors in the hypothalamus.
- Thirst. Stimulated by lower extracellular volume, higher plasma osmolarity, angiotensin II and the brain centers for thirst are located in the hypothalamus.
Potassium Regulation
Potassium is filtered in the renal corpuscle and most of it absorbed in the tubules. Any changes in potassium excretion, however, are mainly due to changes in potassium secretion by cortical collecting ducts. This secretion is associated with reabsorption of sodium by Na, K-ATPase. Aldosterone-secreting cells are sensitive to potassium concentration of their extracellular fluid and an increased potassium concentration stimulates aldosterone production, thereby increasing potassium secretion and its excretion from the body.
Calcium Regulation
In addition to the gastrointestinal tract and kidneys, which determine net intake and excretion of calcium, calcium can be redistributed between extracellular fluid and bone.
- Kidneys. Calcium is filtered in the renal corpuscle and most of it is reabsorbed. There is no tubular secretion of calcium. Therefore, Calcium excreted = Calcium filtered – Calcium absorbed Control of calcium excretion is exerted mainly on reabsorption.
- Gastrointestinal tract. Calcium absorption in the GI tract is under hormonal control and is a major means for control of calcium balance.
- Parathyroid hormone. Parathyroid hormones are produced by parathyroid glands in the neck that are controlled directly by extracellular calcium concentration. It increases the movement of calcium from bone tissue into the extracellular fluid, increases renal tubular calcium reabsorption and stimulating the production of 1, 25-dihydroxy-vitamin D3.
- 1, 25-dihydroxyvitamin D3. 1, 25-dihydroxy-vitamin D3 is derived from vitamin D in the liver and kidneys, this hormone increases intestinal absorption of calcium.
- Calcitonin. Parafollicular cells of thyroid gland secrete calcitonin; it decreases plasma calcium concentration by reducing bone resorption.
Hydrogen Ion Regulation
Hydrogen ions can be redistributed in the body by binding it reversibly with a buffer such as bicarbonates, phosphates, proteins and Hb.
- Respiratory Mechanisms. Ventilation is altered by reflex mechanisms in order to compensate for H + ion imbalance.
- Renal Mechanisms. Kidneys compensate for H + ion imbalance by altering plasma HCO3 – ion concentration. A lowering of plasma H + ion concentration results in excretion of large quantities of HCO3 – ions while a rise in H + ion concentration results in the production of HCO 3 – ions and their addition to plasma by tubular cells.
Bicarbonate Handling
HCO 3 – is filtered at the renal corpuscle and undergoes reabsorption in the tubule. It is also secreted in the collecting ducts. Therefore: HCO 3 – excreted = HCO 3 – filtered + HCO3 – secreted – HCO 3 – reabsorbed Inside the cell, CO 2 and H 2 O combine to form H 2 C0 3 , which dissociates to yield H + and HCO 3 -ions. HCO 3 -moves to the interstitial fluid by diffusion while H + ion is secreted into the lumen by an active process involving H-ATPase pumps. The secreted H + ion combines with filtered HCO 3 – in the lumen and generates CO 2 and H 2 O, which diffuse into the cell and the whole process is repeated. If an excess of H + ions is secreted, it combines with nonbicarbonate buffer, usually HPO 4 -2 , in the lumen and is excreted. In such a case, the HCO 3 – generated within the cell and entering the plasma is a net gain of HCO 3 – .
Select the best answer from the parenthesis. Write your answer in the space provided.
Send Your Results (Optional)
More worksheets:
- Urinary System Quiz
- Rat Urogenital Worksheet
You will also like...
Lotic communities & algae.
Lotic communities have conditions that are rather harsh for typical plants. Thus, the diversity of plant species in loti..
Sensory Systems
A sensory system is a part of the nervous system consisting of sensory receptors that receive stimuli from the internal ..
New Zealand’s Unique Fauna
Meet some of New Zealand's unique fauna, including endemic insects, frogs, reptiles, birds, and mammals, and investigate..
Freshwater Communities & Lentic Waters
Lentic or still water communities can vary greatly in appearance -- from a small temporary puddle to a large lake. The s..
The Homo Species
The evolution of the species of the genus "Homo" led to the emergence of modern humans. Find out more about human evolut..

Still Water Community Plants
This tutorial looks at the adaptations of freshwater plants for them to thrive in still water habitats. Familiarize your..
- How fit Works
STEM Activity: Kidney Experiment
Conduct your own experiment and learn more about the kidneys! Follow along and get creative, or use with the kidney science lesson.
Related Content
Preparing your child for their doctor visit: a printable activity kit.
Going to the doctor can be intimidating! With this fun activity packet, kids and caregivers will learn through play, and feel prepared for their next visit!
fit -Check Door Sign
Create a sign to help you remember to stop and think about making healthy choices.
Preparing Your Child For Their Doctor Visit: A Printable Activity Kit (Español)
¡Ir al médico puede ser intimidante! Con este divertido folleto de actividades, los niños y los cuidadores pueden aprender a través del juego y sentirse preparados para su próxima visita.
fit Games Teacher's Guide
This guide includes information about best practices for grouping children for activities and a fitGames learner assessment rubric.
fit Choices
Kids show choices about recharging their bodies, managing their mood, making healthy food choices, and doing more physical activity.
Times and Places
Kids brainstorm times and places they will do a fit-check.
Sciencing_Icons_Science SCIENCE
Sciencing_icons_biology biology, sciencing_icons_cells cells, sciencing_icons_molecular molecular, sciencing_icons_microorganisms microorganisms, sciencing_icons_genetics genetics, sciencing_icons_human body human body, sciencing_icons_ecology ecology, sciencing_icons_chemistry chemistry, sciencing_icons_atomic & molecular structure atomic & molecular structure, sciencing_icons_bonds bonds, sciencing_icons_reactions reactions, sciencing_icons_stoichiometry stoichiometry, sciencing_icons_solutions solutions, sciencing_icons_acids & bases acids & bases, sciencing_icons_thermodynamics thermodynamics, sciencing_icons_organic chemistry organic chemistry, sciencing_icons_physics physics, sciencing_icons_fundamentals-physics fundamentals, sciencing_icons_electronics electronics, sciencing_icons_waves waves, sciencing_icons_energy energy, sciencing_icons_fluid fluid, sciencing_icons_astronomy astronomy, sciencing_icons_geology geology, sciencing_icons_fundamentals-geology fundamentals, sciencing_icons_minerals & rocks minerals & rocks, sciencing_icons_earth scructure earth structure, sciencing_icons_fossils fossils, sciencing_icons_natural disasters natural disasters, sciencing_icons_nature nature, sciencing_icons_ecosystems ecosystems, sciencing_icons_environment environment, sciencing_icons_insects insects, sciencing_icons_plants & mushrooms plants & mushrooms, sciencing_icons_animals animals, sciencing_icons_math math, sciencing_icons_arithmetic arithmetic, sciencing_icons_addition & subtraction addition & subtraction, sciencing_icons_multiplication & division multiplication & division, sciencing_icons_decimals decimals, sciencing_icons_fractions fractions, sciencing_icons_conversions conversions, sciencing_icons_algebra algebra, sciencing_icons_working with units working with units, sciencing_icons_equations & expressions equations & expressions, sciencing_icons_ratios & proportions ratios & proportions, sciencing_icons_inequalities inequalities, sciencing_icons_exponents & logarithms exponents & logarithms, sciencing_icons_factorization factorization, sciencing_icons_functions functions, sciencing_icons_linear equations linear equations, sciencing_icons_graphs graphs, sciencing_icons_quadratics quadratics, sciencing_icons_polynomials polynomials, sciencing_icons_geometry geometry, sciencing_icons_fundamentals-geometry fundamentals, sciencing_icons_cartesian cartesian, sciencing_icons_circles circles, sciencing_icons_solids solids, sciencing_icons_trigonometry trigonometry, sciencing_icons_probability-statistics probability & statistics, sciencing_icons_mean-median-mode mean/median/mode, sciencing_icons_independent-dependent variables independent/dependent variables, sciencing_icons_deviation deviation, sciencing_icons_correlation correlation, sciencing_icons_sampling sampling, sciencing_icons_distributions distributions, sciencing_icons_probability probability, sciencing_icons_calculus calculus, sciencing_icons_differentiation-integration differentiation/integration, sciencing_icons_application application, sciencing_icons_projects projects, sciencing_icons_news news.
- Share Tweet Email Print
- Home ⋅
- Science Fair Project Ideas for Kids, Middle & High School Students ⋅
Easy Kidney Science Projects
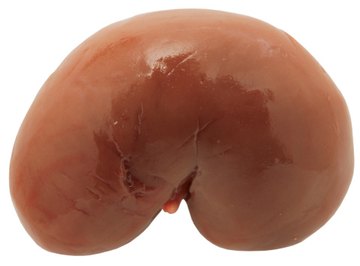
How to Experiment with Coffee Filters to Explain How a Kidney Works
The kidneys are the bean shaped organs that are about the size of a fist located behind the abdomen. They remove extra water and waste from the blood and produce urine. Science projects about the kidneys can be easily constructed to show functions of the kidneys and problems that can occur.
Kidney Model
Science projects about the kidneys should demonstrate their parts and function in the body. Create a clay model about the size of a human fist and bean shaped. On the upper part of each kidney is the adrenal gland which brings blood to be filtered by the kidneys. The other part of the kidneys has tubes called ureters, which run to the bladder and carry the waste produced from filtering the blood. Label each part and research its function to include this information in your project. Build models of both a healthy kidney and one that doesn’t function properly due to disease or age, for comparison.
Kidney Stones
A kidney stone develops from crystals that separate in the urinary tract. Determine if caffeine plays a role in forming or preventing kidney stones. Using calcite stones to represent kidney stones break several stones to similar size and place into separate baby food jars. Add a liquid that contains caffeine in different concentrations such as cola, melted chocolate, tea, coffee to each jar and label the jars. Place a light over each jar to warm them to the temperature of your body (37 degrees Celsius). Observe for two months. During this time, add a small amount of hydrochloric acid to each jar to simulate the body’s stomach acids. Did the caffeine solutions cause the calcite stones to shrink or have no effect?
Urine Production
The kidneys remove waste from the blood and send it to the bladder. Conduct an experiment on how fast the kidneys process different fluids. Drink a liter of water quickly. Wait 20 minutes and then collect your urine in a beaker. Record the volume and color. Use a pH testing strip to record the pH as well. Repeat four times so that you have five samples collected 20 minutes apart. Do the same for two more liquids such as an energy drink and coffee or cola. Determine which fluid produced more urine, which had a higher pH and examine why. For example, energy drinks cause a high output of urine because most energy drinks contain saline. This project can be done with other types of drinks to determine how fast the kidneys process different ingredients.
Filtration System
The kidneys work together as the body’s filtration system. An easy science project for any age is to demonstrate how this works. Mix sand, water, and food coloring in a jar. Half fill another jar with water. Place cheese cloth over the top and slowly pour the sand mixture over it. The filter will allow the colored water through, leaving the sand behind. The water inside the jar changes color because of the food coloring. Dump the water and repeat the experiment adding more water to the sand mixture before pouring on the cheese cloth. The color of the sand and the filtered water will fade as new water is added. This demonstrates how the kidneys clean toxins from the blood.
Related Articles
How to experiment with coffee filters to explain how..., lymphatic system science activities, top ten facts about the human bladder, which part of the body makes blood, what happens to your cells when you are dehydrated, how do energy drinks affect plants, your body on: halloween candy, excretory system science project ideas, body parts & functions, how does the excretory system respond to physical activity, science fair project on the effect of soda on the body, how does the muscular system work with the circulatory..., the effect of salt & sugar on dehydrated cells, science project for the effects that beverages have..., science projects on soda & teeth, human heart science projects, teeth science projects, how to compare the anatomy of a beef heart & a human..., science project on why bones get rubbery in vinegar.
- Argonne National Laboratory: Excretory System Activity
About the Author
Renee Miller began writing professionally in 2008, contributing to websites and the "Community Press" newspaper. She is co-founder of On Fiction Writing, a website for writers. Miller holds a diploma in social services from Clarke College in Belleville, Ontario.
Photo Credits
Stockbyte/Stockbyte/Getty Images
Find Your Next Great Science Fair Project! GO
Thank you for visiting nature.com. You are using a browser version with limited support for CSS. To obtain the best experience, we recommend you use a more up to date browser (or turn off compatibility mode in Internet Explorer). In the meantime, to ensure continued support, we are displaying the site without styles and JavaScript.
