- Open access
- Published: 24 July 2023

Severity of waterborne diseases in developing countries and the effectiveness of ceramic filters for improving water quality
- Godfrey Michael Shayo ORCID: orcid.org/0000-0001-6082-5897 1 ,
- Elianaso Elimbinzi 1 ,
- Godlisten N. Shao 1 &
- Christina Fabian 1
Bulletin of the National Research Centre volume 47 , Article number: 113 ( 2023 ) Cite this article
7972 Accesses
16 Citations
Metrics details
It is anticipated that three (3) billion people will experience water stress by 2025 due to limited access to clean water. Water-related diseases and fatalities affect both industrialized and developing countries. Waterborne diseases are challenging worldwide, especially in developing countries. This article evaluates strategies used by various countries, particularly developing countries, to combat waterborne diseases. These strategies have been largely successful in reducing the prevalence of water-related diseases in developing countries.
Main body of the abstract
The effectiveness of these strategies is evaluated in terms of their ability to remove water contaminants such as bacteria, viruses, and chemicals. Different strategies can be used, including traditional water treatment techniques such as boiling, chlorination, flocculation, solar disinfection and ceramic-based water filtration systems. These methods can help improve water quality and safety. The choice of strategy depends on the specific contaminants in the water and the desired outcome. Proper implementation of these strategies is key to ensuring safe drinking water.
Short conclusion
It was revealed that in developing countries, multiple water treatment techniques are used. This has led to the reduction in waterborne diseases from 50 to 90%. Ceramic-based water purification systems are reportedly the modern and least expensive technique, since they are highly efficient and can be made locally. Thus, ceramic water filtration systems are widely used due to their affordability and easy maintenance.
Waterborne diseases are conditions caused by pathogenic microorganisms such as bacteria, protozoa, and viruses transmitted through water. When measures are delayed, these pathogens may cause adverse effects on human health, such as disability, illness, disorders, or death (Landrigan et al. 2020 ). Transmission of these pathogens occurs while using infected water for drinking, food preparation, and washing clothes (WHO 2022 ). However, most waterborne infections are spread by the fecal–oral pathway, which happens when human feces are consumed by drinking contaminated water or eating infected food, which is mostly caused by inadequate sewage management and sanitation. Waterborne pathogens, which accelerate waterborne diseases, significantly affect people’s health by causing mortality and morbidity (Ferreira et al. 2021 ; Gall et al. 2015a , b ; Shailemo et al. 2016 ). Waterborne diseases can cost people their lives and their socioeconomic status. Several research reports, government and non-government resources demonstrate this quietly. Access to clean water and sanitation facilities is essential for the prevention of waterborne diseases and the protection of public health. Proper management of water resources is critical for the prevention and control of waterborne diseases. Water quality monitoring and surveillance is necessary to protect public health.
Globally, 2.1 billion people lack access to clean and safe drinking water, resulting in 2.2 million deaths from waterborne diseases each year (UN 2019 ). Domestic water supplies must be free of disease-carrying microbes and other chemical contaminants to be safe for human consumption. It was once anticipated that until 2021, only 44% of the world’s population would have access to safe sources of water. This left a larger population, i.e., 56% of the world’s population, with access to unsafe and contaminated water from sewage, septic tanks, latrines, agricultural activities, and other human activities (World Health Organization 2020 ). Contamination of surface and groundwater ensures that waterborne diseases persist, particularly in developing countries. Currently, the global picture of water and health has a strong local dimension, with 1.1 billion people still lacking access to improved drinking water sources and 2.4 billion to adequate sanitation. There is extensive evidence that water-related, sanitation, and hygiene-related diseases account for 2.2 million deaths annually and an annual loss of 8.2 million disability-adjusted life (Anyango 2019 ; Kätzl 2019 ). The severity is much higher in developing countries than developed countries.
Waterborne diseases are one of society's most persistent and economically disastrous biological threats. Four-fifths of all illnesses in developing countries are caused by waterborne diseases, with diarrhea being the leading cause of childhood deaths (Luby et al. 2018 ). Generally, 1.8 million people die every year from waterborne diseases including cholera, typhoid, urinary tract infections, schistosomiasis and other diarrheal diseases. Nevertheless, waterborne diarrhea remains a prominent cause of mortality and sickness among children in developing nations, with 90% of diarrhea fatalities occurring in children under five. Rural residents in developing countries use discharge near or around neighboring shrubs and jungles for defecation, which results in fecal pollution of water in rural African and other developing-country locations. (Manetu and Karanja 2021 ). Common waterborne diseases include bacteria-caused diseases such as cholera, typhoid, and diarrhea, protozoa such as amoebiasis, and viral diseases such as retrovirals, hepatitis A, hepatitis E, and polio infections.
In contrast to many other outbreaks of diseases with incurable diagnoses or expensive preventions and treatments (Paliwal 2021 ), waterborne infections can be combated with local, affordable resources, minimal lifestyle changes, culturally relevant solutions, and clear and affordable awareness campaigns. Due to their ambiguity and variable applicability to different societies, environments, and durations, these sorts of solutions are called acceptable strategies. This paper reviews several strategies on their efficacy in combating waterborne diseases, particularly in rural regions of developing countries. Researchers have reported on several different strategies previously. A number of suggestions are provided, especially for developing countries that still suffer the brunt of waterborne disease. Finally, it suggests cost-effective and easy strategies when employed.
Severity of waterborne diseases in the world, developing countries and rural areas
Waterborne infections are transmitted through infected drinking water and food sources. The major causes of contamination are poor hygiene and sanitation. According to the World Bank, 2.6 billion people worldwide lack access to basic sanitation, which is defined as a clean and safe toilet or latrine (Gall et al. 2015a , b ; Weststrate et al. 2019 ). As a result, more than a quarter of the world's population must defecate behind buildings, in fields, or near communal water supplies. Disease transmission is significant when fecal matter is not properly disposed of. Infection and sickness can result from unintentional contact with excrement by people or other living things like pets or flies. In addition, using untreated human waste as fertilizer in agricultural techniques results in many infectious diseases. Additionally, due to a lack of control over the movement and habitat of most animals, pollution of nearby water sources by the feces of both domesticated and wild animals is a significant issue that is frequently more challenging to manage (Diedrich et al. 2023 ) .
Around 15% of the world's population lives in water-stressed areas (Javed and Kabeer 2018 ). Rural areas in developing nations lack access to reliable clean water supply points. Thus, they are vulnerable to waterborne diseases (Gwenzi and Sanganyado 2019 ). On the other hand, around 2.5 billion people lack access to proper sanitation, and 2–2.5 million people die from diarrhea each year (Javed and Kabeer 2018 ). Therefore, most people in these places drink untreated water from readily available contaminated sources, putting them at risk of contracting waterborne diseases. Generally, contaminated water is commonly used as a medium for disease transmission (Shailemo et al. 2016 Ali and Ahmad 2020 ).
The prevalence of waterborne intestinal pathogens such as bacteria, viruses and protozoa in domestic water sources poses a serious health risk to humans (Wen et al. 2020 ). The majority of outbreaks, though infrequent, are usually associated with sewage-contaminated or inadequately treated water. Figure 1 illustrates the transmission of waterborne diseases in the human population. Contaminated water sources serve as the primary reservoir for various contaminants, including bacteria, viruses and chemicals. These contaminants can enter the human body through ingestion, inhalation, or contact with contaminated water. Inadequate sanitation and poor hygiene practices further facilitate the spread of waterborne diseases. Once inside the body, these pathogens can cause a range of illnesses, such as gastroenteritis, cholera, hepatitis, and parasitic infections. Effective prevention and control measures, such as access to clean drinking water, proper sanitation systems, education on hygiene practices and the employment of water treatment techniques such as filtration, are crucial for reducing the incidence and impact of waterborne diseases. By addressing these factors, we can safeguard public health and promote a safer and healthier environment. Furthermore, sewage system failure and overpopulation raise the danger of infectious disease transmission, either via the virtual presence of a large number of bacteria in the environment or through contaminated drinking water (Mwambete and Tairo 2018 ).
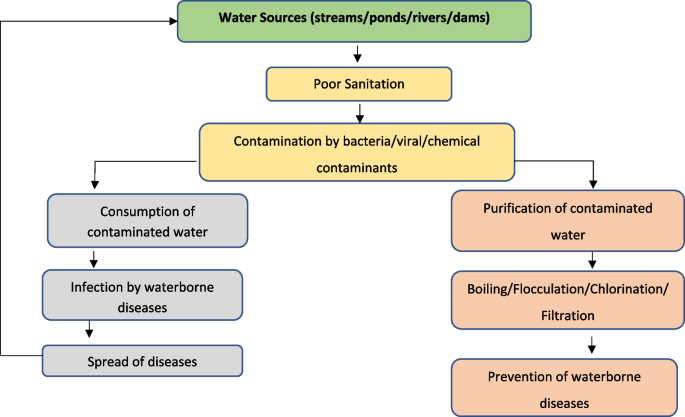
Schematic presentation of waterborne diseases transmission in human being
Although access to clean water is somehow managed in urban areas of developing countries, the situation is still poor or non-existent in rural parts of these countries (Murei et al. 2022 ). Approximately, 49% of unimproved sources, such as dug wells, natural springs, and other surface water sources are observed in rural areas. Diarrhea occurs worldwide and causes 4% of all deaths and 5% of disability loss. For example, in Bangladesh, 35 million people are daily exposed to elevated arsenic levels in their drinking water. This will ultimately threaten their health and shorten their life expectancy (World Health Organization 2020 ). Infection is common in low-income and middle-income countries with poor sanitary conditions and hygiene practices, where most children almost 90%, have been infected with the hepatitis A virus before 10 years, most often without symptoms (WHO 2022 ). Infection rates are low in high-income countries with proper sanitary and hygiene conditions.
Strategies in combating waterborne diseases
There are ways for disadvantaged people all over the world, especially those living in rural regions, to get access to clean water for drinking and other household needs. These may be referred to point-of-use (POU). Several domestic treatment methods, including boiling, sun disinfection, filtration, chemical disinfection like chlorination and flocculation, and/or sedimentation, have been implemented by several developing nations as part of their adaptation to treatment tactics (Branz et al. 2017 ; Lantagne and Yates 2018 ). The main treatment methods are shown in Table 1 along with each method's characteristics. People use these methods to prevent waterborne illnesses. Results for addressing various water pollutants, such as color, total solids, turbidity, and odor, are highly encouraging. However, in some cases, they cannot remove other water contaminants such as virus, chemicals that is, chlorine, heavy metals and other organic contaminants and bacteria contaminants. This leaves it up to researchers to investigate the efficacy of creating a ceramic filtration system with multiple capabilities for water purification. This includes the incorporation of nanomaterials like silver, copper, and gold to remove bacterial and pathogenic microorganisms. However, the incorporation of hydroxyapatite helps to remove heavy metal chemical contaminants and improve pores structure for correction of color, pH, turbidity, total dissolved solids and biological oxygen demagnetization.