- View all journals
- Explore content
- About the journal
- Publish with us
- Sign up for alerts
- Review Article
- Open access
- Published: 01 April 2023
The kidney, volume homeostasis and osmoregulation in space: current perspective and knowledge gaps
- Rik H. G. Olde Engberink ORCID: orcid.org/0000-0002-3177-2279 1 , 2 ,
- Paula J. van Oosten 1 , 2 ,
- Tobias Weber 3 , 4 ,
- Kevin Tabury ORCID: orcid.org/0000-0002-8004-3718 5 ,
- Sarah Baatout ORCID: orcid.org/0000-0001-8110-751X 5 ,
- Keith Siew 6 ,
- Stephen B. Walsh 6 ,
- Giovanna Valenti 7 ,
- Alexander Chouker ORCID: orcid.org/0000-0002-0133-4104 8 ,
- Pierre Boutouyrie 9 , 10 ,
- Martina Heer 11 , 12 ,
- Jens Jordan ORCID: orcid.org/0000-0003-4518-0706 13 &
- Nandu Goswami ORCID: orcid.org/0000-0002-6704-0723 14 , 15
npj Microgravity volume 9 , Article number: 29 ( 2023 ) Cite this article
4291 Accesses
7 Citations
5 Altmetric
Metrics details
- Translational research
An Author Correction to this article was published on 01 August 2023
This article has been updated
Although we have sent humans into space for more than 50 years crucial questions regarding kidney physiology, volume regulation and osmoregulation remain unanswered. The complex interactions between the renin-angiotensin-aldosterone system, the sympathetic nervous system, osmoregulatory responses, glomerular function, tubular function, and environmental factors such as sodium and water intake, motion sickness and ambient temperature make it difficult to establish the exact effect of microgravity and the subsequent fluid shifts and muscle mass loss on these parameters. Unfortunately, not all responses to actual microgravity can be reproduced with head-down tilt bed rest studies, which complicates research on Earth. Better understanding of the effects of microgravity on kidney function, volume regulation and osmoregulation are needed with the advent of long-term deep space missions and planetary surface explorations during which orthostatic intolerance complaints or kidney stone formation can be life-threatening for astronauts. Galactic cosmic radiation may be a new threat to kidney function. In this review, we summarise and highlight the current understandings of the effects of microgravity on kidney function, volume regulation and osmoregulation and discuss knowledge gaps that future studies should address.
Similar content being viewed by others

Cosmic kidney disease: an integrated pan-omic, physiological and morphological study into spaceflight-induced renal dysfunction
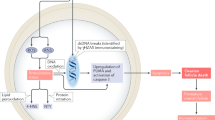
Reproductive hazards of space travel in women and men
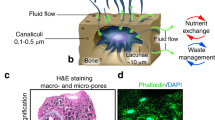
Changes in interstitial fluid flow, mass transport and the bone cell response in microgravity and normogravity
Introduction.
The effect of microgravity on kidney function and volume homeostasis has been of interest for researchers since 1966 1 . Fluid redistribution from the legs to the abdomen, thorax and the head was one of the first observations that was considered to impact kidney physiology 2 . Many studies during space flight and simulated microgravity on Earth have tried to establish the effects of microgravity on kidney function and volume homeostasis. However, essential questions remain unanswered.
Investigation of kidney physiology in space is complex because of the simultaneous changes in osmoregulation, volume regulation and glomerular and tubular function in response to microgravity. To understand the exact impact of microgravity, all responses should be thoroughly tested. This goal is difficult to achieve due to logistic issues and the fact that most measurements are influenced by fluid and nutrient intake, countermeasures, and space flight duration.
Better understanding of kidney physiology and volume homeostasis in space is of special interest because orthostatic intolerance commonly occurs after space flight, which is still unresolved despite the use of extensive countermeasures 3 . More targeted countermeasures that are directed to intervene in kidney physiology and volume homeostasis could conceivably ameliorate orthostatic intolerance. The problem of orthostatic intolerance will become even more important during future exploration missions when astronauts are exposed to longer periods of microgravity and then get re-exposed to a hypogravity environment upon landing on a planetary surface without the usual medical care that is available after return to Earth 4 . In these situations, an orthostatic intolerance-induced fall could be life- and mission threatening. In addition, kidney stone formation could jeopardize future long-term deep space missions where evacuations times will be significantly longer than currently from the International Space Station. In this review, we discuss the key findings of the last decades of relevant research and highlight the most important research gaps concerning volume regulation, osmoregulation and kidney function that should be addressed to prepare astronauts and space medicine experts for future deep space exploration missions (Table 1 ).
Volume regulation
Body weight and compartments.
The central redistribution of fluids was thought to be the main factor impacting body fluid regulation in space. However, some space flight observations cannot be explained by this mechanism. For many years, the immediate and persistent loss of body weight was attributed to fluid loss secondary to increased diuresis as a result of the Henry-Gauer reflex 5 . However, further research demonstrated that diuresis early inflight did not take place, total body water content did not change, and that the reduction of extracellular and plasma volume is compensated by an increase in intracellular volume during short-term space flight 6 . In line with this observation, Leach et al. did not find any increase in sodium or water excretion that could induce a reduction in total body water. Similar responses were observed in head-down tilt bed rest studies 7 , 8 , 9 .
Sodium homeostasis and tissue sodium storage
Recently, there has been a paradigm shift in sodium and water homeostasis, which may shed new light on sodium balance during space flight. Long-term balance studies and metabolic ward studies demonstrated that sodium homeostasis is more complicated than the established intra- and extracellular compartment model 10 , 11 , 12 . These studies showed that 24-hour sodium excretion can differ up to 80 mmol from 24-hour sodium intake during fixed sodium intake, thereby inducing large fluctuations in total body sodium content up to thousands of mmols over weeks (Fig. 1 ). Surprisingly, these substantial fluctuations in total body sodium content, which were related to infradian cortisol and aldosterone rhythms, did not result in concomitant changes in body water, body weight, or blood pressure, and were associated with low-grade metabolic acidosis 10 , 12 , 13 . These data challenge previous assumptions on sodium homeostasis of the past 70 years.
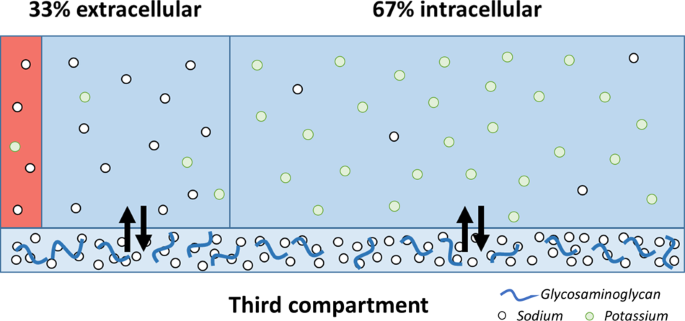
High sodium concentrations (~140 mmol/L) and low potassium concentrations (~4 mmol/L) are found in the extracellular compartment, which consists of an interstitial (blue) and intravascular (red) space. In the intracellular compartment, which compromises 67% of total body water, sodium concentration is low (~10 mmol/L) and potassium concentrations are high (140–150 mmol/L). The classical view on sodium homeostasis suggests that all compartment are equilibrated and that changes in extracellular sodium concentration induce a fluid shift from the intracellular to extracellular compartment. However, supraphysiological high sodium concentrations have been found in the skin and muscle, constituting a third compartment, which is not in osmotic equilibration with the other fluid compartments 98 .
Experimental studies suggest that sodium excess can be neutralized by negatively-charged glycosaminoglycans in the skin, skeletal muscles, and glycocalyx 14 . After binding to glycosaminoglycans, sodium is osmotically inactivated such that sodium retention is not accompanied by water retention. As a result, local sodium concentrations can increase significantly. This process has been described as nonosmotic sodium storage (Fig. 1 ). Others demonstrated that excess sodium may be shifted to the intracellular compartment, in particular to skeletal muscle 15 . In fact, high skin and muscle sodium content has been observed in patients with arterial hypertension, hyperaldosteronism and heart failure, and after high sodium intake 16 , 17 .
The exact cause and function of skin sodium accumulation in humans is not yet understood but experimental evidence demonstrated involvement of the lymphatic system and immune cells, in particular macrophages, in this process 18 . More recently, increased skin sodium content was hypothesized to be an evolutionary measure to limit skin water loss and conserve water in situations of water loss or shortage, which could come at the expense of an increase in blood pressure and muscle catabolism 19 .
Tissue sodium storage during space flight
The discovery of a third compartment for sodium accumulation resulted in new insights for common problems such as hypertension and hyponatremia, but it may also affect hemodynamics and osmoregulation in space (Fig. 2 ) 20 , 21 , 22 . The space flight observation that plasma volume and blood pressure decrease while heart rate increases could be explained in part by volume depletion 7 , 23 . However, a high sodium diet during head-down tilt bed rest did not reverse the decrease in plasma volume, but even resulted in a greater loss of plasma volume, and data from space flight experiments demonstrated significant sodium retention 5 , 8 , 9 , 24 , 25 .
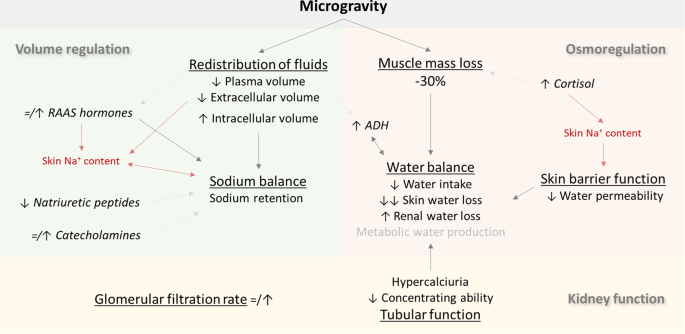
Light grey lines and text indicate findings that are not consistent in literature or have not been investigated thoroughly. Lines in red depict the hypothesized effect of sodium storage in the skin and muscle compartment. ADH antidiuretics hormone, RAAS renin-angiotensin-aldosterone system.
These findings challenge the traditional concept that ingested sodium is either added to the extracellular space, thereby restoring plasma and interstitial volume, or excreted, thereby stabilizing sodium balance. Some of the ingested sodium seems to disappear without inducing the expected fluid retention 24 . These data suggest that the ‘missing’ sodium is stored in the third compartment, which is not in equilibrium with plasma volume and prevents sodium from being excreted by the kidneys, or could be moved to the intracellular compartment.
So far, tissue sodium storage has not been investigated during space travel or bed rest studies. Also, it is unknown how much sodium can potentially accumulate in this third compartment during bed rest. A previous study reported an increasing total body sodium content from day 2 to the study end at day 18 resulting in a 741-mmol positive sodium balance 24 . The amount of sodium that can accumulate in the third compartment is particularly relevant for future deep space exploration missions with long exposure times to microgravity and subsequent planetary surface exploration activities.
During space flight, the third compartment for sodium accumulation (i.e. skin interstitium and muscle) is subject to change. Most studies investigating extracellular volume have reported an initial and persistent reduction without restoration of extracellular volume to pre-existent values, but data on the skin compartment, which represents an important part of the extracellular volume, are scarce 26 . The most comprehensive study on skin function during space flights suggests that skin thickness is not affected by microgravity but other studies have reported opposite results 27 . Contrary to the above, the muscle compartment has been extensively investigated: muscle volume has been shown to decrease, with up to 30% loss of muscle volume in certain muscle groups of the lower, weight-bearing limbs 28 . The reduction of muscle volume could potentially decrease the capacity for muscle sodium accumulation. One may hypothesize that this may contribute to orthostatic intolerance after space flight by reducing the ability to release sodium from the tissues when gravity is restored. This hypothesis is in line with a recent study showing a release of sodium from the tissue stores within 30 min after a hypotonic stimulus 20 . It is, however, unknown whether tissue sodium may be able to play a role in acute hypovolemia. Interestingly, even after return to earth, the body keeps on retaining sodium during the first days (Fig. 3 ) 6 .
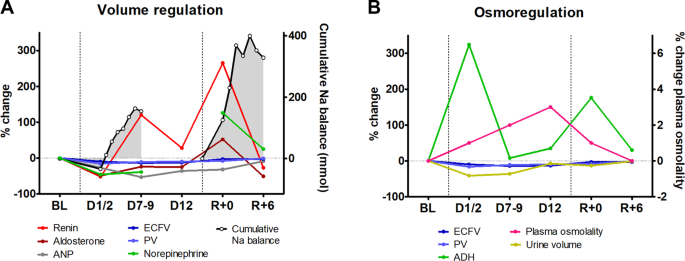
Data on volume regulation ( A ) and osmoregulation ( B ) responses, which are derived from the 9-day and 14-day Spacelab Life Sciences 1 and 2 (SLS-1 and SLS-2) missions 6 . The cumulative sodium balance data is reset at the end of space flight (before R + 0). ADH antidiuretic hormone, ANP atrial natriuretic peptides, BL pre-flight baseline, D day(s) into space flight, R day after return to Earth, ECFV extracellular fluid volume, Na sodium, PV plasma volume.