Water quality and resource protection are still funded by international and non-governmental groups. Several cases of aquatic infectious diseases have been documented (Annan et al. 2018 ). Incorporating nanoparticles of noble metals into filtration technology seems to be a viable option. Some studies have reported the removal of viral and chemical contaminants through doping conventional ceramic water filters with metal oxide. Conventional ceramic water filters have been advantageous in the filtration of some water contaminants, such as bacteria, protozoa and other contaminants with ≥ 2 µm diameter size (Nigay et al. 2019 ). Recently, some studies have reported the removal of viruses through doping of standard ceramic water filters with metal oxides, such as aluminum oxide, magnesium oxide, iron oxide and titanium oxide (Mutuma et al. 2015 ; Nigay et al. 2019 ; Shao et al. 2014 , 2015 ) and chemical contaminants through hydroxyapatite (HA) doping (Haider et al. 2019 ; Nigay et al. 2019 ; Farrow et al. 2018 ). For a decade, the filtration of water contaminants such as physical, chemical and biological contaminants has been in practice in several countries. This is to address the problem of lack of safe and clean drinking water.
Viral waterborne diseases in developing countries
Viruses are the tiniest microorganisms of all parasites, with an approximate size ranging from 0.03 to 0.1 µm. Viruses are present in drinking water sources but their impact on human health is less widely understood and acknowledged. However, swallowing them can have major health consequences (Gall et al. 2015a , b ; Adelodun et al. 2021 ). More than 100 different human and animal enteric viruses have been identified as water transmissible. Rotavirus, enterovirus, norovirus and hepatitis A and E are all viral infections spread through water. Researchers have had limited success in deactivating or eliminating viruses from drinking water (Annan et al. 2018 ). Surface water contamination with enteric viruses due to human waste disposal is a public health hazard. This is especially true if these surface waterways are used for recreational, irrigation or drinking water production (Gall et al. 2015a , b ; McKee and Cruz 2021 ). Polluted water transfers viruses, including drinking and recreational water. Outbreaks involving huge numbers of diseased people are typical because numerous people may ingest a batch of water or come into contact with contaminated materials (McKee and Cruz 2021 ). Viral gastroenteritis outbreaks are mostly caused by norovirus, whereas viral hepatitis outbreaks are mostly caused by Hepatitis A Virus and rarely by Hepatitis E Virus (Bosch et al. 2011 ; McKee and Cruz 2021 ).
Viral infections, particularly those caused by rotavirus, are the most common causes of acute diarrheal diseases. Over half a million people worldwide die each year from the rotavirus, which is so pervasive that it infects almost every child by the age of five (Charoenwat et al. 2022 ). Typically, viral hepatitis affects the liver. It can be acute (fresh infection, fast onset) or chronic (long onset) (Aggarwal 2011 ; Kim et al. 2021 ). Infection with one of the five known hepatotropic viruses (hepatitis A, B, C, D and E viruses) causes viral hepatitis. Viral-based waterborne diseases can also be transmitted through inhalation or contact with skin and eyes which can both spread viruses, resulting in respiratory and ocular diseases. For healthy people, viral infections are typically self-limiting, but in children under five, the elderly, immune-compromised adults and pregnant women, they are at higher risk (Gall et al. 2015a , b ). Waterborne virus-based infections may be more common in developing countries, where hunger is common, and there are huge populations of HIV-positive (Gall et al. 2015a , b ; WHO 2022 ).
For this paper, only waterborne hepatitis viruses A and E will be discussed. In populations with unsafe water and inadequate sanitation, viral hepatitis A and E are food and waterborne diseases that can cause acute epidemics. They do not cause chronic infection or liver damage, and there is no treatment for them. Improvements in sanitation, food safety, and immunization are all effective prevention methods (Aggarwal 2011 ; Kim et al. 2021 ). The most typical clinical outcome of hepatitis A or E virus infection is a sickness typified by an abrupt onset of fever and systemic symptoms, followed by jaundice a few days later.
Hepatitis A and E viral waterborne diseases
Hepatitis A is a self-limiting liver illness caused by Hepatitis A virus infection. Hepatitis A viral infection spreads by the fecal–oral route, which can be transmitted directly from person to person or indirectly through the intake of feces-contaminated food or water (Foster et al. 2019 ). Because the hepatitis A virus is abundantly discharged in feces and may live in the environment for extended periods of time, it is usually a food-waterborne illness (Foster et al. 2019 ; Gullón et al. 2017 ). In regions where sanitation is inadequate and living conditions are dense, infections arise early in life. Infections are delayed due to increased sanitation and hygiene, and the number of people vulnerable to the disease rises (Gullón et al. 2017 ). In these circumstances, fecal contamination from a single source might result in explosive epidemics. Adults are increasingly contracting hepatitis A virus infections in most developed countries, where hepatitis A is no longer considered a childhood illness (Foster et al. 2019 ; Gullón et al. 2017 ).
Hepatitis E is an acute hepatitis caused by the Hepatitis E Virus infection. The virus spreads predominantly by the fecal–oral route, and it is extremely prevalent in certain underdeveloped nations where drinking water might be contaminated (Aggarwal 2011 ; Magana-Arachchi and Wanigatunge 2020 ). It manifests itself as outbreaks and occasional instances of acute hepatitis in these highly endemic locations. The illness is usually self-limiting and resembles other hepatotropic viruses. However, in some cases, the condition progresses to severe liver failure (Magana-Arachchi and Wanigatunge 2020 ). The Indian subcontinent, China, Southeast and Central Asia, the Middle East and northern and western Africa are all highly endemic to hepatitis E (Yekta et al. 2021 ). Hepatitis E outbreaks of various magnitudes have been documented in these regions. Furthermore, hepatitis E virus infection is responsible for a substantial number of sporadic acute hepatitis cases in these locations. The most prevalent mode of illness transmission in these places is water (Yekta et al. 2021 ). The hepatitis E virus has been linked to a 25% mortality rate in pregnant women (World Health Organization 2022 ). Several strategies have been discussed to combat hepatitis A and E viral waterborne infections including physical elimination, chemical treatment and UV light disinfection.
Strategies for combating viral waterborne diseases in developing countries
In the elimination of viral water contaminants from drinking water, several strategies have been used. However, there are two common and effective strategies used in the world and particularly in developed countries, which are physical elimination of pathogens by conventional treatment and the inactivation of viral pathogens using ultraviolet irradiation or chemical oxidants such as chlorine, chloramines, ozone and chlorine dioxide (Gall et al. 2015a , b ). Because viruses are so small, conventional treatment methods, such as filtration, are unsuccessful in physically eliminating them (Gall et al. 2015a , b ; Nigay et al. 2019 ). Disinfectants are heavily dependent on water chemistry and local restrictions. A common disinfection technique in recent years has been chlorination, where free chlorine is derived from hypochlorous acid and hypochlorite ions that are dissolved in water and hydrolyzed. This strategy has been used to disinfect water since the early 1900s (Branz et al. 2017 ; Gall et al. 2015a , b ; Lantagne and Yates 2018 ). This powerful oxidant renders most viruses dormant. However, free chlorine treatment may release harmful disinfection by-products and fails to control Cryptosporidium, a protozoan that causes diarrhea and spreads through water (Khan et al. 2019 ; Gall et al. 2015a , b ). To control the formation of regulated toxic disinfection by-products, some drinking water utilities are switching to monochloramine which is formed by mixing chlorine and ammonia with the latter in slightly excess; and/or either monochromatic (254 nm) or polychromatic (200–300 nm) ultraviolet (UV) light to control both disinfection by-products formation and Cryptosporidium contamination. In spite of these modifications to the disinfection method, the UV light technique comes with a very high cost for virus control compared to other conventional methods (Gall et al. 2015a , b ; Ibrahim et al. 2021 ).
In order to deal with the viral-based waterborne situation, total abstinence from all water sources such as streams, ponds, rivers and lakes is necessary, as well as other water sources that may be contaminated by waterborne pathogens and other chemicals. With a variety of methods, some developed countries, such as the United States, Canada, the Netherlands, and Western Australia, have shown efficiency in wastewater treatment. This is due to differences in socioeconomic factors (Ferreira et al. 2021 ). Most waterborne illnesses are not prevalent in developed countries because of sophisticated water systems that filter and chlorinate water to eradicate all disease-carrying organisms. In developing countries, however, waterborne diseases such as Hepatitis A and E, remain prevalent. The strategies employed in developed countries may not be feasible, particularly in rural areas where proper sanitation and infrastructure for water management are difficult to attain (Levy et al. 2018 ). As a result, this review recommends using point-of-use water treatment technology as a replacement, particularly for ceramic water filters that can be produced at a price affordable for rural residents when doped with metal oxides like alumina, titania, iron oxide, zinc oxide, or magnesium oxide (Mutuma et al. 2015 ; Nigay et al. 2019 ).
Bacterial waterborne diseases
Bacteria are single-celled or non-cellular, spherical, spiral or rod-shaped microorganisms that reproduce by fission and are key pathogens and biochemical characteristics. Bacteria are well-known diarrhea-causing diseases transmitted through contaminated drinking water. Depending on the bacteria kind and number present, these bacteria may or may not be detrimental, but the cumulative effect might be devastating. Bacteria are generally between 0.5 and 2 µm long (Annan et al. 2018 ). Vibrio cholerae , Salmonella sp., Campylobacter sp., Shigella sp., and Staphylococcus aureus are all bacteria spread through water. Coliform bacteria are a group of microorganisms found in the environment and mammals' intestines. They are usually harmless, but their presence indicates that drinking water's microbiological quality is of concern (Mwambete and Tairo 2018 ; World Health Organization 2006 ). Some coliforms bacteria include Escherichia, Serratia, Enterobacter, Proteus, Klebsiella, Citrobacter, Yersinia and Hafnia species . However, E. coli is the only member found in the intestines of mammals including humans; thus, its presence indicates recent fecal contamination and the possible presence of other waterborne pathogens.
Drinking water is a significant vehicle for bacterial waterborne infections such as cholera, diarrhea and typhoid fever (Gwenzi and Sanganyado 2019 ; Mwambete and Tairo 2018 ; World Health Organization 2006 ). Cholera is caused by the bacteria Vibrio cholerae , which causes severe diarrhea, vomiting, dehydration and death. It can be severe if not treated properly, up to 50% of the time. However, medication can reduce the severity to as little as 1% of the time. Cholera causes 100,000 deaths worldwide (Lee et al. 2017 ). Salmonella typhi bacteria are the source of the potentially fatal bacterial infection known as typhoid fever. There are still roughly 21 million cases of typhoid fever each year in developing nations. Only people carry Salmonella typhi . Typhoid fever patients have bacteria in their blood and intestines. Few people, called carriers, recover from typhoid fever but still carry the germ. Sick persons and carriers excrete S. typhi in their stools. Consuming or drinking food or beverages that have been touched by someone shedding S. typhi bacteria or drinking or washing food with sewage contaminated with S. typhi bacteria can result in typhoid fever (Brockett et al. 2020 ).
In developing countries, E. coli is the most common cause of diarrheal disease infections and human gastrointestinal tract infections caused by ingesting contaminated water (Gwenzi and Sanganyado 2019 ). In Africa, for instance, a severe cholera epidemic broke out in Zimbabwe in 2008 and quickly spread to neighboring nations (Zambia, Botswana, Mozambique and South Africa). Due to poor sanitation and waste management practices and a limited supply of clean piped water, the scarcity of safe drinking water in Zimbabwe's urban areas had a significant role in the development and spread of the disease. Poor water sanitation and hygiene are linked to a higher proportion of intestinal parasitic infections, with the majority being fecal–oral (Gwenzi and Sanganyado 2019 ; Gwimbi et al. 2019 ). In rural regions of most developing nations, where water supplies are communally shared and exposed to many fecal–oral transmission paths within their neighborhood boundaries, bacterial contamination of drinking water is a major contributor to waterborne illnesses (Reece et al. 2017 ; Iwu and Okoh 2019 ). E. coli infections linked to polluted water continue to be a serious public health problem, as their presence indicates the prevalence of deadly disorders such as diarrhea (Iwu and Okoh 2019 ). Despite the fact that the endemicity and intensity of bacterial waterborne illnesses have decreased in developing countries, the case fatality rates in cholera cases remain significantly higher in Africa (about 60%) than in Asia (29%) (Montufar-Salcedo, 2018 ). However, the World Health Organization (WHO) reports that 1.3 million suspected cases of typhoid fever have been recorded in Africa since 2021, with 502 deaths (2%) out of 30,934 confirmed cases in DRC. These are the most common bacterial-associated waterborne diseases in most developing countries (Gwimbi et al. 2019 ).
Strategies for combating bacterial waterborne diseases
Recently, bacterial-based water contaminants have been solved thanks to the availability of common point-of-use water treatment technologies. In most developing countries, the technologies include boiling, chlorination of contaminated water, solar disinfection and filtration techniques such as bone char, bio-sand, slow sand, membrane purifiers and ceramic filters (Farrow et al. 2018 ). Although all of them work effectively in bacterial removal, ceramic filters are perceived by most users and developers due to their easy and affordable cost of fabrication, as they require the availability of regional materials such as clay, soil, sawdust, starch, wheat flour, and milled rice husk which hence makes their dissemination to people cost-effective and economically sound. The incorporation of noble metals into ceramic water filters ensures the efficient functioning or performance of the filters, this is to say, by increasing bacterial disinfection or by increasing bio-film disinfection ability. Metal oxide nanoparticles' antibacterial capabilities, manufacturing techniques and microorganisms removed during water treatment are summarized in Table 2 .
From Table 2 , based on the different Lewis-dot structures, metal oxides display diverse physicochemical and functional properties, including magnetic, optical, mechanical, and electrical properties/features (Raghunath and Perumal 2017 ). They have shown the ability to interact with bacteria through electrostatic interactions through prokaryotic cell walls and enzyme or DNA alteration through reactive oxygen species (ROS) production (Gold et al. 2018 ). Under light exposure (He et al. 2016 ), magnesium oxide nanoparticles act as antibacterial agents and produce ROS. The ROS then enters the bacterial cell membrane while reducing both oxidative stresses on the cell organelles and lipid peroxidation, thereby preventing oxidative degradation of lipids (Gold et al. 2018 ). Since titania is a strong photocatalytic material with high oxidizing power and long-term stability, it can generate ROS with a wavelength of around 320–385 nm, hence its ability as an antimicrobial agent (Kumaravel et al. 2021 ). The action of metal oxide antimicrobial agents involves several working mechanisms, including cell membrane damage due to electrostatic interaction, disruption in metal/metal ion homeostasis, production of ROS and oxidative stress, protein and enzyme dysfunction, genotoxicity, signal transduction inhibition, and photo-removal (Raghunath and Perumal 2017 ).
However, from Table 2, it is anticipated that a higher concentration of MgO inhibits bacteria’s growth against E. coli which is higher than Bacillus sp . On the other hand, CuO provides more room to be used as a biocidal agent, such as against B. subtilis. This is due to its cost-effectiveness and better biocidal ability than other noble metal oxides (Hoseinnejad et al. 2018 ). In many studies, ZnO is proposed to have higher antibacterial ability than other metal oxides since they can pose a threat to both gram-positive and gram-negative bacteria. Furthermore, Al 2 O 3 at high concentrations has mild deactivation properties owing to the free radical scavenging capability of nanoparticles that prevent cell wall disintegration (Makvandi et al. 2020 ). Nevertheless, Al 2 O 3 has also been suggested to trap viral contaminants due to its positively charged surface (Nigay et al. 2019 ).
Chemical contamination of water
Water is a carrier of infectious microorganisms such as bacteria, parasites and viruses that spread via the fecal–oral route in water-based diseases. Similarly, chemicals are sometimes thought to be a source of infectious agents (Javed and Kabeer 2018 ). Water-stressed areas are home to about 15% of the world's population. Waterborne diseases are caused by chemical toxins, mostly found in industrial, municipal, and agricultural wastes (Javed and Kabeer 2018 ). For instance, heavy metals such as chromium, cadmium, nickel, lead, mercury and arsenic; cations, such as sodium, potassium, and calcium; anions, such as carbonates, bicarbonates, and nitrates; and pesticides, such as dichlorodiphenyltrichloroethane and benzene hexachloride enter water bodies from point and non-point sources and cause several health complications among people in many developing countries (Syafrudin et al. 2021 ).
Pesticide use has a number of advantages, including better food quality and quantity and reduced insect-borne diseases, but it has also prompted concerns about potential negative impacts on the environment, especially water sources (Syafrudin et al. 2021 ). Pesticides end up in bodies of water due to runoff from agricultural fields and industrial waste. Soluble pesticides are taken away by water molecules, which percolate lower into the soil layers and eventually reach surface waters and groundwater (Syafrudin et al. 2021 ). As a result, water quality deteriorates and drinkable water quantity decreases. Drinking water contaminated with heavy metals, pesticides, cations, and anions causes life-threatening complications in the gastrointestinal, renal, cardiovascular, pulmonary, and reproductive systems (Syafrudin et al. 2021 ). Furthermore, chemicals carried by polluted water can cause urinary tract burning and calculi, leukomelanosis, hyperkeratosis, black foot disease, neuropathy and cancer (Javed and Kabeer 2018 ; Syafrudin et al. 2021 ).
Chemicals in drinking water that exceed allowable levels may harm human health. This could be caused by human activities or natural occurrences. Chemical pollutants in drinking water have also been linked to a wide range of negative health impacts, including cancer, cardiovascular illness, neurological disease, and miscarriages. Leaching, spills, runoff, and air deposition are ways through which chemicals enter water systems (Annan et al. 2018 ). Heavy metals are found naturally in the earth's crust and are long-lasting environmental pollutants since they cannot be degraded or removed. They enter the human system in tiny amounts from food, air, and water, and bioaccumulate over time (Ali et al. 2017 ; Annan et al. 2018 ). Table 3 shows WHO and USEPA maximum permissible heavy metals in drinking water values.
With an acceptable concentration of 0.002 mg/L poisonous level, thallium and mercury are the most poisonous metals (Table 3 ). This puts human health at high risk compared to all other metals mentioned in the table. While nitrate, with a WHO rating of 11.3 mg/L and a USEPA rating of 10.0 mg/L, represents the highest allowable chemical concentration in the human body. Chemical contaminants in drinking water can pose a threat to human health sometimes, but the human body needs several heavy metal elements in their divalent cation forms, such as Zn 2+ , Fe 2+ and Cu 2+ . For instance, these metal divalents are required by the human body in the regulation of numerous physiological functions. These functions include protein and nucleic acid synthesis, antioxidant defense, and membrane stabilization. However, these metal divalents are required by the human body at very low concentration (Ali et al. 2017 ; Rehman et al. 2021 ). If their concentration exceeds the body's requirement level, metal divalent leads to health effects (Ali et al. 2017 ). Other heavy metals are poisonous to humans, such as Cd 2+ , Pb 2+ , Co 2+ , Pt 2+ and Ni 2+ . When the human body is contaminated with these metals, the kidneys, for instance, suffer the most. Hence, several effects are observed, including a decrease in essential elements entry due to heavy metal competition (Ali et al. 2017 ; Rehman et al. 2021 ).
Strategies for combating chemical contamination of water
Several studies have reported some positive progress advances in the discovery of therapeutic tools, such as cell protectors and metal chelators. These tools can be administered when an individual has taken the chemicals in any way, particularly through contaminated drinking water. But treatment must be a last option if, at all costs, the situation can be prevented from happening. Studies have reported developing point-of-use water treatment technologies, such as ceramic water filters, among many others as speculated in Table 1 , being more feasible for many people due to their low cost and ease of fabrication (Gupta et al. 2018 ; Farrow et al. 2018 ). Ceramic filters can be boosted in their efficiency in the removal of heavy metals, pesticides, and organic chemical contaminants when doped with hydroxyapatite chemicals, and the chemical materials made from bones (Haider et al. 2019 ; Nigay et al. 2019 ; Farrow et al. 2018 ). Nigay et al. ( 2019 ) reported that through a substitution mechanism, HA chemicals can interchange their chemical contents, that is, calcium ions, hydroxyl groups, and phosphate groups, with the heavy metal chemicals present in the contaminated water (Nigay et al. 2019 ).