Muscle sodium storage capacity may be restored by countermeasures that are directed to prevent muscle atrophy. This hypothesis is supported by recent studies demonstrating that the combination of exercise training during space travel and saline loading right after space travel is able to mitigate orthostatic related symptoms 29 , 30 . The exact contribution of both interventions to the mitigation of orthostatic hypotension and potential restoration of the nonosmotic sodium storage capacity is unknown.
Considering the recently proposed role of cortisol in sodium homeostasis, one could hypothesize that the hypothalamic-pituitary-adrenal axis could contribute to volume regulation during microgravity. Throughout the relatively short Skylab flights, cortisol levels were increased but long-duration space flights showed that cortisol levels return to baseline values after 160 days 6 , 31 , 32 . High cortisol levels are generally known to impact protein and muscle catabolism but the recently discovered role in infradian sodium excretion rhythms is less known 10 . Although cortisol can bind to the mineralocorticoid receptors, thus promoting sodium retention, long-term balance studies associated high cortisol levels with a negative sodium balance 10 , 33 . Future studies are needed to investigate the interaction between cortisol, (muscle) catabolism and long-term volume and osmoregulation during space flight.
Renin, aldosterone and natriuretic peptides
The renin-angiotensin-aldosterone system has been studied many times during space flight with different results. These conflicting data can, at least in part, be explained by differences in assays, body posture and space flight duration. Overall, renin and aldosterone levels sharply decrease on the first day of space flight and seem to return to or above pre-flight values thereafter 6 , 25 , 34 . Like on Earth, a high sodium diet or sodium infusion are able to decrease aldosterone levels indicating that sodium balance remains linked to the renin-angiotensin-aldosterone system (RAAS) 25 , 35 . Nevertheless, RAAS hormones are not always in line with the net sodium balance, in particular as observed during early space flight when a positive sodium balance was observed and aldosterone levels were low to normal 6 .
Natriuretic peptides also fall right after space flight but, in contrast to RAAS hormones, remain significantly suppressed after four weeks in space 6 , 25 . Mid-regional pro-atrial natriuretic peptide responded similarly to an increase in sodium intake from 2 to 5.5 grams/day in space and on Earth, however, measurements were reset to lower levels in space 25 . This finding may be partly explained by the reduction in thoracic blood volume during space flight 25 . So far it is unknown whether the reduction in natriuretic peptides is an appropriate physiological response to reduced cardiac preload or whether the response is maladaptive such that sodium balance gets out of tune.
Sympathetic nervous system
Data on sympathetic nervous activity during space flight are contrasting and may be related to mission duration as well as inter-individual variability in the susceptibility to the space environment 36 . Several studies demonstrated that plasma and urine catecholamine levels do not change during long-term space flight 37 , 38 . On the other hand, measurements of whole-body noradrenaline spill-over and peroneal nerve muscle sympathetic activity in six male astronauts during a shorter term space shuttle mission indicated increased sympathetic activity 39 . Possibly, sympathetic measurements during shorter term missions may be confounded by the psychological and physiological stresses imposed by adaptation to the space environment combined with an intense work schedule. Likewise, data after return to Earth show variable sympathetic responses. Although two studies observed a sharp increase in sympathetic activity, a third study could not reproduce this finding despite a substantial increase in cardiac output and decrease in blood pressure 37 , 38 , 40 . However, the link between orthostatic intolerance and sympathetic nerve activity is supported by studies that relate orthostatic complaints to an attenuated increase in plasma norepinephrine and increased norepinephrine metabolites after space flight, and increased plasma norepinephrine after bed rest 23 , 41 , 42 . Interestingly, plasma norepinephrine levels were not affected by acute saline infusion suggesting that a change in volume status may not be the sole mechanism affecting sympathetic nervous activity during spaceflight 35 . Given the importance of the sympathetic nervous system in maintaining orthostatic tolerance and the intense cross-talk between sympathetic nervous system and the RAAS system, which is reviewed in the following section, there is a need for additional studies on sympathetic responses during long duration missions.
Interactions between the sympathetic system and RAAS hormones
Sympathetic activation is one of the driving forces of the renin angiotensin system, either renal, tissular or brain 43 . Both sympathetic activation and RAAS activation may lead to sodium retention, and contribute to the variability in blood pressure responses during space flight 44 . However, only two studies with ten subjects on three different space flights investigated the interaction between neurohumoral systems and sodium and water balance (Fig. 3 ) 6 . The overstimulation of the sympathetic system and RAAS during spaceflight contrasts with the lower levels of cardiac natriuretic peptides 45 . On Earth, cardiac natriuretic peptides and the renin-angiotensin-aldosterone axis are usually regulated in a reciprocal fashion. Whether this resetting of natriuretic peptides in space reflects appropriate responses to reduced central blood volume, true natriuretic peptide deficiency or dysregulation of the RAAS or sympathetic system deserves further studies. These questions are worth investigating since cardiovascular drugs targeting these systems have transformed the prognosis of hypertension and heart failure by treating excessive counter regulations rather than the original cause of disease. In many instances, adaptation to spaceflight might resemble this situation, and cardiovascular drugs targeting neurohormonal response represent interesting candidate drugs for prevention of spaceflight-induced health issues.
Osmoregulation
Water balance.
Microgravity influences water balance but the benefits or mechanisms behind these changes are poorly understood. The available data on water balance should be interpreted in the light that all more recent spaceflights required a minimal oral water intake after the observation that fluid intake decreased significantly when astronauts were not reminded of drinking during the first space missions 34 , 46 , 47 . Taking this and other limitations of space flight studies into account, Drummer et al. concluded that the net water balance during microgravity exposure is likely to be neutral 5 . Nevertheless, water balance is subject to substantial changes in thirst sensation as well as renal and skin water losses.
The reduction in fluid intake upon space travel may result from decreased thirst sensation 48 . This observation cannot be explained by hypo-osmolality, increased extracellular volume or high levels of natriuretic peptides, three triggers that are known to lower thirst sensation on Earth 49 , 50 . Possibly, lower angiotensin-II levels, which may be anticipated from the reduced renin levels during early space flight 6 , may contribute to reduced thirst sensation, although angiotensin-II has been mainly demonstrated to increase thirst upon extracellular volume depletion or infusion, and data on an acute decrease in angiotensin-II levels are not available to this date 50 . Also, thirst sensation could be less due to motion sickness but this could only explain the reduced thirst sensation during the first days upon arrival in microgravity as space motion sickness typically resolves itself after a couple of days 51 .
An alternative explanation of diminished fluid intake may be the production of endogenous metabolic water. Recently, data derived from a terrestrial space station simulating a Mars journey could demonstrate that body water regulation and energy homeostasis are intimately linked by a fundamental physiological adaptation principle designed to prevent dehydration 52 , 53 . These studies related muscle catabolism, which is a major problem during space travel, to metabolic water production. To this date, this aestivation-like mechanism, which has been shown to be activated during renal or skin water loss, has not been explored in microgravity 19 , 54 .
Another determinant of water balance is skin water loss. In contrast to what would be expected in hypobaric conditions, transepidermal water loss is reduced during space flight by approximately 10–20% 27 , 47 . This is accompanied by an improved skin hydration status 27 . An intervention study that temporarily increased skin temperature by 1° Celsius found that, after bed rest, there was an increased threshold for cutaneous vasodilation and sweating 55 . These data on skin barrier function during space flight parallel the findings of a recent study in rats with chronic renal failure and secondary muscle catabolism, increased skin sodium content and reduced skin water loss 19 . This experimental study suggested that a reduction in skin water loss may reflect an adaptive response to overcome excessive (renal) fluid losses and is associated with high skin sodium content.
Data from studies with fluid intake interventions may help to understand the physiology of water balance. Sato et al. conducted a 20-day study of head-down tilt bed rest and instructed participants to drink the amount of fluid that was excreted by the kidneys the previous day 56 . This approach resulted in subjects drinking and urinating more and more every day, from approximately 1100 ml/day to 2400 ml/day at the end of the study. These data suggest that there is a second source of water, which may be of metabolic origin due to muscle wasting, and that the reduced fluid intake may be a consequence. Another study investigated an oral water load of 600 mL during short- and long-term space flight and bed rest. Unexpectedly, urinary flow rate did not increase in space whereas a 5-fold increase was observed on Earth after acute supine position, short-term or long-term bed rest 57 . The observations in space suggest dehydration at baseline resulting in water retention but measurements of urine output and osmolality, after an overnight fast, were similar to the bed rest groups and did not indicate dehydration. This study underscores the lack of understanding of the water balance during microgravity and the notion that data from bed rest studies can be significantly different from data during actual space flight 58 .
Antidiuretic hormone
Water balance is mainly regulated by antidiuretic hormone (ADH), which is secreted upon high plasma osmolality or decreased extracellular volume. During long-term space flight, ADH levels are increased 5 , 48 . As plasma osmolality does not change after long-term space flight, the increased levels may be the consequences of decreased plasma and extracellular volume. Grigoriev et al. showed that the antidiuretic effect of ADH may be attenuated as a result of spaceflight, by demonstrating that higher ADH levels were needed to achieve a similar reabsorption rate of osmotically free water 48 . An important limitation, however, is that measurements were performed directly post-flight, after restoration of gravity. Other post-flight measurements show that the urine concentrating ability of the kidney after a 12-hour food and water deprivation test decreases with space flight to a maximum urine osmolality of 782 mOsm/L after 3–6 months of space flight, which could also be explained by a limited capacity to increase the tonicity of the renal medulla.
In the previously discussed water loading study of Norsk et al., which demonstrated an attenuated increase in urinary flow rate after water loading during space flight compared to bed rest, ADH was not measured 57 . However, the limited decrease in urine osmolality after water loading during space flight suggests either increased ADH levels or changes in the responsiveness of renal collecting ducts to ADH 57 . This discrepancy between space flight and bed rest may be explained by the observations that, in contrast to the increased ADH levels during space flight, ADH decreased during bed rest 59 , 60 .
Kidney function
Glomerular function.
Reports on the effects of space flight on glomerular function vary. Leach et al., who measured creatinine and inulin clearance simultaneously during space flight, reported an increase during the first days and normalization to baseline values afterwards 6 . Surprisingly, this increase took place during the initial reduction in extracellular and plasma volume, and reduced fluid intake, although the latter has been demonstrated to increase glomerular filtration rate on Earth 61 . Another remarkable observation is that creatinine clearance, which is known to overestimate glomerular function, was lower than measured inulin clearance. The observed reduction in plasma urea concentration supports the temporary increase in glomerular filtration rate 6 .
The transient increase in (estimated) glomerular filtration rate has not been observed in older studies, which can be explained by the lack of in-flight measurements and the absence of instructions to maintain adequate fluid intake in these studies.
The observed increased glomerular filtration rate by Leach et al. was not associated with a significant increase in effective renal plasma flow, which suggests an increase in filtration fraction 6 . This finding cannot explain the previously discussed observation of sodium retention, which may therefore be of tubular origin. On the other hand, the large standard deviation of effective renal plasma flow should be taken into account. The coefficient of variation of day-8 creatinine clearance and effective renal plasma flow in the study of Leach et al. was 27% and 39%, respectively, whereas the percentage change from baseline was 20% and 17%, respectively 6 . This suggests that the low sample size and the relative inaccuracy of effective renal plasma flow measurements may contribute to the previously suggested difference in both variables. An alternative explanation is that space conditions led to a change in the balance between afferent and efferent arteriolar constriction in renal glomeruli thereby increasing filtration fraction.
During bed rest, in healthy men, plasma creatinine levels gradually decreased to a new steady state after 2–3 weeks reflecting loss of muscle mass 62 . As 24-hour creatinine excretion did not change this indicates an increase in creatinine clearance in the first 2–3 weeks after bed rest. Interestingly, cystatine C levels showed a similar decrease as plasma creatinine. Contrary to space flight observations, plasma urea increased during the first weeks of bed rest and was related to the observed reduction in muscle mass 62 . Interestingly, Arinell et al. found different results in females 63 . After 60 days of bed rest, plasma cystatin C showed a significant increase whereas creatinine levels remained stable. These data underline the importance that future studies should take sex-specific effects into account.
Tubular function
Histopathological assessment of rat kidneys after simulated microgravity demonstrate degeneration and necrosis of tubular epithelial cells 64 . One could expect that such changes would translate into proteinuria, impaired urine acidification, altered urine electrolyte content or a diminished renal concentrating ability.
The most notable change in 24-hour urine composition during space flight is the increase in urine calcium excretion, which is attributed to increased bone mineral loss. Daily urine calcium excretion has been reported to increase by approximately 20–80 mg/day during microgravity and bed rest 65 , 66 , 67 .
Hypercalciuria has been linked to water balance as high urine calcium content has been demonstrated to induce renal water loss by proteolysis of aquaporin-2 and thereby limiting the maximal urinary concentration and calcium saturation 68 , 69 , 70 . The amount of water loss due to hypercalciuria is likely to be clinically relevant. In hypercalciuric children, hypercalciuria was associated with a 250-mL higher 24-hour urine volume and a 147-mOsm/kg lower urine osmolality 70 . Administration of synthetic ADH (DDAVP) in these hypercalciuric children increased urine osmolality, but only to the baseline levels of normocalciuric children. In bed rest studies, a decrease in aquaporin-2 excretion was associated with increased calcium excretion supporting the view that urinary calcium can modulate ADH-dependent urine concentration through down-regulation of aquaporin-2 expression/trafficking 68 . This phenomenon could have a key role in the prevention of urine supersaturation and stone formation due to hypercalciuria and may contribute to the uncoupling of sodium and water balance during space flight.
Pastushkova et al. analysed urine protein composition before and after space flight. Three proteins, which were not found in the urine on Earth, could be detected in the urine after space flight 71 . One of these proteins, aminopeptidase A, is a marker of renal tissue hypoxia and tubular dysfunction. However, aminopeptidase A was only found seven days after space flight and was not observed three days after return to Earth. Given the lack of in-flight measurements, this may therefore represent changes after space flight instead of changes induced by space flight. Future studies investigating the effects of tubular function during microgravity should therefore focus on in-flight measurements.
Kidney stones
Astronauts and cosmonauts have an unusually high rate of kidney stone formation, despite being screened for absence of kidney stone history. Stones are usually seen after space flight 67 . Among 357 astronauts, 22 experienced at least one episode of kidney stones with a total of 36 episodes 72 . Kidney stone formation is of mission critical significance. In the past, one Soviet in-flight renal stone episode nearly caused a mission termination due to intractable symptoms, but was relieved by spontaneous stone passage by the cosmonaut just before an urgent deorbit was initiated 73 . It has been demonstrated that space flight associated changes in urinary biochemistry favour kidney stone formation 74 , 75 . Besides hypercalciuria due to bone mineral loss, hyperphosphaturia, hypocitraturia and a decrease in urine pH have been reported following microgravity 67 . These changes are the consequence of the high dietary acid load whereas phosphate is also from bone origin 76 . In addition, the reduced water intake and diuresis is a major risk factor for kidney stones. Interestingly, microgravity may also have a direct effect on the crystallisation and nucleation of nascent kidney stones, but this is dwarfed by the net biochemical urinary changes observed in space flight 77 . Changes in the microarchitecture of the kidney can lead to abnormal renal calcification and stones (e.g. in medullary sponge kidney), and changes in the nephron architecture can occur with the kinds of electrolyte disturbance that can be caused by space flight 78 , 79 .
A randomized, double-blind, placebo-controlled study that was performed during space flight demonstrated that supplementation of potassium-citrate lowers urinary calcium excretion and increases urine citrate levels and urine pH during space flight thus reducing the propensity to cristallization 65 . For this reason, potassium-citrate is routinely used by crewmembers who are felt to be at elevated risk for stone formation. During bed rest, potassium-magnesium-citrate supplements were demonstrated to attenuate the increase in calcium excretion and increase in urine pH, potassium, magnesium and citrate levels 66 . Besides these supplementations, compliance with the daily recommended fluid intake of >2 litre is crucial to lower the risk for renal stone formation. Other dietary recommendation such as a low protein and sodium diet, which are advised to individuals at risk for renal stones on Earth, are thought to have a similar beneficial effect during space flight but hard data to support these recommendations are lacking. Considering the previously discussed knowledge gaps on sodium and water homeostasis and muscle metabolism, the exact net effect of these general recommendations may turn out differently.
By interfering with bone resorption, the renal excretion of calcium may also be limited. Administration of pamidronate was shown to reduce 24-hour calcium excretion with 55–65% during the first 30 days of bed rest whereas resistive exercise training was not effective 80 . These large effects, however, could not be reproduced during space flight 26 . A more recent study demonstrated that the combination of alendronate and advanced resistive exercise device training could lower daily calcium excretion below pre-flight values and prevent an increase in calcium oxalate and calcium phosphate relative supersaturation, an effect that could not be achieved with exercise only 81 . In the last decades, vitamin D supplementation was initiated during space flight and doses were increased to 1000 IU per day, which was demonstrated to keep vitamin D at adequate levels without any adverse effects 82 . However, the exact relation between vitamin D dose, 24-hour calcium excretion and renal stone risk during space flights remains to be defined.
Galactic cosmic radiation
There is concern regarding the effect of galactic cosmic radiation (GCR) exposure on the longer missions planned as part of the Artemis Program and the Deep Space Transport/Mars Missions. To date this has mostly focused on the risk of carcinogenesis but the kidney is an exquisitely radiation sensitive organ 83 , 84 , 85 . In fact, the kidney is the dose limiting organ in abdominal radiotherapy and total body irradiation 83 . Beside mitochondrial damage due to the oxidative stress generated by gravitational changes, GCR can further enhance mitochondrial damage through the radiation-induced reactive oxygen species 86 . The resulting mitochondrial damage affect the kidneys at multiple levels in both tubular and glomerular integrity, and micro- and macrovascular function 87 , 88 . To this date, data are limited, but there is emerging animal and astronaut data that link exposure to high atomic number and energy (HZE) ions to mitochondrial damage 89 , 90 . We postulate that the most vulnerable component of the kidney architecture is the proximal tubule, because of its high oxidative metabolism (mitochondria rich), its low glycolytic ability and its crucial role in mass transport of solutes and water. Indeed, tubular injury is an early and prominent histological feature in iatrogenic radiation nephropathy 91 . Due to their full dependence on mitochondrial aerobic metabolism, proximal tubular cells are extremely vulnerable to mitochondrial damage. Altogether, our understanding on the molecular and cellular mechanisms linked to how chronic exposure to GCR impacts kidneys remains to be elucidate and warrant urgent investigation to develop mitigation strategies.
Clinical pharmacology and the kidney
The kidney is not only an essential organ for fluid and ions homeostasis, it is also the main excretion pathway for xenobiotics, among them drugs and toxic compounds. Systematic pharmacodynamic and pharmacokinetic studies in space are lacking. Yet, the profound change in renal physiology could conceivably affect renal drug elimination. Moreover, anecdotal evidence suggests that the response to drug therapies may be altered in space. This issue coupled with limited shelf life of many medications and potential degradation through radiation might be particularly problematic during long-term lunar or mars missions where it is not possible to return to Earth on short notice 92 . To date, around 80 different drugs are available on the ISS 93 . Yet, only the pharmacokinetics of acetaminophen, scopolamine, promethazine and antipyrine have been tested in space 94 . Although, these studies have several limitations, an apparent trend toward altered drug disposition during spaceflight compared to measurements on Earth could be seen 92 , 94 . In particular, the expression changes of CYP450 superfamily of enzymes, which metabolizes 70–80% of all prescription drugs, was observed in rodents, but data in humans are lacking 93 , 95 . Drugs acting on the kidney might be useful for prevention of lithiasis and bone loss. For instance, thiazide-like compounds decrease calciuria, lower the incidence of recurrent kidney stones, prevent bone loss and appear to prevent hip fractures, but their use is limited by the lack of knowledge about their pharmacodynamics and kinetics during spaceflight 96 , 97 . Better understanding of renal changes and their influence on blood detoxification is a call for interdisciplinary programs.
Outlook and summary
To be able to safely increase the length of future space missions, we need future studies to investigate basic kidney physiology, osmoregulation and volume regulation, and develop new countermeasures that are directed to preserve kidney function and prevent potential life-threatening orthostatic complaints and kidney stone formation. A substantial part of these data could be gathered in studies that investigate other hypotheses but allow accurate documentation of sodium and water balance, and repeated urine collections and blood draws for relatively simple analyses of RAAS and antidiuretic hormones, natriuretic peptides, sympathetic nervous activity and catabolic hormones.
Change history
01 august 2023.
A Correction to this paper has been published: https://doi.org/10.1038/s41526-023-00307-x
Dietlein, L. F. & Harris, E. F. Experiment M-5, bioassays of body fluids. NTRS - Nasa Technical Reports Server (Document ID: 19670006697). https://ntrs.nasa.gov/citations/19670006697 (1966).
Moore, T. P. & Thornton, W. E. Space shuttle inflight and postflight fluid shifts measured by leg volume changes. Aviat. Space Environ. Med. 58 , A91–A96 (1987).
CAS PubMed Google Scholar
Goswami, N., van Loon, J., Roessler, A., Blaber, A. P. & White, O. Editorial: Gravitational Physiology, Aging and Medicine. Front Physiol. 10 , 1338 (2019).
Article PubMed PubMed Central Google Scholar
Goswami, N. et al. Human physiology adaptation to altered gravity environments. Acta Astronautica 189 , 216–221 (2021).
Article Google Scholar
Drummer, C., Norsk, P. & Heer, M. Water and sodium balance in space. Am. J. Kidney Dis. 38 , 684–690 (2001).
Article CAS PubMed Google Scholar
Leach, C. S. et al. Regulation of body fluid compartments during short-term spaceflight. 1985). 81 , 105–116. https://doi.org/10.1152/jappl.1996.81.1.105 (1996).
Fortney, S. M., Turner, C., Steinmann, L., Driscoll, T. & Alfrey, C. Blood volume responses of men and women to bed rest. J. Clin. Pharm. 34 , 434–439 (1994).
Article CAS Google Scholar
Hinghofer-Szalkay, H. G. et al. Sodium intake does not influence bioimpedance-derived extracellular volume loss in head-down rest. Aviat. Space Environ. Med 75 , 1036–1041 (2004).
PubMed Google Scholar
Williams, W. J. et al. Effect of dietary sodium on fluid/electrolyte regulation during bed rest. Aviat. Space Environ. Med 74 , 37–46 (2003).
Rakova, N. et al. Long-term space flight simulation reveals infradian rhythmicity in human Na(+) balance. Cell Metab. 17 , 125–131 (2013).
Titze, J. et al. Reduced osmotically inactive Na storage capacity and hypertension in the Dahl model. Am. J. Physiol. Ren. Physiol. 283 , F134–F141 (2002).
Heer, M., Baisch, F., Kropp, J., Gerzer, R. & Drummer, C. High dietary sodium chloride consumption may not induce body fluid retention in humans. Am. J. Physiol. Ren. Physiol. 278 , F585–F595 (2000).
Frings-Meuthen, P., Baecker, N. & Heer, M. Low-grade metabolic acidosis may be the cause of sodium chloride-induced exaggerated bone resorption. J. Bone Min. Res 23 , 517–524 (2008).
Olde Engberink, R. H., Rorije, N. M., Homan van der Heide, J. J., van den Born, B. J. & Vogt, L. Role of the vascular wall in sodium homeostasis and salt sensitivity. J. Am. Soc. Nephrol. 26 , 777–783 (2015).
Wiig, H., Luft, F. C. & Titze, J. M. The interstitium conducts extrarenal storage of sodium and represents a third compartment essential for extracellular volume and blood pressure homeostasis. Acta Physiol. 222 . https://doi.org/10.1111/apha.13006 (2018).
Kopp, C. et al. 23Na magnetic resonance imaging-determined tissue sodium in healthy subjects and hypertensive patients. Hypertension 61 , 635–640 (2013).
Titze, J. et al. Glycosaminoglycan polymerization may enable osmotically inactive Na+ storage in the skin. Am. J. Physiol. Heart Circ. Physiol. 287 , H203–H208 (2004).
Machnik, A. et al. Mononuclear phagocyte system depletion blocks interstitial tonicity-responsive enhancer binding protein/vascular endothelial growth factor C expression and induces salt-sensitive hypertension in rats. Hypertension 55 , 755–761 (2010).
Kovarik, J. J. et al. Adaptive physiological water conservation explains hypertension and muscle catabolism in experimental chronic renal failure. Acta Physiol. 232 , e13629 (2021).