Future prospect
Ceramic water filters, as used in many developed countries such as the USA, Netherlands, Canada, and Western Australia, can be used in developing countries with some modifications. This will improve performance and efficiency at the point-of-use. Conventional ceramic water filters can improve water quality in several parameters but fail in others. For instance, most bacterial contaminants can be physically filtered through conventional ceramic water filters. However, after some time of filter use, bacteria and mold grow on the surface of the system. Incorporating ceramic water filters with noble metals such as silver, copper, or gold in their nanoparticle form removes bacteria and prevents the system from becoming infected with protozoa (Loza et al. 2020 ; Praveena and Aris 2015 ). However, for several years, viral-based contaminants have been linked to hepatitis A and E diseases, which may cause liver cancer if chronic. Removal of viruses is quite challenging due to their small size, so they cannot be removed through physical strains. However, doping ceramic water filters with metal oxides including titania, alumina, magnesium oxide, or iron oxide facilitates the adsorption of viruses from water (Haider et al. 2019 ; Mutuma et al. 2015 ; Shao et al. 2015 ). This is due to the fact that viruses have negative surface charges and hence can be attracted to metal oxides, which are positively charged. Additionally, chemicals can be removed from water by hydroxyapatite chemicals (Haider et al. 2019 ; Nigay et al. 2019 ; Farrow et al. 2018 ). Doping ceramic water filters with hydroxyapatite is feasible and increases chemical removal efficiency. Therefore, the feasibility of having one system that simultaneously removes bacterial, viral, and chemical contaminants is quite possible. This is when a ceramic water filter is incorporated with noble metal nanoparticles and doped with metal oxides and hydroxyapatite.
Conclusions
Regardless of the disinfection method employed by a drinking water utility, cross-contamination can happen throughout the water distribution infrastructure. This is due to cavitation and unintended depressurization when treated water moves from the treatment facility to the point-of-use. However, because municipal water services are typically not available in poor nations, residents must acquire water from other nearby sources. Most of these sources are tainted with pollutants and bacteria that cause waterborne illness. The World Health Organization estimated in 2017 that environmental changes including expanding access to clean drinking water and raising sanitation and hygiene standards may prevent 94% of cases of waterborne diarrhea diseases. However, the increasing water availability, sanitation, hand washing, and domestic water treatment and safe storage can reduce diarrhea episodes by 25%, 32%, 45% and 39%, respectively. Although, these distribution systems need additional disinfectants. This review also offers recommendations for how developing nations can lower waterborne illnesses prevalence. These include raising the quantity and quality of drinking water, ensuring safe sewage disposal, and offering accessible, affordable sanitation solutions. For example, the adoption of point-of-use water treatment technologies. These technologies are simple, low-cost, and have the potential to reduce waterborne illnesses significantly. Furthermore, these solutions should be combined with educational campaigns to ensure that people are aware of how to use and maintain the technologies.
Availability of data and materials
Not applicable.
Abbreviations
Reactive oxygen species
Escherichia coli
United State Environmental Protection Agency
World Health Organization
Point of use
Ceramic water filters
Human immunodeficiency virus
Hydroxyapatite
Ultraviolet light
Hepatitis A viruses
Hepatitis E viruses
United Nation
Adelodun B, Ajibade FO, Ighalo JO, Odey G, Ibrahim RG, Kareem KY et al (2021) Assessment of socioeconomic inequality based on virus-contaminated water usage in developing countries: a review. Environ Res 192:110309
Article CAS PubMed Google Scholar
Aggarwal R (2011) Clinical presentation of hepatitis E. Virus Res 161(1):15–22. https://doi.org/10.1016/j.virusres.2011.03.017
Aggarwal R, Naik S (2009) Epidemiology of hepatitis E: current status. J Gastroenterol Hepatol 24(9):1484–1493. https://doi.org/10.1111/j.1440-1746.2009.05933.x
Article PubMed Google Scholar
Ali A, Ahmed A, Gad A (2017) Chemical and microstructural analyses for heavy metals removal from water media by ceramic membrane filtration. Water Sci Technol 75(2):439–450. https://doi.org/10.2166/wst.2016.537
Ali SA, Ahmad A (2020) Analysing water-borne diseases susceptibility in Kolkata Municipal Corporation using WQI and GIS based Kriging interpolation. GeoJournal 85(4):1151–1174
Article Google Scholar
Annan E, Agyei-Tuffour B, Bensah YD, Konadu DS, Yaya A, Onwona-Agyeman B, Nyankson E (2018) Application of clay ceramics and nanotechnology in water treatment: a review. Cogent Eng 5(1):1476017
Anyango MJ (2019) Water, sanitation and hygiene practices as predictors of diarrhoea occurrence among school age children in Ganze Sub County, Kenya. 206
Azam A, Ahmed AS, Oves M, Khan MS, Habib SS, Memic A (2012) Antimicrobial activity of metal oxide nanoparticles against Gram-positive and Gram-negative bacteria: a comparative study. Int J Nanomed. https://doi.org/10.2147/IJN.S35347
Bitew BD, Gete YK, Biks GA, Adafrie TT (2018) The effect of SODIS water treatment intervention at the household level in reducing diarrheal incidence among children under 5 years of age: a cluster randomized controlled trial in Dabat district, northwest Ethiopia. Trials 19(1):412. https://doi.org/10.1186/s13063-018-2797-y
Article PubMed PubMed Central Google Scholar
Bosch A, Sánchez G, Abbaszadegan M, Carducci A, Guix S, Le Guyader FS, Netshikweta R, Pintó RM, van der Poel WHM, Rutjes S, Sano D, Taylor MB, van Zyl WB, Rodríguez-Lázaro D, Kovač K, Sellwood J (2011) Analytical methods for virus detection in water and food. Food Anal Methods 4(1):4–12. https://doi.org/10.1007/s12161-010-9161-5
Branz A, Levine M, Lehmann L, Bastable A, Ali SI, Kadir K, Yates T, Bloom D, Lantagne D (2017) Chlorination of drinking water in emergencies: a review of knowledge to develop recommendations for implementation and research needed. Waterlines 36(1):4–39. https://doi.org/10.3362/1756-3488.2017.002
Brockett S, Wolfe MK, Hamot A, Appiah GD, Mintz ED, Lantagne D (2020) Associations among water, sanitation, and hygiene, and food exposures and typhoid fever in Case-Control studies: a systematic review and meta-analysis. Am J Trop Med Hyg 103(3):1020
Bui XT, Nguyen PT, Nguyen VT, Dao TS, Nguyen PD (2020) Microplastics pollution in wastewater: characteristics, occurrence and removal technologies. Environ Technol Innov 19:101013
Bulta AL, Micheal GAW (2019) Evaluation of the efficiency of ceramic filters for water treatment in Kambata Tabaro zone, southern Ethiopia. Environ Syst Res 8(1):1. https://doi.org/10.1186/s40068-018-0129-6
Burleson G, Tilt B, Sharp K, MacCarty N (2019) Reinventing boiling: A rapid ethnographic and engineering evaluation of a high-efficiency thermal water treatment technology in Uganda. Energy Res Soc Sci 52:68–77
Charoenwat B, Suwannaying K, Paibool W, Laoaroon N, Sutra S, Thepsuthammarat K (2022) Burden and pattern of acute diarrhea in Thai children under 5 years of age: a 5-year descriptive analysis based on Thailand National Health Coverage (NHC) data. BMC Public Health 22(1):1–10. https://doi.org/10.1016/j.erss.2019.02.009
Diedrich A, Sivaganesan M, Willis JR, Sharifi A, Shanks OC (2023) Genetic fecal source identification in urban streams impacted by municipal separate storm sewer system discharges. PLoS ONE 18(1):e0278548
Article CAS PubMed PubMed Central Google Scholar
El-Taweel GE, Ali GH (2000) Evaluation of roughing and slow sand filters for water treatment. Water Air Soil Pollut 120(8):21–28.
Article ADS CAS Google Scholar
Farrow C, McBean E, Huang G, Yang A, Wu Y, Liu Z, Li Y (2018) Ceramic water filters: a point-of-use water treatment technology to remove bacteria from drinking water in Longhai City, Fujian Province. China J Environ Inf 32(2):63–68
Google Scholar
Ferreira DC, Graziele I, Marques RC, Gonçalves J (2021) Investment in drinking water and sanitation infrastructure and its impact on waterborne diseases dissemination: The Brazilian case. Sci Total Environ 779:146279. https://doi.org/10.1016/j.scitotenv.2021.146279
Article ADS CAS PubMed Google Scholar
Fewtrell L, Kaufmann RB, Kay D, Enanoria W, Haller L, Colford JM (2005) Water, sanitation, and hygiene interventions to reduce diarrhoea in less developed countries: a systematic review and meta-analysis. Lancet Infect Dis 5(1):42–52. https://doi.org/10.1016/S1473-3099(04)01253-8
Foster MA, Hofmeister MG, Kupronis BA, Lin Y, Xia G-L, Yin S, Teshale E (2019) Increase in hepatitis A virus infections—United States, 2013–2018. MMWR Morb Mortal Weekly Rep 68(18):413–415. https://doi.org/10.15585/mmwr.mm6818a2
Gall AM, Mariñas BJ, Lu Y, Shisler JL (2015a) Waterborne viruses: a barrier to safe drinking water. PLoS Pathog 11(6):e1004867. https://doi.org/10.1371/journal.ppat.1004867
Gall AM, Shisler JL, Mariñas BJ (2015b) Analysis of the viral replication cycle of adenovirus serotype 2 after inactivation by free chlorine. Environ Sci Technol 49(7):4584–4590. https://doi.org/10.1021/acs.est.5b00301
Ghernaout D (2014) Coagulation and chlorination of NOM and algae in water treatment: a review. Int J Environ Monit Anal 2(6):23. https://doi.org/10.11648/j.ijema.s.2014020601.14
Ghernaout D (2017) Water treatment chlorination: an updated mechanistic insight review. Chem Res J 2:125–138
CAS Google Scholar
Gold K, Slay B, Knackstedt M, Gaharwar AK (2018) Antimicrobial activity of metal and metal-oxide based nanoparticles. Adv Ther 1(3):1700033. https://doi.org/10.1002/adtp.201700033
Article CAS Google Scholar
Gullón P, Varela C, Martínez EV, Gómez-Barroso D (2017) Association between meteorological factors and hepatitis A in Spain 2010–2014. Environ Int 102:230–235. https://doi.org/10.1016/j.envint.2017.03.008
Gupta S, Satankar RK, Kaurwar A, Aravind U, Sharif M, Plappally A (2018) Household production of ceramic water filters in western Rajasthan, India. Int J Serv Learn Eng Humanit Eng Soc Entrep 13(1):53–66. https://doi.org/10.24908/ijsle.v13i1.11150
Gwenzi W, Sanganyado E (2019) Recurrent Cholera Outbreaks in Sub-Saharan Africa: moving beyond epidemiology to understand the environmental reservoirs and drivers. Challenges 10(1):1. https://doi.org/10.3390/challe10010001
Gwimbi P, George M, Ramphalile M (2019) Bacterial contamination of drinking water sources in rural villages of Mohale Basin, Lesotho: exposures through neighbourhood sanitation and hygiene practices. Environ Health Prev Med 24(1):33. https://doi.org/10.1186/s12199-019-0790-z
Haider MS, Shao G, Ahmad A, Imran SM, Abbas N, Abbas G, Hussain M, Kim HT (2019) Facile, single-pot preparation of nanoporous SiO 2 particles (carrier) with AgNPs at core and crust for controlled disinfectant release. J Saudi Chem Soc 23(7):828–835. https://doi.org/10.1016/j.jscs.2019.02.005
He Y, Ingudam S, Reed S, Gehring A, Strobaugh TP, Irwin P (2016) Study on the mechanism of antibacterial action of magnesium oxide nanoparticles against foodborne pathogens. J Nanobiotechnology 14(1):54. https://doi.org/10.1186/s12951-016-0202-0
Hoseinnejad M, Jafari SM, Katouzian I (2018) Inorganic and metal nanoparticles and their antimicrobial activity in food packaging applications. Crit Rev Microbiol 44(2):161–181. https://doi.org/10.1080/1040841X.2017.1332001
Ibrahim Y, Ouda M, Kadadou D, Banat F, Naddeo V, Alsafar H et al (2021) Detection and removal of waterborne enteric viruses from wastewater: a comprehensive review. J Environ Chem Eng 9(4):105613
Iwu CD, Okoh AI (2019) Preharvest transmission routes of fresh produce associated bacterial pathogens with outbreak potentials: a review. Int J Environ Res Public Health 16(22):4407
Javed A, Kabeer A (2018) Enhancing waterborne diseases in pakistan & their possible control. Am Acad Sci Res J Eng Technol Sci 49(1):248–256
Jeon I, Ryberg EC, Alvarez PJ, Kim JH (2022) Technology assessment of solar disinfection for drinking water treatment. Nat Sustain 5(9):801–808
Kallman EN, Oyanedel-Craver VA, Smith JA (2011) Ceramic filters impregnated with silver nanoparticles for point-of-use water treatment in rural Guatemala. J Environ Eng 137(6):407–415. https://doi.org/10.1061/(ASCE)EE.1943-7870.0000330
Kätzl K (2019) Anaerobic biochar filtration of municipal raw sewage for wastewater reuse (Doctoral dissertation, Ruhr-Universität Bochum)
Kiagho B, Machunda R, Hilonga A, Njau K (2016) Performance of water filters towards the removal of selected pollutants in Arusha, Tanzania. Tanzan J Sci 42(1):134–147
Kim JU, Ingiliz P, Shimakawa Y, Lemoine M (2021) Improving care of migrants is key for viral hepatitis elimination in Europe. Bull World Health Organ 99(4):280–286. https://doi.org/10.2471/BLT.20.260919
Khan A, Shams S, Khan S, Khan MI, Khan S, Ali A (2019) Evaluation of prevalence and risk factors associated with Cryptosporidium infection in rural population of district Buner, Pakistan. PLoS ONE 14(1):e0209188
Kumaravel V, Nair KM, Mathew S, Bartlett J, Kennedy JE, Manning HG et al (2021) Antimicrobial TiO 2 nanocomposite coatings for surfaces, dental and orthopaedic implants. Chem Eng J 416:129071
Landrigan PJ, Stegeman JJ, Fleming LE, Allemand D, Anderson DM, Backer LC, Rampal P (2020) Human health and ocean pollution. Ann Glob Health 86(1):151
Lantagne D, Klarman M, Mayer A, Preston K, Napotnik J, Jellison K (2010) Effect of production variables on microbiological removal in locally-produced ceramic filters for household water treatment. Int J Environ Health Res 20(3):171–187. https://doi.org/10.1080/09603120903440665
Lantagne D, Yates T (2018) Household water treatment and cholera control. J Infect Dis 218(suppl_3):S147–S153. https://doi.org/10.1093/infdis/jiy488
Lee EC, Kelly MR, Ochocki BM, Akinwumi SM, Hamre KES, Tien JH, Eisenberg MC (2017) Model distinguishability and inference robustness in mechanisms of cholera transmission and loss of immunity. J Theor Biol 420:68–81. https://doi.org/10.1016/j.jtbi.2017.01.032
Article ADS MathSciNet PubMed PubMed Central MATH Google Scholar
Levy K, Smith SM, Carlton EJ (2018) Climate change impacts on waterborne diseases: moving toward designing interventions. Curr Environ Health Rep 5(2):272–282. https://doi.org/10.1007/s40572-018-0199-7
Li Y, Li J, Ding J, Song Z, Yang B, Zhang C, Guan B (2022) Degradation of nano-sized polystyrene plastics by ozonation or chlorination in drinking water disinfection processes. Chem Eng J 427:131690
Loomis D, Sobsey MD, Brown J (2008) Local drinking water filters reduce diarrheal disease in cambodia: a randomized, controlled trial of the ceramic water purifier. Am J Trop Med Hyg 79(3):394–400. https://doi.org/10.4269/ajtmh.2008.79.394
Loza K, Heggen M, Epple M (2020) Synthesis, structure, properties, and applications of bimetallic nanoparticles of noble metals. Adv Func Mater 30(21):1909260. https://doi.org/10.1002/adfm.201909260
Luby SP, Rahman M, Arnold BF, Unicomb L, Ashraf S, Winch PJ et al (2018) Effects of water quality, sanitation, handwashing, and nutritional interventions on diarrhoea and child growth in rural Bangladesh: a cluster randomised controlled trial. Lancet Global Health 6(3):e302–e315
Magana-Arachchi DN, Wanigatunge RP (2020) Ubiquitous waterborne pathogens. In: Waterborne pathogens, pp 15–42. Butterworth-Heinemann
Makvandi P, Wang C, Zare EN, Borzacchiello A, Niu L, Tay FR (2020) Metal-based nanomaterials in biomedical applications: antimicrobial activity and cytotoxicity aspects. Adv Func Mater 30(22):1910021. https://doi.org/10.1002/adfm.201910021
Manetu WM, Karanja AM (2021) Waterborne disease risk factors and intervention practices: a review. Oalib 08(05):1–11. https://doi.org/10.4236/oalib.1107401
McKee AM, Cruz MA (2021) Microbial and viral indicators of pathogens and human health risks from recreational exposure to waters impaired by fecal contamination. J Sustain Water Built Environ 7(2):03121001
Mohammed Sadiq I, Chandrasekaran N, Mukherjee A (2010) Studies on effect of TiO 2 nanoparticles on growth and membrane permeability of Escherichia coli , Pseudomonas aeruginosa , and Bacillus subtilis . Curr Nanosci 6(4):381–387. https://doi.org/10.2174/157341310791658973
Article ADS Google Scholar
Montgomery MA, Elimelech M (2007) Water and sanitation in developing countries: including health in the equation. Environ Sci Technol 41(1):17–24. https://doi.org/10.1021/es072435t
Article ADS PubMed Google Scholar
Montufar Salcedo C (2018) Modification of the treatment protocol as a strategy in the control of the cholera epidemic in Haiti 2016–2017. Med Case Rep Rev 1(3):1–3
Mulugeta S, Helmreich B, Drewes JE, Nigussie A (2020) Consequences of fluctuating depth of filter media on coliform removal performance and effluent reuse opportunities of a bio-sand filter in municipal wastewater treatment. J Environ Chem Eng 8(5):104135. https://doi.org/10.1016/j.jece.2020.104135
Murei A, Mogane B, Mothiba DP, Mochware OTW, Sekgobela JM, Mudau M et al (2022) Barriers to water and sanitation safety plans in rural areas of South Africa—a case study in the Vhembe District, Limpopo Province. Water 14(8):1244
Mutuma BK, Shao GN, Kim WD, Kim HT (2015) Sol–gel synthesis of mesoporous anatase–brookite and anatase–brookite–rutile TiO 2 nanoparticles and their photocatalytic properties. J Colloid Interface Sci 442:1–7
Mwambete KD, Tairo VP (2018) Bacteriological quality of household drinking water and water disinfection practices in Kinondoni Municipality, Tanzania. Int J Health Sci 1:10
Nigay P-M, Salifu AA, Obayemi JD, White CE, Nzihou A, Soboyejo WO (2019) Ceramic water filters for the removal of bacterial, chemical, and viral contaminants. J Environ Eng 145(10):04019066. https://doi.org/10.1061/(ASCE)EE.1943-7870.0001579
Norton DM, Rahman M, Shane AL, Hossain Z, Kulick RM, Bhuiyan MI, Wahed MA, Yunus M, Islam MS, Breiman RF, Henderson A, Keswick BH, Luby SP (2009) Flocculant-disinfectant point-of-use water treatment for reducing arsenic exposure in rural Bangladesh. Int J Environ Health Res 19(1):17–29. https://doi.org/10.1080/09603120802272219
Obafunmi T, Ocheme J, Gajere B (2020) Oligodynamic effect of precious metals on skin bacteria. Fudma J Sci 4(3):601–608. https://doi.org/10.33003/fjs-2020-0403-334
Okoh EO, Miner CA, Envuladu EA, Mohammed A, Ugochi J (2020) Effect of household water treatment on microbiological quality of drinking water in rural communities of Plateau State, Nigeria: a comparative study of two treatment modalities
Paliwal I (2021) Detection of Trichomonas vaginalis , Giardia and Cryptosporidium spp. in remote indigenous communities in Canada using a point-of-care device
Parham S, Wicaksono DHB, Bagherbaigi S, Lee SL, Nur H (2016) Antimicrobial treatment of different metal oxide nanoparticles: a critical review. J Chin Chem Soc 63(4):385–393. https://doi.org/10.1002/jccs.201500446
Peterson KM, Diedrich DE, Lavigne DJ (2008) Strategies for combating waterborne diarrheal diseases in the developing world, 39.
Praveena SM, Aris AZ (2015) Application of low-cost materials coated with silver nanoparticle as water filter in Escherichia coli removal. Water Qual Expo Health 7(4):617–625. https://doi.org/10.1007/s12403-015-0167-5
Quang DV, Sarawade PB, Hilonga A, Kim J-K, Shim YH, Shao GN, Kim HT (2012) Synthesis of silver nanoparticles within the pores of functionalized-free silica beads: the effect of pore size and porous structure. Mater Lett 68:350–353. https://doi.org/10.1016/j.matlet.2011.10.073
Raghunath A, Perumal E (2017) Metal oxide nanoparticles as antimicrobial agents: a promise for the future. Int J Antimicrob Agents 49(2):137–152. https://doi.org/10.1016/j.ijantimicag.2016.11.011
Rayner J, Luo X, Schubert J, Lennon P, Jellison K, Lantagne D (2017) The effects of input materials on ceramic water filter efficacy for household drinking water treatment. Water Supply 17(3):859–869. https://doi.org/10.2166/ws.2016.176
Reece SM, Sinha A, Grieshop AP (2017) Primary and photochemically aged aerosol emissions from biomass cookstoves: chemical and physical characterization. Environ Sci Technol 51(16):9379–9390.