Wouda, R. D., Dekker, S. E. I., Reijm, J., Olde Engberink, R. H. G. & Vogt, L. Effects of Water Loading on Observed and Predicted Plasma Sodium, and Fluid and Urine Cation Excretion in Healthy Individuals. Am. J. Kidney Dis. 74 , 320–327 (2019).
Olde Engberink, R. H. G., Selvarajah, V. & Vogt, L. Clinical impact of tissue sodium storage. Pediatr. Nephrol. 35 , 1373–1380 (2020).
Article PubMed Google Scholar
Olde Engberink, R. H., Rorije, N. M., van den Born, B. H. & Vogt, L. Quantification of nonosmotic sodium storage capacity following acute hypertonic saline infusion in healthy individuals. Kidney Int. 91 , 738–745 (2017).
Meck, J. V. et al. Mechanisms of postspaceflight orthostatic hypotension: low alpha1-adrenergic receptor responses before flight and central autonomic dysregulation postflight. Am. J. Physiol. Heart circulatory Physiol. 286 , H1486–H1495 (2004).
Drummer, C. et al. Water and sodium balances and their relation to body mass changes in microgravity. Eur. J. Clin. Investig. 30 , 1066–1075 (2000).
Frings-Meuthen, P. et al. Natriuretic Peptide Resetting in Astronauts. Circulation 141 , 1593–1595 (2020).
Smith, S. M., Zwart, S. R., Douglas, G. L. & Heer, M. Human Adaptation to Spaceflight: The Role of Food and Nutrition , NP-2021-03-003-JSC (2nd ed.). Houston, TX: National Aeronautics and Space Administration Lyndon B. Johnson Space Center (2021).
Braun, N. et al. Current Data on Effects of Long-Term Missions on the International Space Station on Skin Physiological Parameters. Ski. Pharm. Physiol. 32 , 43–51 (2019).
LeBlanc, A. D. et al. Regional changes in muscle mass following 17 weeks of bed rest. 1985 ). 73 , 2172–2178. https://doi.org/10.1152/jappl.1992.73.5.2172 (1992).
Fu, Q. et al. Impact of Prolonged Spaceflight on Orthostatic Tolerance During Ambulation and Blood Pressure Profiles in Astronauts. Circulation 140 , 729–738 (2019).
Goswami, N., Blaber, A. P., Hinghofer-Szalkay, H. & Convertino, V. A. Lower Body Negative Pressure: Physiological Effects, Applications, and Implementation. Physiol Rev. 99 , 807–851 (2019).
Leach, C. S. & Rambaut, P. C. Biochemical observations of long duration manned orbital spaceflight. J Am Med Womens Assoc (1972) 30 , 153–172 (1975).
Lee, S. M. C. et al. Arterial structure and function during and after long-duration spaceflight. 1985) . 129 , 108-123. https://doi.org/10.1152/japplphysiol.00550.2019 (2020).
Gomez-Sanchez, E. & Gomez-Sanchez, C. E. The multifaceted mineralocorticoid receptor. Compr. Physiol. 4 , 965–994 (2014).
Berry, C. A., Coons, D. O., Catterson, A. D. & Fred Kelly, G. Man’s Respons to Long-Duration Flight in the Gemini Spacecraft. NASA SP-121 , 27 (1966).
Norsk, P. et al. Renal and endocrine responses in humans to isotonic saline infusion during microgravity. 1985) . 78 , 2253-2259. https://doi.org/10.1152/jappl.1995.78.6.2253 (1995).
Jordan, J., Limper, U. & Tank, J. Cardiovascular autonomic nervous system responses and orthostatic intolerance in astronauts and their relevance in daily medicine. Neurol. Sci. 43 , 3039–3051 (2022).
Kvetnansky, R. et al. Plasma and urine catecholamine levels in cosmonauts during long-term stay on Space Station Salyut-7. Acta Astronaut 17 , 181–186 (1988).
Norsk, P., Asmar, A., Damgaard, M. & Christensen, N. J. Fluid shifts, vasodilatation and ambulatory blood pressure reduction during long duration spaceflight. J. Physiol. 593 , 573–584 (2015).
Article CAS PubMed PubMed Central Google Scholar
Ertl, A. C. et al. Human muscle sympathetic nerve activity and plasma noradrenaline kinetics in space. J. Physiol. 538 , 321–329 (2002).
Fritsch-Yelle, J. M., Charles, J. B., Jones, M. M., Beightol, L. A. & Eckberg, D. L. Spaceflight alters autonomic regulation of arterial pressure in humans. 1985) . 77 , 1776–1783. https://doi.org/10.1152/jappl.1994.77.4.1776 (1994).
Waters, W. W., Ziegler, M. G. & Meck, J. V. Postspaceflight orthostatic hypotension occurs mostly in women and is predicted by low vascular resistance. 1985) . 92 , 586–594. https://doi.org/10.1152/japplphysiol.00544.2001 (2002).
Shoemaker, J. K., Hogeman, C. S. & Sinoway, L. I. Contributions of MSNA and stroke volume to orthostatic intolerance following bed rest. Am. J. Physiol. 277 , R1084–R1090 (1999).
Young, C. N. & Davisson, R. L. Angiotensin-II, the Brain, and Hypertension. Hypertension 66 , 920–926 (2015).
Baevsky, R. M. et al. Autonomic cardiovascular and respiratory control during prolonged spaceflights aboard the International Space Station. 1985) . 103 , 156–161. https://doi.org/10.1152/japplphysiol.00137.2007 (2007).
Christensen, N. J., Drummer, C. & Norsk, P. Renal and sympathoadrenal responses in space. Am. J. Kidney Dis. 38 , 679–683 (2001).
Johnston, R. S., Dietlein, L. F. & Berry, C. A. Biomedical Results of Apollo. (Washington, DC, US Government Printing Office, NASA-SP 368, 25, 1975).
Leach, C. S., Leonard, J. I., Rambaut, P. C. & Johnson, P. C. Evaporative water loss in man in a gravity-free environment. J. Appl Physiol. Respir. Environ. Exerc Physiol. 45 , 430–436 (1978).
Grigoriev, A. I., Morukov, B. V. & Vorobiev, D. V. Water and electrolyte studies during long-term missions onboard the space stations SALYUT and MIR. Clin. Investig. 72 , 169–189 (1994).
McKinley, M. J. & Johnson, A. K. The physiological regulation of thirst and fluid intake. N. Physiol. Sci. 19 , 1–6 (2004).
Google Scholar
Leib, D. E., Zimmerman, C. A. & Knight, Z. A. Thirst. Curr. Biol. 26 , R1260–R1265 (2016).
Heer, M. & Paloski, W. H. Space motion sickness: incidence, etiology, and countermeasures. Auton. Neurosci. 129 , 77–79 (2006).
Rakova, N. et al. Increased salt consumption induces body water conservation and decreases fluid intake. J. Clin. Invest. 127 , 1932–1943 (2017).
Kitada, K. et al. High salt intake reprioritizes osmolyte and energy metabolism for body fluid conservation. J. Clin. Investig. 127 , 1944–1959 (2017).
Wild, J. et al. Aestivation motifs explain hypertension and muscle mass loss in mice with psoriatic skin barrier defect. Acta Physiol. (Oxf.). 232 , e13628 (2021).
Michikami, D. et al. Attenuated thermoregulatory sweating and cutaneous vasodilation after 14-day bed rest in humans. 1985) . 96 , 107–114. https://doi.org/10.1152/japplphysiol.00025.2003 (2004).
Sato, M. et al. Effects of encouraged water drinking on thermoregulatory responses after 20 days of head-down bed rest in humans.
Norsk, P. et al. Unexpected renal responses in space. Lancet 356 , 1577–1578 (2000).
Norsk, P. et al. Revised hypothesis and future perspectives. Am. J. Kidney Dis. 38 , 696–698 (2001).
Bestle, M. H., Norsk, P. & Bie, P. Fluid volume and osmoregulation in humans after a week of head-down bed rest. Am. J. Physiol. Regul. Integr. Comp. Physiol. 281 , R310–R317 (2001).
Tamma, G. et al. Early Biomarkers of Altered Renal Function and Orthostatic Intolerance During 10-day Bedrest. Front Physiol. 13 , 858867 (2022).
Anastasio, P. et al. Level of hydration and renal function in healthy humans. Kidney Int 60 , 748–756 (2001).
Bilancio, G. et al. Effects of bed-rest on urea and creatinine: correlation with changes in fat-free mass. PLoS One 9 , e108805 (2014).
Arinell, K., Christensen, K., Blanc, S., Larsson, A. & Fröbert, O. Effect of prolonged standardized bed rest on cystatin C and other markers of cardiovascular risk. BMC Physiol. 11 , 17 (2011).
Ding, Y. et al. Study of histopathological and molecular changes of rat kidney under simulated weightlessness and resistance training protective effect. PLoS One 6 , e20008 (2011).
Whitson, P. A. et al. Effect of potassium citrate therapy on the risk of renal stone formation during spaceflight. J. Urol. 182 , 2490–2496 (2009).
Zerwekh, J. E., Odvina, C. V., Wuermser, L. A. & Pak, C. Y. Reduction of renal stone risk by potassium-magnesium citrate during 5 weeks of bed rest. J. Urol. 177 , 2179–2184 (2007).
Patel, S. R., Witthaus, M. W., Erturk, E. S., Rabinowitz, R. & Nakada, S. Y. A history of urolithiasis risk in space. Can. J. Urol. 27 , 10233–10237 (2020).
Tamma, G. et al. A decrease in aquaporin 2 excretion is associated with bed rest induced high calciuria. J. Transl. Med 12 , 133 (2014).
Drummer, C. et al. Vasopressin, hypercalciuria and aquaporin-the key elements for impaired renal water handling in astronauts? Nephron 92 , 503–514 (2002).
Procino, G. et al. Calcium-sensing receptor and aquaporin 2 interplay in hypercalciuria-associated renal concentrating defect in humans. An in vivo and in vitro study. PLoS One 7 , e33145 (2012).
Pastushkova, L. et al. Detection of renal tissue and urinary tract proteins in the human urine after space flight. PLoS One 8 , e71652 (2013).
Jones, J. A., Pietrzyk, R. A., Cristea, O. & Whitson, P. A. in Principles of Clinical Medicine for Space Flight (M. R. Barratt, E. S. Baker, & S. L. Pool eds) 545–579 (Springer New York, 2019).
Pietrzyk, R. A., Jones, J. A., Sams, C. F. & Whitson, P. A. Renal stone formation among astronauts. Aviat. Space Environ. Med 78 , A9–A13 (2007).
Whitson, P. A., Pietrzyk, R. A., Morukov, B. V. & Sams, C. F. The risk of renal stone formation during and after long duration space flight. Nephron 89 , 264–270 (2001).
Whitson, P. A., Pietrzyk, R. A., Pak, C. Y. & Cintrón, N. M. Alterations in renal stone risk factors after space flight. J. Urol. 150 , 803–807 (1993).
Zwart, S. R. et al. Dietary acid load and bone turnover during long-duration spaceflight and bed rest. Am. J. Clin. Nutr. 107 , 834–844 (2018).
Kassemi, M. & Thompson, D. Prediction of renal crystalline size distributions in space using a PBE analytic model. 1. Effect of microgravity-induced biochemical alterations. Am. J. Physiol. Ren. Physiol. 311 , F520–F530 (2016).
Holliday, M. A., Winters, R. W., Welt, L. G., Macdowell, M. & Oliver, J. The renal lesions of electrolyte imbalance. II. The combined effect on renal architecture of phosphate loading and potassium depletion. J. Exp. Med 110 , 161–168 (1959).
Jones, J. A., Cherian, S. F., Barr, Y. R. & Stocco, A. Medullary sponge kidney and urinary calculi: aeromedical concerns. Aviat. Space Environ. Med 79 , 707–711 (2008).
Okada, A. et al. Risk of renal stone formation induced by long-term bed rest could be decreased by premedication with bisphosphonate and increased by resistive exercise. Int J. Urol. 15 , 630–635 (2008).
Okada, A. A.-O. et al. Bisphosphonate Use May Reduce the Risk of Urolithiasis in Astronauts on Long-Term Spaceflights. JBMR Plus . 6 , e10550 (2021).
PubMed PubMed Central Google Scholar
Smith, S. M. et al. Benefits for bone from resistance exercise and nutrition in long-duration spaceflight: Evidence from biochemistry and densitometry. J. Bone Min. Res 27 , 1896–1906 (2012).
Dawson, L. A. et al. Radiation-associated kidney injury. Int J. Radiat. Oncol. Biol. Phys. 76 , S108–S115 (2010).
Klaus, R., Niyazi, M. & Lange-Sperandio, B. Radiation-induced kidney toxicity: molecular and cellular pathogenesis. Radiat. Oncol. 16 , 43 (2021).