Rehman AU, Nazir S, Irshad R, Tahir K, ur Rehman K, Islam RU, Wahab Z (2021) Toxicity of heavy metals in plants and animals and their uptake by magnetic iron oxide nanoparticles. J Mol Liq 321:114455
Sawai J, Himizu K, Yamamoto O (2005) Kinetics of bacterial death by heated dolomite powder slurry. Soil Biol Biochem 37(8):1484–1489. https://doi.org/10.1016/j.soilbio.2005.01.011
Shailemo DHP, Kwaambwa HM, Kandawa-Schulz M, Msagati TAM (2016) Antibacterial activity of Moringa ovalifolia and Moringa oleifera methanol, N-hexane and water seeds and bark extracts against pathogens that are implicated in water borne diseases. Green Sustain Chem 06(02):71–77. https://doi.org/10.4236/gsc.2016.62006
Shao GN, Engole M, Imran SM, Jeon SJ, Kim HT (2015) Sol–gel synthesis of photoactive kaolinite-titania: effect of the preparation method and their photocatalytic properties. Appl Surf Sci 331:98–107. https://doi.org/10.1016/j.apsusc.2014.12.199
Shao GN, Imran SM, Jeon SJ, Engole M, Abbas N, Salman Haider M, Kang SJ, Kim HT (2014) Sol–gel synthesis of photoactive zirconia–titania from metal salts and investigation of their photocatalytic properties in the photodegradation of methylene blue. Powder Technol 258:99–109. https://doi.org/10.1016/j.powtec.2014.03.024
Sobsey MD, Brown J (2012) Boiling as household water treatment in Cambodia: a longitudinal study of boiling practice and microbiological effectiveness. Am J Trop Med Hyg 87(3):394–398. https://doi.org/10.4269/ajtmh.2012.11-0715
Syafrudin M, Kristanti RA, Yuniarto A, Hadibarata T, Rhee J, Al-onazi WA, Algarni TS, Almarri AH, Al-Mohaimeed AM (2021) Pesticides in drinking water—a review. Int J Environ Res Public Health 18(2):468. https://doi.org/10.3390/ijerph18020468
Thill A, Zeyons O, Spalla O, Chauvat F, Rose J, Auffan M, Flank AM (2006) Cytotoxicity of CeO 2 nanoparticles for Escherichia coli . physico-chemical insight of the cytotoxicity mechanism. Environ Sci Technol 40(19):6151–6156. https://doi.org/10.1021/es060999b
Tsao NH, Malatesta KA, Anuku NE, Soboyejo WO (2015) Virus filtration in porous iron (III) oxide doped ceramic water filters. Adv Mater Res 1132:284–294. https://doi.org/10.4028/www.scientific.net/AMR.1132.284
Ubomba-Jaswa E, Navntoft C, Polo-López MI, Fernandez-Ibáñez P, McGuigan KG (2009) Solar disinfection of drinking water (SODIS): an investigation of the effect of UV-A dose on inactivation efficiency. Photochem Photobiol Sci 8(5):587. https://doi.org/10.1039/b816593a
UN (2019) The United Nations world water development report 2019: leaving no one behind
Vargas-Reus MA, Memarzadeh K, Huang J, Ren GG, Allaker RP (2012) Antimicrobial activity of nanoparticulate metal oxides against peri-implantitis pathogens. Int J Antimicrob Agents 40(2):135–139. https://doi.org/10.1016/j.ijantimicag.2012.04.012
Vega-Jiménez AL, Vázquez-Olmos AR, Acosta-Gío E, Álvarez-Pérez MA (2019) In vitro antimicrobial activity evaluation of metal oxide nanoparticles. Nanoemulsions Prop Fabr Appl 78812(2):1–18
Verma S, Daverey A, Sharma A (2017) Slow sand filtration for water and wastewater treatment—a review. Environ Technol Rev 6(1):47–58
Waddington H, Snilstveit B (2009) Effectiveness and sustainability of water, sanitation, and hygiene interventions in combating diarrhoea. J Dev Eff 1(3):295–335. https://doi.org/10.1080/19439340903141175
Wang Z, Lee Y-H, Wu B, Horst A, Kang Y, Tang YJ, Chen D-R (2010) Anti-microbial activities of aerosolized transition metal oxide nanoparticles. Chemosphere 80(5):525–529. https://doi.org/10.1016/j.chemosphere.2010.04.047
Wen X, Chen F, Lin Y, Zhu H, Yuan F, Kuang D et al (2020) Microbial indicators and their use for monitoring drinking water quality—a review. Sustainability 12(6):2249
Weststrate J, Dijkstra G, Eshuis J, Gianoli A, Rusca M (2019) The sustainable development goal on water and sanitation: learning from the millennium development goals. Soc Indic Res 143:795–810
World Health Organization (2006) Guidelines for drinking-water quality: First addendum to the third edition, volume 1: recommendations
World Health Organization (2022) Guidelines for drinking‑water quality: Fourth edition incorporating the first and second addenda (4th ed + 1st add + 2nd add). World Health Organization. https://apps.who.int/iris/handle/10665/352532
World Health Organization (2020) State of the world’s sanitation: an urgent call to transform sanitation for better health, environments, economies and societies
Yekta R, Vahid-Dastjerdi L, Norouzbeigi S, Mortazavian AM (2021) Food products as potential carriers of SARS-CoV-2. Food Control 123:107754
Download references
Acknowledgements
We acknowledge Mkwawa University College of Education (MUCE) for material support
Author information
Authors and affiliations.
Department of Chemistry, Mkwawa University College of Education, University of Dar es Salaam, P.O. Box 2513, Iringa, Tanzania
Godfrey Michael Shayo, Elianaso Elimbinzi, Godlisten N. Shao & Christina Fabian
You can also search for this author in PubMed Google Scholar
Contributions
GMS participated in designing, writing, and submitting the manuscript. GNS originated the water purification system idea and conducted research relating to chemical contaminants in water and final approval of the version to be submitted. CFP involved in organization of the manuscript, editing of the manuscript, and revision of the manuscript critically for important intellectual content. EE was a major contributor to the manuscript's writing and interpretation of the relevant literature. All authors have read and approved the manuscript.
Corresponding author
Correspondence to Godfrey Michael Shayo .
Ethics declarations
Ethics approval and consent to participate, consent for publications, competing interests.
The authors declare that they have no competing interests.
Additional information
Publisher's note.
Springer Nature remains neutral with regard to jurisdictional claims in published maps and institutional affiliations.
Rights and permissions
Open Access This article is licensed under a Creative Commons Attribution 4.0 International License, which permits use, sharing, adaptation, distribution and reproduction in any medium or format, as long as you give appropriate credit to the original author(s) and the source, provide a link to the Creative Commons licence, and indicate if changes were made. The images or other third party material in this article are included in the article's Creative Commons licence, unless indicated otherwise in a credit line to the material. If material is not included in the article's Creative Commons licence and your intended use is not permitted by statutory regulation or exceeds the permitted use, you will need to obtain permission directly from the copyright holder. To view a copy of this licence, visit http://creativecommons.org/licenses/by/4.0/ .
Reprints and permissions
About this article
Cite this article.
Shayo, G.M., Elimbinzi, E., Shao, G.N. et al. Severity of waterborne diseases in developing countries and the effectiveness of ceramic filters for improving water quality. Bull Natl Res Cent 47 , 113 (2023). https://doi.org/10.1186/s42269-023-01088-9
Download citation
Received : 05 May 2023
Accepted : 17 July 2023
Published : 24 July 2023
DOI : https://doi.org/10.1186/s42269-023-01088-9
Share this article
Anyone you share the following link with will be able to read this content:
Sorry, a shareable link is not currently available for this article.
Provided by the Springer Nature SharedIt content-sharing initiative
- Waterborne diseases
- Developing countries
- Drinking water
- Water contaminants
- Water-filtration strategies
- Water quality
Loading metrics
Open Access
Peer-reviewed
Research Article
Global Distribution of Outbreaks of Water-Associated Infectious Diseases
Affiliations College of Public Health, The Ohio State University, Columbus, Ohio, United States of America, Department of Schistosomiasis, Jiangsu Institute of Parasitic Diseases, Wuxi, People's Republic of China
Affiliation Ohio Agricultural Research and Development Center, Wooster, Ohio, United States of America
Affiliation School of Earth Sciences, The Ohio State University, Columbus, Ohio, United States of America
Affiliation College of Public Health, The Ohio State University, Columbus, Ohio, United States of America
* E-mail: [email protected]
- Kun Yang,
- Jeffrey LeJeune,
- Doug Alsdorf,
- Bo Lu,
- C. K. Shum,
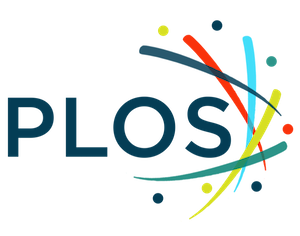
- Published: February 14, 2012
- https://doi.org/10.1371/journal.pntd.0001483
- Reader Comments
Water plays an important role in the transmission of many infectious diseases, which pose a great burden on global public health. However, the global distribution of these water-associated infectious diseases and underlying factors remain largely unexplored.
Methods and Findings
Based on the Global Infectious Disease and Epidemiology Network (GIDEON), a global database including water-associated pathogens and diseases was developed. In this study, reported outbreak events associated with corresponding water-associated infectious diseases from 1991 to 2008 were extracted from the database. The location of each reported outbreak event was identified and geocoded into a GIS database. Also collected in the GIS database included geo-referenced socio-environmental information including population density (2000), annual accumulated temperature, surface water area, and average annual precipitation. Poisson models with Bayesian inference were developed to explore the association between these socio-environmental factors and distribution of the reported outbreak events. Based on model predictions a global relative risk map was generated. A total of 1,428 reported outbreak events were retrieved from the database. The analysis suggested that outbreaks of water-associated diseases are significantly correlated with socio-environmental factors. Population density is a significant risk factor for all categories of reported outbreaks of water-associated diseases; water-related diseases (e.g., vector-borne diseases) are associated with accumulated temperature; water-washed diseases (e.g., conjunctivitis) are inversely related to surface water area; both water-borne and water-related diseases are inversely related to average annual rainfall. Based on the model predictions, “hotspots” of risks for all categories of water-associated diseases were explored.
Conclusions
At the global scale, water-associated infectious diseases are significantly correlated with socio-environmental factors, impacting all regions which are affected disproportionately by different categories of water-associated infectious diseases.