Stewart, F. A. et al. ICRP publication 118: ICRP statement on tissue reactions and early and late effects of radiation in normal tissues and organs-threshold doses for tissue reactions in a radiation protection context. Ann. ICRP 41 , 1–322 (2012).
Li, M. et al. Health risks of space exploration: targeted and nontargeted oxidative injury by high-charge and high-energy particles. Antioxid. Redox Signal. 20 , 1501–1523 (2014).
Turker, M. S. et al. Simulated space radiation-induced mutants in the mouse kidney display widespread genomic change. PLoS One 12 , e0180412 (2017).
Pavlakou, P., Dounousi, E., Roumeliotis, S., Eleftheriadis, T. & Liakopoulos, V. Oxidative Stress and the Kidney in the Space Environment. Int J. Mol. Sci. 19 , 3176 (2018).
Jain, M. R. et al. In vivo space radiation-induced non-targeted responses: late effects on molecular signaling in mitochondria. Curr. Mol. Pharm. 4 , 106–114 (2011).
Indo, H. P. et al. Changes in mitochondrial homeostasis and redox status in astronauts following long stays in space. Sci. Rep. 6 , 39015 (2016).
Cassady, J. R. Clinical radiation nephropathy. Int J. Radiat. Oncol. Biol. Phys. 31 , 1249–1256 (1995).
Tran, Q. D. et al. Space Medicines for Space Health. ACS Med Chem. Lett. 13 , 1231–1247 (2022).
Schmidt, M. A., Schmidt, C. M. & Goodwin, T. J. in Handbook of Space Pharmaceuticals (Y. V. Pathak, M. A dos Santos, & L. Zea eds) 389–427 (Springer International Publishing, 2022).
Eyal, S. How do the pharmacokinetics of drugs change in astronauts in space? Expert Opin. Drug Metab. Toxicol. 16 , 353–356 (2020).
Baba, T. et al. Analysis of gene and protein expression of cytochrome P450 and stress-associated molecules in rat liver after spaceflight. Pathol. Int 58 , 589–595 (2008).
Cheng, L., Zhang, K. & Zhang, Z. Effectiveness of thiazides on serum and urinary calcium levels and bone mineral density in patients with osteoporosis: a systematic review and meta-analysis. Drug Des. Devel Ther. 12 , 3929–3935 (2018).
Aung, K. & Htay, T. Thiazide diuretics and the risk of hip fracture. Cochrane Database Syst Rev ., CD005185. https://doi.org/10.7326/0003-4819-139-6-200309160-00010 (2011).
Olde Engberink, R. H. G., Selvarajah, V. & Vogt, L. Clinical impact of tissue sodium storage. Pediatr. Nephrol. 30 , 019–04305 (2019).
Download references
Acknowledgements
K.S. is supported by the Wellcome Trust [110282/Z/15/Z]. K.S. and S.W. are supported by the UK Space Agency [ST/X000036/1].
Author information
Authors and affiliations.
Amsterdam UMC location University of Amsterdam, Department of Internal Medicine, Section of Nephrology, Meibergdreef 9, Amsterdam, The Netherlands
Rik H. G. Olde Engberink & Paula J. van Oosten
Amsterdam Cardiovascular Sciences, Microcirculation, Amsterdam, The Netherlands
Space Medicine Team, European Astronaut Centre (EAC), Cologne, Germany
Tobias Weber
KBR GmbH, Cologne, Germany
Radiobiology Unit, Belgian Nuclear Research Centre, SCK CEN, Mol, Belgium
Kevin Tabury & Sarah Baatout
London Tubular Centre, UCL Department of Renal Medicine, University College London, London, UK
Keith Siew & Stephen B. Walsh
Department of Biosciences, Biotechnologies and Biopharmaceutics, University of Bari Aldo Moro, Bari, Italy
Giovanna Valenti
Laboratory of Translational Research Stress and Immunity, Department of Anesthesiology, Hospital of the Ludwig-Maximilians-University (LUM), Munich, Germany
Alexander Chouker
Université Paris Cité, Inserm, PARCC, F-75015, Paris, France
Pierre Boutouyrie
Service de Pharmacologie, DMU CARTE, AP-HP, Hôpital Européen Georges Pompidou, FR-75015, Paris, France
German Aerospace Center (DLR), Institute of Aerospace Medicine, Cologne, Germany
Martina Heer
Institute of Nutritional and Food Sciences, University of Bonn, Bonn, Germany
Institute of Aerospace Medicine, German Aerospace Center (DLR) and University of Cologne, Cologne, Germany
Jens Jordan
Gravitational Physiology and Medicine Research Unit, Division of Physiology, Otto Löwi Research Center of Vascular Biology, Inflammation, and Immunity, Medical University of Graz, Graz, Austria
Nandu Goswami
College of Medicine, Mohammed Bin Rashid University of Medicine and Health Sciences, Dubai, United Arab Emirates
You can also search for this author in PubMed Google Scholar
Contributions
R.O.E. and P.vO. drafted the article. T.W., K.T., S.B., K.S., S.W., G.V., A.C., P.B., M.H., J.J. and N.G. critically revised the manuscript. All authors reviewed and approved the final version of the manuscript.
Corresponding author
Correspondence to Rik H. G. Olde Engberink .
Ethics declarations
Competing interests.
The authors declare no competing interests.
Additional information
Publisher’s note Springer Nature remains neutral with regard to jurisdictional claims in published maps and institutional affiliations.
Rights and permissions
Open Access This article is licensed under a Creative Commons Attribution 4.0 International License, which permits use, sharing, adaptation, distribution and reproduction in any medium or format, as long as you give appropriate credit to the original author(s) and the source, provide a link to the Creative Commons license, and indicate if changes were made. The images or other third party material in this article are included in the article’s Creative Commons license, unless indicated otherwise in a credit line to the material. If material is not included in the article’s Creative Commons license and your intended use is not permitted by statutory regulation or exceeds the permitted use, you will need to obtain permission directly from the copyright holder. To view a copy of this license, visit http://creativecommons.org/licenses/by/4.0/ .
Reprints and permissions
About this article
Cite this article.
Olde Engberink, R.H.G., van Oosten, P.J., Weber, T. et al. The kidney, volume homeostasis and osmoregulation in space: current perspective and knowledge gaps. npj Microgravity 9 , 29 (2023). https://doi.org/10.1038/s41526-023-00268-1
Download citation
Received : 08 May 2022
Accepted : 13 March 2023
Published : 01 April 2023
DOI : https://doi.org/10.1038/s41526-023-00268-1
Share this article
Anyone you share the following link with will be able to read this content:
Sorry, a shareable link is not currently available for this article.
Provided by the Springer Nature SharedIt content-sharing initiative
This article is cited by
A second space age spanning omics, platforms and medicine across orbits.
- Christopher E. Mason
- James Green
- Afshin Beheshti
Nature (2024)
Quick links
- Explore articles by subject
- Guide to authors
- Editorial policies
Sign up for the Nature Briefing: Translational Research newsletter — top stories in biotechnology, drug discovery and pharma.

Get to know your kidneys and how to keep them healthy
Try this stem activity about the science behind healthy choices for your kidneys.

Sanford Fit and Sanford PROMISE (Program for the Midwest Initiative in Science Exploration) have teamed up to bring you an amazing new video lesson about the science behind healthy choices and your kidneys.
Why kidneys?
The kidney is one of the many organs that are crucial for keeping you healthy and you probably have two of them. They not only filter your blood, but they also maintain the proper balance of water and nutrients in your body.
“The kidneys get rid of metabolic waste products and maintain the composition of our blood to provide an ideal internal environment for all our organs,” said Dr. Kameswaran Surendran , associate scientist at Sanford Research.
Kidney research at Sanford
Dr. Surendran leads groundbreaking kidney research studies at Sanford Research in Sioux Falls, South Dakota. His lab team focuses on the different types of kidney cells and the role genetics play in proper kidney function. Currently, his lab is focusing on how genetics influence the kidney’s ability to respond to certain types of diets.
“We hope to be able to identify eating habits that are less stressful for our kidneys to keep our kidneys and our bodies healthy,” he said.
One of the many interesting things to understand about the kidney is how it filters blood. The kidneys remove waste from the blood while retaining contents that are necessary for proper body functioning. Most of the blood contents are initially filtered out and flow through a tube heading towards the bladder.
Before the liquid leaves the kidney, the kidney cells reclaim the pieces that the body still needs and returns them to the bloodstream.
“It would be similar to us deciding to clean our homes by first throwing out most of our belongings along with our trash down a long and winding trash chute, and as everything falls through the chute, our family members stand along the chute to actively grab back all the things we still value before it is too late.”
How to keep kidneys healthy
Now that you know how amazing kidneys are, you can take action to help keep your kidneys healthy by making fit choices.
Being active, choosing healthy foods, and drinking plenty of water are great choices you can make to keep your kidneys and body healthy.
Keeping kidneys hydrated is really important. Thirst, nausea, headaches, and even tiredness could be signs that you aren’t getting enough water.
Get hands-on
To learn more and have some fun in your classroom or at home, explore the free resources below. We have a cool video and fun printables to help you and your students learn more about kidneys.
Learning about kidneys video
This video covers three things:
- A hands-on experiment you can do at home or in your classroom
- Easy-to-understand explanation of how a kidney works
- Interesting facts about the kidney from a Sanford Research scientist
Kidney lessons printables
Use the printables to guide you through the lesson and to decorate your space with healthy messages.
- Coloring sheets : These sheets show the different aspects of kidney function. Sheet 1 includes the excretory system including kidneys, ureters, and bladder. Sheet 2 is a cross section of the kidney. Sheet 3 is a nephron and collecting duct.
- Compare and contrast : This sheet is used to help students learn about similarities and differences. You could compare and contrast between the excretory system and digestive system or between your filter and an actual kidney.
- Kidney experiment : This document will help you understand the hands-on activity shown in the video. This is just a starting guide – feel free to let kids get creative and take the experiment in their own direction.
- Scientific method : This document allows students to be scientists by following the same procedure used by researchers. This can be used to frame the experiment from the video and can be used for any question a learner may want to pursue.
- Vocabulary page : The vocabulary terms included in this document are used in the video. Principal and intercalated cells are specific cells that are studied by Dr. Surendran at Sanford Research.
- Healthy choices posters : Decorate your space and inspire kids to make healthy choices.
Sanford PROMISE aims to inspire the next generation of scientists and raise awareness of the benefits of research. Together, Sanford Fit and Sanford PROMISE are some of the leaders in Sanford Health ’s outreach for youth.
- Researcher earns $1.9M grant to study kidney disease genes
- Sanford recognized for kidney transplant care
- Sanford PROMISE celebrates 10 years of learning by doing
Posted In Children's , Family Medicine , Healthy Living , Parenting , Research
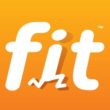
Our content is for informational purposes only and should not be relied upon as medical advice. The content is not designed to replace a physician's medical assessment and medical judgment. As always, please consult first with your physician about health matters. Click here for Terms and Conditions.
Stay up to date with news from Sanford Health
Stay connected.
- About Sanford
- Medical Professionals
- Mobile Apps
- Video Library
- Sanford Health News
- Classes and Events
- Contact Media Relations
- Patient Education
- Sanford Health Plan
- Sanford Health Foundation
- Sanford Imagenetics
- Sanford Research
- Sanford Innovations
- Edith Sanford Breast Center
- Sanford World Clinic
- Sanford Wellness Centers
- Lorraine Cross Award
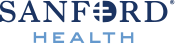
- Privacy of Health Information
- Privacy Statement
- Terms and Conditions
- sanfordhealth.org
- Employee Resources
- Success Center
Warning: The NCBI web site requires JavaScript to function. more...

An official website of the United States government
The .gov means it's official. Federal government websites often end in .gov or .mil. Before sharing sensitive information, make sure you're on a federal government site.
The site is secure. The https:// ensures that you are connecting to the official website and that any information you provide is encrypted and transmitted securely.
- Publications
- Account settings
- Browse Titles
NCBI Bookshelf. A service of the National Library of Medicine, National Institutes of Health.
StatPearls [Internet]. Treasure Island (FL): StatPearls Publishing; 2024 Jan-.

StatPearls [Internet].
Physiology, renal.
Ifeanyichukwu Ogobuiro ; Faiz Tuma .
Affiliations
Last Update: July 24, 2023 .