Author Summary
Water is essential for maintaining life on Earth but can also serve as a media for many pathogenic organisms, causing a high disease burden globally. However, how the global distribution of water-associated infectious pathogens/diseases looks like and how such distribution is related to possible social and environmental factors remain largely unknown. In this study, we compiled a database on distribution, biology, and epidemiology of water-associated infectious diseases and collected data on population density, annual accumulated temperature, surface water areas, average annual precipitation, and per capita GDP at the global scale. From the database we extracted reported outbreak events from 1991 to 2008 and developed models to explore the association between the distribution of these outbreaks and social and environmental factors. A total of1,428 outbreaks had been reported and this number only reflected ‘the tip of the iceberg’ of the much bigger problem. We found that the outbreaks of water-associated infectious diseases are significantly correlated with social and environmental factors and that all regions are affected disproportionately by different categories of diseases. Relative risk maps are generated to show ‘hotspots’ of risks for different diseases. Despite certain limitations, the findings may be instrumental for future studies and prioritizing health resources.
Citation: Yang K, LeJeune J, Alsdorf D, Lu B, Shum CK, Liang S (2012) Global Distribution of Outbreaks of Water-Associated Infectious Diseases. PLoS Negl Trop Dis 6(2): e1483. https://doi.org/10.1371/journal.pntd.0001483
Editor: Simon Brooker, London School of Hygiene & Tropical Medicine, United Kingdom
Received: April 13, 2011; Accepted: November 30, 2011; Published: February 14, 2012
Copyright: © 2012 Yang et al. This is an open-access article distributed under the terms of the Creative Commons Attribution License, which permits unrestricted use, distribution, and reproduction in any medium, provided the original author and source are credited.
Funding: This work is supported in part by NIH/NIAID (grant no. R01AI068854) and by the pilot research program by the Climate, Water, Carbon program and Public Health Preparedness of Infectious Diseases (PHPID) of the Ohio State University. SL is thankful for the support from International Gateway Research Grant sponsored by Office of International Affairs (OIA) of the Ohio State University. The funders had no role in study design, data collection and analysis, decision to publish, or preparation of the manuscript.
Competing interests: The authors have declared that no competing interests exist.
Introduction
Although substantial advances in biomedical sciences and public health measures have facilitated control of many infectious diseases in the past century, the world has witnessed an increasing incidence and geographical expansion of emerging and re-emerging infectious diseases [1] , which, together with some other old ones, remain among the leading causes of deaths and disability worldwide [2] , [3] . The global environmental, ecological, and socio-economic changes have a significant impact on the distribution, emergence and re-emergence of infectious diseases and are expected to continue to influence such trend [1] , [4] , [5] , [6] , [7] , [8] , [9] . Some recent studies at both global and regional scales have suggested that climatic factors, human movement, and agricultural practices are important factors underlying the distribution, emergence, and re-emergence of infectious diseases [1] , [6] , [10] .
Water is essential for maintaining life on Earth. Meanwhile, water can also serve as a media for hazardous substances and pathogenic organisms, posing substantial health threats to humans through a variety of pathways. During the past few decades, human development, population growth, extreme weather events, natural calamities, and climate change have exerted many diverse pressures on both the quality and quantity of water resources which may in turn impact conditions fostering water-associated diseases. Worldwide, water-associated infectious diseases are a major cause of morbidity and mortality [11] , [12] , [13] . A conservative estimate indicated that 4.0% of global deaths and 5.7% of the global disease burden (in DALYs) were attributable to a small subset of water, sanitation, and hygiene (WSH) related infectious diseases including diarrheal diseases, schistosomiasis, trachoma, ascariasis, trichuriasis, and hookworm infections [11] , [14] , [15] . Although unknown, the actual disease burden attributable to water-associated pathogens is expected to be much higher. A total of 1415 species of microorganisms have been reported to be pathogenic, among which approximately 348 are water-associated, causing 115 infectious diseases [5] .Yet, their distribution and associated factors at the global scale remain largely unexplored.
Although the linkage between the hydrological cycle and infectious diseases has long been recognized, the underlying mechanisms shaping this relationship at global and regional scales are rarely characterized. Recent developments in hydrology and geo-spatial technology, and increasing availability of spatial socio-environmental information provide an opportunity to explore this issue. Geospatial techniques (e.g. Geographic Information System, or GIS, and spatial analytical techniques) offer a means for developing and organizing spatially explicit information. For example, the availability of information on terrestrial surface water area from the Global Lakes and Wetland Database [16] , could allow the exploration of the possible relationship between the availability of terrestrial surface water and distribution of water-associated diseases at the global scale.
In this study, a comprehensive database has been developed for global water-associated infectious pathogens and diseases and socio-environmental information which have been integrated into a GIS database. The overall goal of our study is to explore the possible relationship between global distribution of water-associated infectious diseases and socio-environmental factors. In this study reported outbreaks of water-associated diseases were chosen as the study subject as they were available in the developed database and provided semiquantitative information (e.g. yes or no, and frequency of outbreaks). Our specific aims in this study were to describe the global distribution of reported outbreaks caused by water-associated infectious diseases from 1991 to 2008, to explore potential risk factors associated with spatio-temporal distributions of these outbreaks, and to develop a global risk map for these diseases.
1. Disease database development
Primary source of information on water-associated pathogens and infectious diseases for the database developed in present study was based on the Global Infectious Disease and Epidemiology Network (GIDEON), a subscription- and web-based comprehensive global infectious diseases database which provides extensive geographical and epidemiological information including outbreaks for 337 recognized infectious diseases in 231 countries and regions. Data in GIDEON are collated through a system of computer macros and dedicated source lists developed over the past 15 years. A monthly search of Medline is conducted against a list of GIDEON key words (similar to Mesh terms in PubMed), and titles/abstracts of interest are reviewed. In addition, all standard publications of WHO and CDC are scanned for relevance before they are collated and entered into GIDEON. The GIDEON infectious diseases database provides a chronological listing of all reported outbreaks of infectious diseases, which are listed by year and country, with specific location information available for the majority of reported outbreaks. For those without specific location information, original publications or reports were searched to extract the information. To assess GIDEON's completeness on the reported outbreaks, a systematic search based on PubMed, ISI Web of Knowledge, WHO and CDC reports was conducted on reported outbreaks (1991–2008) for 10 randomly chosen water-associated diseases. Search terms included names of specific pathogen(s)/disease(s) and country/region, “outbreak”, “epidemic”, and “epidemics”, respectively. Chi-square test was performed to compare results from the independent search vs. that from GIDEON – our results were largely in agreement with that from GIDEON ( X 2 = 591.2, P <0.001). Based on the database developed, water-associated diseases and their corresponding causal agents were systematically reviewed, together with extensive literature review for relevant environmental, biological, and epidemiologic characteristics. For each disease, the following information was included in the database we developed.
- Taxonomic group of causative agents. Five general groups were included - bacteria (including rickettsia), virus (including prions), fungi, protozoa, and helminthes (including cestodes, nematodes, trematodes and acanthocephalans).
- Water mediation of the disease transmission. Following a general framework on the classification of water-associated infectious disease [17] , each disease was classified into one of the following five categories: water-borne, water-based, water-related, water-washed, and water-dispersed. Water-borne diseases, such as typhoid and cholera, are typically caused by enteric microorganisms, which enter water sources through fecal contamination and cause infections in humans through ingestion of contaminated water. To account for water-borne pathogens (e.g. Cryptosporidium, Giardia ) whose transmission can be through accidental ingestion of, or exposure to, contaminated water in recreational settings (for example), we identified outbreaks caused by this transmission pathway and included them in “water-carried diseases”, a sub-group of water-borne diseases by following Steiner et al. [21] ; water-based diseases commonly refer to diseases caused by infections of worms which must spend parts of their life cycles in the aquatic environment, such as schistosomiasis; water-related diseases, such as malaria and trypanosomiasis, need water for breeding of insect vectors to fulfill the transmission cycle; water-washed diseases are those whose transmission is due to poor personal and/or domestic hygiene as a result of lack of appropriate water; and finally, water-dispersed diseases are caused by infections of agents which proliferate in fresh water and enter the human body through the respiratory tract, such as Legionella .
- Transmission routes. Based on the process and nature of transmission, each disease was assigned to one of the four primary transmission groups following the framework by Eisenberg et al. [4] : directly transmitted, vector-borne , environmentally-mediated, and zoonotic. The directly transmitted diseases are those primarily caused by pathogens transmitted via person-to-person contact, where “contact” between humans is the principle mode of transmission, either through intimate proximity (e.g. droplet spray) or bodily fluid exchange. In this group, humans are the only host and the environment typically does not serve as reservoir for the pathogens. Vector-borne diseases are caused by pathogens which are carried by vectors (e.g. mosquitoes) and transmitted to humans through biting. For environmentally-mediated diseases, the environment (e.g., food, water and soil) plays a significant role in a pathogen's life cycle and transmission occurs between humans and the environment directly or indirectly. The zoonotic transmission diseases are diseases that are naturally transmitted between vertebrates and humans. For diseases which may have more than one transmission route, their primary transmission route was used in the database.
- Outbreak events and emergence/re-emergence of water-associated infectious diseases. In the database an outbreak was defined as an increase in cases of disease above what was normally expected in that population in that area and a reported outbreak referred to an outbreak that was reported. Reported outbreaks of water-associated diseases between 1991 and 2008 were extracted from the database. For each outbreak, information including the causal agent, time, and location of the outbreak was extracted from the database. Most of the reported outbreaks had location information (e.g. villages, counties, or cities where the outbreaks took place). For those without location documented in the database (GIDEON), original publications or reports were checked to retrieve outbreak locations. For reported outbreaks, the spatial scales of reports obtained were on the order of municipality/county or smaller. Based on centroid points of geographical areas (e.g., village, county, or city) where outbreaks were reported, the outbreaks were positioned in Google Earth® and corresponding longitudinal and latitudinal information were extrapolated to ArcGIS (9.2) for grid-based (one degree) analyses described below. For causal agents of the outbreaks, they were also characterized as either emerging/re-emerging or non-emerging pathogen(s) by following the criteria previously defined [5] .
2. Socio-environmental database
The database included the following information - grid-based global human population density (per km 2 ) based on the 2000 global population dataset, which was developed by Socioeconomic Data and Applications Center (SEDAC) of Columbia University between 2003 and 2005, providing globally consistent and spatially explicit human population information ( http://sedac.ciesin.columbia.edu/gpw/ ); global average accumulated temperature (degree days, with a spatial resolution of 0.5 degree) for the period between 1961 and1990 from United Nation Environmental Protection( http://www.unep.org/ ), which was based on the degree that the temperature rose above zero degree and the number of days in the period during which this excess was maintained [18] ; surface area (km 2 ) of water bodies including large lakes , rivers, and wetland, collected from the global lakes and wetlands database ( http://www.worldwildlife.org/ ); the average rainfall (mm) per year for the period between 1961 and1990 from FAO ( http://www.fao.org ); and per capita Gross Domestic Product (GDP) which was based on each a country's GDP divided by the total number of people in the country ( http://sedac.ciesin.columbia.edu/ddc/baseline/ ). The scale of all information collected was converted to one-degree grid in the GIS database.