- Introduction
The renal system consists of the kidney, ureters, and the urethra. The overall function of the system filters approximately 200 liters of fluid a day from renal blood flow which allows for toxins, metabolic waste products, and excess ion to be excreted while keeping essential substances in the blood. The kidney regulates plasma osmolarity by modulating the amount of water, solutes, and electrolytes in the blood. It ensures long term acid-base balance and also produces erythropoietin which stimulates the production of red blood cell. It also produces renin for blood pressure regulation and carries out the conversion of vitamin D to its active form. The renal development, the process of urine production and excretion, and the clinical significance of the renal system will be the focus of this article.
- Development
Three different sets of kidneys develop consecutively from the urogenital ridges, and the last set persists to become the adult kidney. The first renal tubular system is called the pronephros. Pronephros develop during the fourth week of embryonic development but quickly degenerates as mesonephros appears. Mesonephric kidney degenerates as the metanephros develops through its remnant is incorporated into the male reproductive system. The metanephros begins its development around the fifth week of embryonic development as ureteric buds. As the ureteric buds develop, it induces the formation nephrons. [1] The distal ends of the ureteric buds develop into the renal pelvis, calyces, and collecting ducts as the proximal aspect of the ureteric buds develop into ureters. [1] [2] A structure called cloaca develops to form the rectum, anal canal, and urogenital sinus. The urogenital sinus then forms into the urinary bladder and the urethra. By the third month of fetal development, metanephric kidney is able to excrete urine into the amniotic fluid. [3]
Glomerular Filtration
Glomerular filtration is the initial process in urine production. It is a passive process in which hydrostatic pressure pushes fluid and solute through a membrane with no energy requirement. The filtration membrane has three layers: fenestrated endothelium of the glomerular capillaries which allow blood components except the cells to pass through; basement membrane, which is a negatively charged physical barrier that prevents proteins from permeating; and foot processes of podocytes of the glomerular capsule that creates more selective filtration. The outward and inward force from the capillaries determines how much water and solutes crosses the filtration membrane. Hydrostatic pressure from the glomerular capillaries is the major filtration force with a pressure of 55mmHg. The other potential filtration force is the capsular space colloid osmotic pressure, but it is zero because proteins are not usually present within the capsular space. Then the capsular space hydrostatic pressure and the colloid osmotic pressure in glomerular capillaries negate the filtration force from the hydrostatic pressure in the glomerular capillaries, creating a net filtration pressure which plays a big role in the glomerular filtration rate (GFR). [4]
GFR is the volume of fluid filtered in a minute, and it depends on the net filtration pressure, the total available surface area for filtration, and filtration membrane permeability. The normal GFR is between 120 to 125ml/min. It is regulated intrinsically and extrinsically to maintain the GFR. The intrinsic control function by adjusting its own resistance to blood flow via a myogenic mechanism and a tubuloglomerular feedback mechanism. The myogenic mechanism maintains the GFR by constricting the afferent arteriole when the vascular smooth muscle stretches due to high blood pressure. It dilates the vascular smooth muscle when pressure is low within the afferent arteriole allowing more blood to flow through. Then the tubuloglomerular feedback mechanism function to maintain the GFR by sensing the amount of NaCl within the tubule. Macula densa cells sense NaCl around the ascending limb of the nephron loop. [5] When blood pressure is high, the GFR will also be high; this decreases the time needed for sodium reabsorption, and therefore sodium concentration is high in the tubule. The macula densa cell senses it and releases the vasoconstrictor chemicals which constricts the afferent arteriole and reduces blood flow. Then when the pressure is low, Na gets reabsorbed more causing its concentration in the tubule to be low, and macula densa do not release vasoconstricting molecules. [6] [7]
The extrinsic control maintains the GFR and also maintains the systemic blood pressure via the sympathetic nervous system and the renin-angiotensin-aldosterone mechanism. When the volume of fluid in the extracellular decreases excessively, norepinephrine and epinephrine get released and causes vasoconstriction leading to a decrease in blood flow to the kidney and the level of GFR. Also, the renin-angiotensin-aldosterone axis gets activated by three means when the blood pressure drops. The first is the activation of the beta-1 adrenergic receptor, which causes the release of renin from the granular cells of the kidney. The second mechanism is the macula densa cells which senses low NaCl concentration during decreased blood flow to the kidney and trigger the granular cells to release renin. The third mechanism is the stretch receptor around the granular cells senses decreased tension during decreased blood flow to the kidney and also trigger the release of renin, therefore, regulating the glomerular filtration. [6]
T ubular Reabsorption
The four different tubular segments have each unique absorptive properties. The first is the proximal convoluted tubule (PCT). The PCT cells have the most absorptive capability. In the normal circumstance, the PCT reabsorbs all the glucose and amino acids as well as 65% of Na and water. The PCT reabsorb sodium ions by primary active transport via a basolateral Na-K pump. It reabsorbs glucose, amino acids, and vitamins through secondary active transport with Na and an electrochemical gradient drives passive paracellular diffusion. The PCT reabsorbs water by osmosis that is driven by solute reabsorption. It also reabsorbs lipid-soluble solutes via passive diffusion driven by the concentration gradient created by reabsorption of water. Reabsorption of urea occurs in the PCT as well by passive paracellular diffusion driven by a chemical gradient. [8]
From the PCT, the non-reabsorbed filtrates move on to the nephron loop. The nephron loop functionally divides into a descending and an ascending limb. The descending limb functions to reabsorb water via osmosis. This process is possible due to the abundance of aquaporins. Solutes do not get reabsorbed in this region. However, in the ascending limb, Na moves passively down its concentration gradient in the thin segment of the ascending limb, and also sodium, potassium, and chlorides get reabsorbed together through a symporter in the thick segment of the ascending limb. The presence of Na-K ATPase in the basolateral membrane keeps this symporter functional by creating an ionic gradient. There is also the reabsorption of the calcium and magnesium ions in the ascending limb via passive paracellular diffusion driven by the electrochemical gradient. No water reabsorption in the ascending limb. [9]
The next tubular segment for reabsorption is the distal convoluted tubule (DCT). There is a primary active sodium transport at the basolateral membrane and secondary active transport at the apical membrane through Na-Cl symporter and channels. This process is aldosterone regulated at the distal portion. There is also calcium reabsorption via passive uptake controlled by the parathyroid hormone. Aldosterone targets the cells of the distal portion of the DCT causing synthesis and retention of apical Na and K channel as well as the synthesis of Na-K ATPase. [8]
Right after the DCT, there is a collecting tubule where the final stage of reabsorption occurs. The reabsorptions that occur here include primary active sodium transport at basolateral membrane; secondary active transport at apical membrane via Na-Cl symporter and channels with aldosterone regulation; passive calcium uptake via PTH-modulated channels in the apical membrane; and primary and secondary active transport in the basolateral membrane. [10]
Tubular Secretion
Tubular secretion function is to dispose of substances such as drugs and metabolites that bind to plasma protein. Tubular secretion also functions to eliminate undesirable substances that were reabsorbed passively such as urea and uric acids. Elimination of excess potassium via aldosterone hormone regulation at collecting duct and distal DCT are part of tubular secretion function. There is an elimination of hydrogen ion when the blood pH drops below the normal range. Then when the blood pH increases above the normal range, reabsorption of chloride ions occurs as bicarbonic acid gets excreted. The secretion of creatinine, ammonia, and many other organic acids and basics occur. [11]
Storage of Urine
Once the production of urine is complete, it travels through a structure called ureter for urine storage in the bladder. There are two ureters in a human body; one on each side; left and right. They are slender tubes with three-layered walls: the mucosa that contains a transitional epithelial tissue; muscularis that is composed of the internal longitudinal layer and the external circular layer; and adventitia that is a fibrous connective tissue that covers the ureter's external surface. As urine make its way to the ureters, the stretching of the ureter's smooth muscle results in peristaltic contractile waves that help move the urine into the bladder. [12] The oblique insertion of the ureter at the posterior bladder wall prevents backflow of urine. Once the urine is in the bladder, the bladder's unique anatomy allows for efficient storage of urine.
The bladder is essentially a muscular sac with three layers. Its three layers are similar to the ureter except that the muscular layer has muscle fibers organized in inner and outer longitudinal layers and a middle circular layer. The muscular layer is also known as the detrusor muscle. The distensibility of the bladder allows it to hold a maximum capacity of up to 1000ml, though normal functional capacity is 300 to 400mL. [13] The bladder has three openings at the smooth triangular region of the bladder; this is called the trigone. Two of the openings are where the distal portions of the ureters insert, and the other opening is the orifice for the urethra.
The urethra is a thin-walled muscular tube that functions to drain urine out of the bladder. Its mucosa lining consists of mostly pseudostratified columnar epithelium through the proximal portion has transitional epithelial tissue. The thickening of the detrusor muscle at the bladder-urethra junction forms the internal urethral sphincter which has an autonomic nervous system control. The urethra has an additional function for males as it transports semen. In males, the urethra is approximately 22.3 cm long with three regions which include the prostatic urethra, membranous urethra, and the spongy urethra. [14] Females, on the other hand, has a urethra that is approximately 3.8 to 5.1 cm long with an external urethral orifice that lies anterior to the vaginal opening and posterior to the clitoris. [15]
Micturition Process
Micturition process entails contraction of the detrusor muscle and relaxation of the internal and external urethral sphincter. The process is slightly different based on age. Children younger than three years old have the micturition process coordinated by the spinal reflex. It starts with urine accumulation in the bladder that stretches the detrusor muscle causing activation of stretch receptors. The stretch sensation is carried by the visceral afferent to the sacral region of the spinal cord where it synapses with the interneuron that excites the parasympathetic neurons and inhibits the sympathetic neurons. The visceral afferent impulse concurrently decreases the firing of the somatic efferent that normally keeps the external urethral sphincter closed allowing reflexive urine output. However, after the age of 3, there is an override of reflexive urination where there is conscious control of the external urethral sphincter. [16] High bladder volume activates the pontine micturition center which activates the parasympathetic nervous system and inhibits the sympathetic nervous system as well as triggers awareness of a full bladder; consequently leading to relaxation of the internal sphincter and a choice to relax the external urethral sphincter once ready to void. Low bladder volume activates the pontine storage center which activates the sympathetic nervous system and inhibits the parasympathetic nervous system cumulatively allowing the accumulation of urine in the bladder. [17]
- Clinical Significance
The renal system pathologies have a wide range of clinical presentations. Emphysematous urinary tract infections, chronic kidney disease, nephrolithiasis, and urinary incontinence in men and women are topics of discussion below.
Emphysematous UTI is a form of UTI, where infections of the lower or upper urinary tract present with gas formation. Escherichia coli and Klebsiella pneumoniae commonly cause emphysematous UTI although Proteus , Enterococcus , Pseudomonas , Clostridium , and Candida spp can be part of the causative organism. [18] The common risk factors seen in patients with emphysematous urinary tract infections are diabetes and urinary tract obstruction. [19] Emphysematous UTI usually manifests as cystitis, pyelitis, and pyelonephritis with common presentations such as fever, chills, flank or abdominal pain, nausea, and vomiting. Laboratory testing can reveal elevated serum creatinine, pyuria, leukocytosis, and hyperglycemia. Diagnosis can be made with plain film and/or computed tomography, which will show air in the renal parenchyma, bladder, or surrounding tissue. [19] Treatment of emphysematous UTI is usually by systemic antibiotics. [19] [20] Percutaneous drainage might be necessary for pyelonephritis.
Chronic kidney diseases are not uncommon. Approximately 16.8% of the US population has chronic kidney disease (CKD). [21] CKD is the presence of kidney damage with urinary albumin excretion of over 29 mg/day or decreased kidney function with GFR less than 60mL/min/1.73m^2 for three or more months. CKD is classified based on the six GFR stages and the three albuminuria stages. Clinical manifestations include edema and hypertension although some patients can be asymptomatic. Laboratory testings are essential in the diagnosis of CKD. An increase in serum creatinine and urea concentration are very common findings. Hyperphosphatemia, hyperkalemia, hypocalcemia, elevated parathyroid hormone, and metabolic acidosis may also be present in the lab findings. When CKD is suspected, ultrasound, urinalysis with microscopy, and albumin to creatinine ratio are necessary. Ultrasound will help rule out any form of obstruction. [22] Urinalysis with microscopy will help rule out glomerulonephritis in the absence of albuminuria, RBC cast or dysmorphic RBC. Urinalysis can also help rule out interstitial nephritis when sterile pyuria is negative. Once urinalysis is deemed normal, the patient needs evaluation for renovascular disease. If there is no evidence of renovascular disease as a causative factor, a kidney biopsy might be conducted, then evaluation for renal replacement therapy can be done. [23] Management of CKD involves treatment of reversible causes, preventing or slowing the progression of renal disease, treatment of the complications of the renal failure, medication adjustment, and proper education of a patient on the renal disease and on the possibility of needing renal replacement therapy. [24] [25]
Nephrolithiasis is another pathology commonly seen in the renal system. Nephrolithiasis is the presence of crystallized calcium, magnesium, cystine, or uric acid in the renal system. Calcium stones are known to cause eighty percent of nephrolithiasis. Calcium stone has two forms: calcium oxalate which is the most common and the calcium phosphate. [26] [27] Several risk factors lead to nephrolithiasis including high oxalate diet, prior history of nephrolithiasis, family history of nephrolithiasis, recurrent UTI, and enhanced enteric oxalate absorption caused by gastric bypass procedures, bariatric surgery, and short bowel syndrome. [28] [29] Approximately seventy percent of the patients with nephrolithiasis are symptoms free. [30] The most common symptoms associated with nephrolithiasis are waves of waxing and waning unilateral flank pain that lasts 20 to 60 minutes. Hematuria is also a common symptom seen in nephrolithiasis. As the diagnosis of nephrolithiasis is under consideration, other possible pathologies need to be ruled out. For instance, pyelonephritis frequently presents with flank pain, although it also presents with a fever, which is not usually present with nephrolithiasis. Ectopic pregnancy can be mistaken for renal colic. In this case, a renal and pelvic ultrasound can help to clarify. [31] Once symptomatic ureteral stone is clinically suspected, non-contrast renal CT should follow. Pain management should also commence. If urosepsis is present, emergent decompression should be conducted. If urosepsis is absent, the size of the stone should undergo evaluation. Observation, symptomatic treatment, alpha-blocker, and urine straining is appropriate for patients with a stone size of less than 10 mm. Extracorporeal shock wave lithotripsy or ureteroscopy can potentially help patients with stones greater than 10 mm. [32]
Urinary incontinence is also a common renal pathology. It is an involuntary leakage of urine. There are four types of incontinence: urgency incontinence, stress incontinence, mixed incontinence, and incontinence due to incomplete bladder emptying. There are similarities and differences in the causative factors for the different types of urinary incontinence between men and women. In men, 11 to 34 percent of men older than 65 years have urinary incontinence. [33] Advancement in age, prostate disease, history of urinary tract infections, neurologic disease, and diabetes are some of the known risk factors for urinary incontinence. The processes responsible for urgency incontinence is poorly understood, although the understanding is that uninhibited bladder contraction is usually present. Stress urinary incontinence in men is commonly known to be caused by poor urethral sphincter function. Stress urinary incontinence is often a condition in men that underwent prostate surgery or had pelvic trauma and neurologic disorder. [34] Mixed incontinence is when both stress and urge incontinence is present. Overflow incontinence is due to detrusor contractility and/or bladder outlet obstruction. The common presentation for overflow incontinence is nocturnal enuresis due to pelvic floor relaxation that usually occurs at night in combination with a full bladder. Management of urinary incontinence usually starts with lifestyle interventions such as dietary change, weight loss, and pelvic floor muscle exercise. If these lifestyle interventions are not improving the condition, then alpha-blockers can be started. Many other drugs can be used, such as an antimuscarinic or beta-adrenergic agonist, and duloxetine. [35] [36] Then if patients are unresponsive to medical management, invasive treatments such as percutaneous tibial nerve stimulation, sacral nerve stimulation, botulinum toxin injection, transurethral bulking agent injection, perineal slings, or artificial urinary sphincters are all potential options. [37] [38] [39]
Approximately 50 percent of adult women experience urinary incontinence. [40] Common risk factor for urinary incontinence in women includes advanced age, obesity, parity, and mode of child delivery. Stress incontinence is the most common type of urinary incontinence in women between age 45 to 49 years. [41] Mechanisms of stress incontinence include urethral hypermobility which is usually due to insufficient support of pelvic floor musculature and vaginal connective tissue to the urethra and bladder neck as a result of vaginal deliveries. Intrinsic sphincter insufficiency is another factor that leads to stress incontinence in women, and it is mostly as a result of multiple pelvic or incontinence surgeries. [42] Urgency incontinence is commonly seen in older women and is marked by detrusor overactivity as seen in men with urgency incontinence. [43] Overflow incontinence in women results from detrusor inactivity and bladder obstruction. Detrusor contractility decreases with age and a decrease in estrogen has been found to play a role. [44] In women, bladder outlet obstruction is commonly the result of fibroid and advanced pelvic organ prolapse. Management of urinary incontinence in women is very similar to men where lifestyle intervention is started first before pharmacological therapy. Surgical intervention might be necessary.
- Review Questions
- Access free multiple choice questions on this topic.
- Comment on this article.
Disclosure: Ifeanyichukwu Ogobuiro declares no relevant financial relationships with ineligible companies.
Disclosure: Faiz Tuma declares no relevant financial relationships with ineligible companies.
This book is distributed under the terms of the Creative Commons Attribution-NonCommercial-NoDerivatives 4.0 International (CC BY-NC-ND 4.0) ( http://creativecommons.org/licenses/by-nc-nd/4.0/ ), which permits others to distribute the work, provided that the article is not altered or used commercially. You are not required to obtain permission to distribute this article, provided that you credit the author and journal.
- Cite this Page Ogobuiro I, Tuma F. Physiology, Renal. [Updated 2023 Jul 24]. In: StatPearls [Internet]. Treasure Island (FL): StatPearls Publishing; 2024 Jan-.
In this Page
Bulk download.
- Bulk download StatPearls data from FTP
Related information
- PMC PubMed Central citations
- PubMed Links to PubMed
Similar articles in PubMed
- Electrolytes. [StatPearls. 2024] Electrolytes. Shrimanker I, Bhattarai S. StatPearls. 2024 Jan
- [Electrolyte and acid-base balance disorders in advanced chronic kidney disease]. [Nefrologia. 2008] [Electrolyte and acid-base balance disorders in advanced chronic kidney disease]. Alcázar Arroyo R. Nefrologia. 2008; 28 Suppl 3:87-93.
- Renal Function Tests. [StatPearls. 2024] Renal Function Tests. Gounden V, Bhatt H, Jialal I. StatPearls. 2024 Jan
- Review Functional adaptation to reduction in renal mass. [Physiol Rev. 1979] Review Functional adaptation to reduction in renal mass. Hayslett JP. Physiol Rev. 1979 Jan; 59(1):137-64.
- Review Effects of calcium entry blockers on renin-angiotensin-aldosterone system, renal function and hemodynamics, salt and water excretion and body fluid composition. [Am J Cardiol. 1985] Review Effects of calcium entry blockers on renin-angiotensin-aldosterone system, renal function and hemodynamics, salt and water excretion and body fluid composition. Bauer JH, Sunderrajan S, Reams G. Am J Cardiol. 1985 Dec 6; 56(16):62H-67H.
Recent Activity
- Physiology, Renal - StatPearls Physiology, Renal - StatPearls
Your browsing activity is empty.
Activity recording is turned off.
Turn recording back on
Connect with NLM
National Library of Medicine 8600 Rockville Pike Bethesda, MD 20894
Web Policies FOIA HHS Vulnerability Disclosure
Help Accessibility Careers

IMAGES
VIDEO
COMMENTS
Homeostasis - kidneys. The kidneys and water balance is a nice topic to teach. Students have direct experience of the process and can observe changes depending on their own water intake. It is satisfying when students understand processes so directly related to their own physiology. Part of the topic deals with kidney dialysis and transplants.
They not only filter your blood, but they also maintain the proper balance of water and nutrients in your body. ... You could compare and contrast between the excretory system and digestive system or between your filter and an actual kidney. Kidney Experiment: This document will help you understand the hands-on activity shown in the video. This ...
The kidneys filter water to help maintain water balance in the body. The body either reabsorbs the water or processes it through the kidneys to the urinary system to excrete it as urine. Water is ...
STEM Activity: Learning About Kidneys. This video covers a hands-on experiment, an easy-to-understand explanation of how a kidney works, and interesting facts about the kidney from a Sanford Research scientist! Follow along with the kidney science lesson here. BE THE FIRST TO KNOW! Get helpful tips and FREE resources delivered to your inbox ...
Principal cell - A cell of the collecting duct responsible for absorbing water. Intercalated cell - A cell of the collecting duct responsible for absorbing acids and bases. Glomeruli - A cluster of capillaries around the end of a kidney tubule where waste products are ˜ltered. Filtrate - Fluid that is ˜ltered from the blood.Much of the ˜ltrate is reabsorbed into the body.
Description. This video explores how the kidneys filter blood to remove wastes and excess fluid from the body, maintaining a healthy balance of water and salts in the blood. Physician Richard Lifton explains that as fluids (mostly water) from blood move through the kidney, almost all of the water, along with salts and nutrients the body needs ...
Water Balance. On a typical day, the average adult will take in about 2500 mL (almost 3 quarts) of aqueous fluids. Although most of the intake comes through the digestive tract, about 230 mL (8 ounces) per day is generated metabolically, in the last steps of aerobic respiration. Additionally, each day about the same volume (2500 mL) of water ...
Figure 26.2.2 - Antidiuretic Hormone (ADH): ADH is produced in the hypothalamus and released by the posterior pituitary gland. It causes the kidneys to retain water, constricts arterioles in the peripheral circulation, and affects some social behaviors in mammals. ADH has two major effects.
Learning Objectives. After this activity and accompanying worksheet, students should be able to: Explain the role of the kidney as a filtering system for blood. Describe the by-products of the excretory system. Model the filtering function of a kidney on a larger scale. Give examples of filters designed by engineers, such as dialysis machines.
Kidneys remove/add substances from/to the plasma. Regulate water concentration, inorganic ion composition, and volume of the internal environment by controlling their excretion. Excrete metabolic wastes, including urea, uric acid, and creatinine into urine. Excrete foreign chemicals in urine. Synthesize glucose from amino acids and other ...
The kidney is the most important organ in the regulation of water balance in the body. Under normal circumstances, the sensitive hypothalamic osmoreceptors detect any change in extracellular tonicity and respond by altering secretion of ADH from the posterior pituitary. The volume receptors are much less sensitive and really function as a ...
In the early 1990s, Agre and colleagues identified the first molecular water channel, aquaporin-1, which was found to be ubiquitously expressed. 22 In the kidney, this water channel is expressed ...
Mechanism of vasopressin secretion and action. Arginine-vasopressin (AVP) also called antidiuretic hormone (ADH) is of seminal importance in controlling water balance through regulation of water reabsorption along the collecting system and thereby urinary output. AVP is a small peptide composed of 9 amino acids.
STEM Activity: Kidney Experiment. Conduct your own experiment and learn more about the kidneys! Follow along and get creative, or use with the kidney science lesson. Get PDF. BE THE FIRST TO KNOW! Get helpful tips and FREE resources delivered to your inbox. Sign Up Now. About Us. Our Mission.
Most of the water and necessary nutrients are reabsorbed back into the blood as the filtrate moves along the renal tubules; thus, the kidney can control water balance by changing the amount of ...
The kidneys also balance the volume of fluids and minerals in the body. This balance in the body is called homeostasis (say: hoh-mee-oh-STAY-sus). If you put all of the water that you take in on one side of a scale and all of the water your body gets rid of on the other side of a scale, the sides of the scale would be balanced.
Conduct an experiment on how fast the kidneys process different fluids. Drink a liter of water quickly. Wait 20 minutes and then collect your urine in a beaker. Record the volume and color. Use a pH testing strip to record the pH as well. Repeat four times so that you have five samples collected 20 minutes apart.
Hypercalciuria has been linked to water balance as high urine calcium content has been demonstrated to induce renal water loss by proteolysis of aquaporin-2 and thereby limiting the maximal ...
Now that you know how amazing kidneys are, you can take action to help keep your kidneys healthy by making fit choices. Being active, choosing healthy foods, and drinking plenty of water are great choices you can make to keep your kidneys and body healthy. Keeping kidneys hydrated is really important. Thirst, nausea, headaches, and even ...
The renal system consists of the kidney, ureters, and the urethra. The overall function of the system filters approximately 200 liters of fluid a day from renal blood flow which allows for toxins, metabolic waste products, and excess ion to be excreted while keeping essential substances in the blood. The kidney regulates plasma osmolarity by modulating the amount of water, solutes, and ...
The bicarbonate buffer system is an acid-base homeostatic mechanism involving the balance of carbonic acid (H2CO3), bicarbonate ion (HCO3-), and carbon dioxide (CO2) in order to maintain pH in the blood and duodenum, among other tissues, to support proper metabolic function. Catalyzed by carbonic anhydrase, carbon dioxide (CO2) reacts with water (H2O) to form carbonic acid (H2CO3), which in ...