3. Statistical analyses
Controlling for reporting bias..
Primary source of information on reported outbreak events was from GIDEON, which is based on peer-reviewed publications and reports of governmental and international agencies (e.g. CDC and WHO), and considered comprehensive. However, as long being recognized, underreporting of infectious disease outbreaks widely exists, depending on a number of factors such as a country's socio-economic status and investment of research resources. For instance, outbreak events are more likely to be reported in developed countries than in developing countries due to greater availability of resources in the former, which may cause reporting bias [19] . To account for the potential bias, reporting efforts for each country were quantified by estimating published articles specifically related to each country from 1991 to 2008 following Jones et al.'s approach [1] . Using PubMed, “infectious disease” and “country name” were used as keywords in the search of publications to approximate the reporting efforts for each country. Figure 1 shows the global trend in the number of publications on infectious diseases and reported outbreaks of water-associated infectious diseases from 1991 to 2008, suggesting a strong correlation between the outbreak events and publications. In the analysis, the number of publications for each country (e.g. grid cells within each country having the same number) was set as an offset variable to control for reporting bias [1] .
- PPT PowerPoint slide
- PNG larger image
- TIFF original image
Shown are the global trends in the number of publications on infectious disease and reported outbreaks of water-associated infectious diseases from 1991 to 2008 (Pearson correlation - 0.935, P<0.001 ).
https://doi.org/10.1371/journal.pntd.0001483.g001
Descriptive/exploratory analysis.
Basic characteristics (e.g. taxonomy and transmission routes) of causal agents associated with the outbreak events were summarized. Temporal trend of outbreak events in relation to the number of publications was tested. Exploratory analysis was conducted using a multivariable logistic regression to control for co-variability between independent variables, with the presence/absence of outbreak events as the dependent variable and all factors including the reporting effort by each country as independent variables. Correlation analyses were conducted for socio-economic variables including population density, global accumulated temperature, per capita GDP. The variables of statistical significance in the correlation analyses were included in the Bayesian analysis described below. All analyses were performed using SPSS (SPSS Inc., USA).
Bayesian analysis.
Risk predictions and mapping.
A total of 1,428 outbreak events had been reported from 1991 to 2008. Outbreaks occurred all over the world and the clusters of reported outbreaks tended to be in west Europe, central Africa, north India and Southeast Asia ( Figure 2 ). Among the reported outbreak events, 70.9% (1,012) were associated with water-borne diseases including 32.9% (471) water-carried, 12.2% (174) water-related, 6.8% (97) water-washed, 2.9% (41) water-based, and 7.3% (104) water-dispersed. 46.7% (667) of the outbreak events were associated with emerging or reemerging pathogens, which appeared in humans for the first time or had occurred previously but were increasing in incidence or expanding into areas where they had not previously been reported [5] . It is found that 49.6% (709) of the outbreak events was caused by bacteria, 39.3% (561) by viruses, and 11.1% (158) by parasites. 6.5% (93) of the outbreak events was caused by agents that could be transmitted by direct contact, 1.1% (16) transmitted through vectors, 63.5% (907) through environmental transmission, and 28.9% (412) by zoonotic routes.
https://doi.org/10.1371/journal.pntd.0001483.g002
The reported outbreak events had shown a significant increase since 1991, which had been accompanied by a significant increase in the number of published articles ( Figure 1 , Pearson correlation - 0.935, P<0.001 ). We used a generalized linear model to test the temporal trend in the outbreak events and found it insignificant (t = 0.046, P = 0.940 ) after controlling for the publication efforts. The number of published articles was therefore used as a covariate in the subsequent statistical analyses.
Table 1 summarizes analyses of the Poisson models without and with spatially structured random effects using Bayesian inference for the five categories of water-associated diseases. The DIC values of the Poisson model with spatial random effects are smaller than that without spatial structure, suggesting that the spatial models provided a better fit to the data. The Poisson models with spatial structure were therefore used for risk factor analysis and mapping.
https://doi.org/10.1371/journal.pntd.0001483.t001
The population density was shown to be a significant risk factor for reported outbreaks of all categories of water-associated infectious diseases and the probability of outbreak occurrence increased with the population density. The accumulated temperature was a significant risk factor for water-related diseases only. The analysis suggested that occurrence of water-washed diseases had significantly inverse relationship with surface water areas. Such inverse relationship was also observed between the average annual rainfall and water-borne diseases (including water-carried) and water-related diseases.
Figure 3 (A–F) shows the risk distribution based on the model predictions with the blue indicating lower risk while the red representing higher risk. The model predictions suggested that west Europe, central Africa, north India were at the higher risk for water-borne diseases (e.g. Escherichia coli diarrhea), and notably, that the higher risk for water-borne diseases in west Europe was primarily driven by water-carried diseases (e.g. cryptosporidiosis). West Europe, North Africa, and Latin America tended to be at higher risk to water-washed diseases (e.g. viral conjunctivitis). Risks associated with water-based diseases (e.g. schistosomiasis) were higher in east Brazil, northwest Africa, central Africa, and southeast of China. High risk areas for water-related diseases (e.g. malaria and dengue fever) were clustered in central Africa in particular Ethiopia and Kenya, and north India. For water-dispersed diseases (e.g. Legionellosis), west Europe seemed to be at higher risk.
Shown are relative risk distributions for different categories of water-associated infectious diseases – water-borne (A), water-carried (B), water-based (C), water-related (D), water-washed (E), and water-dispersed (F). Relative risk estimate was based on the best fit Bayesian model integrating reported outbreaks, random and spatial effects.
https://doi.org/10.1371/journal.pntd.0001483.g003
In the past decade there has been an increasing interest in understanding factors underlying the distribution of infectious pathogens, emerging and re-emerging infectious diseases. Some recent research efforts have been in attempt to determine large-scale ecological factors associated with diversity richness and distribution of infectious and parasitic pathogens [6] , socio-environmental determinants of emerging infectious disease [1] , and to explore the impact of global environmental change on distribution and spread of infectious diseases [23] , [24] . These studies have offered valuable insights into understanding socio-environmental processes and factors underlying the distribution of infectious diseases. In this study, we focused our attention on water-associated infectious diseases and attempted to explore whether these diseases follow similar patterns observed in other studies [1] , [6] , and whether the distribution and occurrence of these diseases were related to terrestrial water dynamics (e.g. precipitation and land-surface water) together with other socio-environmental factors. The transmission of many infectious diseases is closely linked to water and the water-infectious pathogen interactions exhibit a complicated relationship depending on the transmission characteristics of the pathogens and water's roles in the transmission. The study showed that water-associated infectious diseases and outbreaks were broadly distributed throughout the world but the distribution of specific agents/diseases varied greatly from region to region. The majority of reported outbreaks events were associated with water-borne pathogen including those water-carried. Water-borne diseases have a much broader distribution than other water-associated diseases, suggesting a broader impact of waterborne pathogens in particular those related to fecal-oral route and water, sanitation, and hygiene. In addition to water, other environmental factors have also been recognized to play a significant role in the distribution, transmission, and outbreaks of these water-associated diseases [25] , [26] , [27] .
It should be noted that, though, the outbreaks reported here only reflected “the tip of the iceberg” of the much larger problem. A complete count of outbreaks attributable to water-associated pathogens is impossible as underreporting is a universal problem, and reporting efforts and effectiveness may vary from country to country, and pathogens to pathogens, depending on many factors particularly availability of research and surveillance resources, and epidemiological characteristics of causal agents. In developing countries, outbreaks of many vector-borne infectious diseases such as dengue and malaria [28] , [29] and gastrointestinal infections [30] were grossly underreported, partly due to their endemic characteristics. Even in the US, reporting completeness of notifiable infectious diseases varied from 9% to 99%, and was strongly associated with diseases being reported [31] . In general, water-borne pathogens usually exhibit acute manifestations and are more likely to be reported [32] . In contrast, other diseases such as water-based schistosomiasis, a disease of chronic infections and atypical symptoms, are more likely to be underreported. In this study, the primary source of outbreak information was from GIDEON, which is the most comprehensive database on infectious diseases and offers detailed information on epidemiology including distributions and outbreaks of infectious diseases for more than 205 countries and regions, as well as clinical manifestations and treatment associated with each disease [23] , [33] . As expected, GIDEON does not include all outbreak information due to underreporting of outbreak events, but we believe that information from GIDEON is representative and provides an overview of available and recognized outbreak data, as argued by some other studies [23] , [33] .
The distribution of water-associated diseases, like many other infectious diseases, is highly heterogeneous. The spatial structure associated with the distribution of the outbreaks may be important in understanding underlying risk factors. To explore possible associations between socio-environmental factors and the outbreaks at the global scale, two Poisson models (without and with spatial structures) were developed. Among the two models explored, the one incorporating spatial effects provided a better fit to the data. Our findings suggested that the importance of these socio-environmental variables was dependent on the category of water-associated diseases. Human population density was a common significant risk factor for the outbreaks caused by all categories of water-associated diseases, in concurrence with the previous study suggesting that human population was an important predictor of emerging infectious diseases event at the global scale [1] . The accumulated temperature was a significant factor associated with water-related diseases, which was in agreement with many other studies [34] , [35] , [36] , [37] . The transmission of diseases in this category typically involves vectors (e.g. mosquitoes) which require certain energy level (e.g. accumulated temperature) allowing completion of development of vectors and pathogens [10] , [38] , [39] . In this study, terrestrial surface water area (at each grid-region) was found to be inversely proportional to the outbreak events associated with water-washed diseases such as trachoma. The primary determinant of water-washed diseases is poor personal and/or domestic hygiene typically due to insufficient sanitary water for hygienic purpose, and this has been reported in many site-specific studies [40] , [41] , [42] . Our result from a large-scale correlation study supported these points of the previous studies, suggesting that regional water availability may be indicative of local water availability which is closely linked to personal and domestic hygiene. Our analysis indicated a negative relationship between average annual rainfall and water-related diseases, in contrast with some previous studies showing that some outbreaks of water-related diseases are positively associated with heavy rainfall events [8] , [43] , [44] , [45] . This can be partly explained by issues related to scale and timing effects – the majority of studies reporting positive relationship between precipitation and waterborne illness was conducted at local scale and typically time-lag effects were considered. Indeed, the rainfall and water-related diseases exhibit complex relationships as shown in previous studies, and many rainfall-driven transmission and outbreaks were dependent on local circumstances. In addition to rainfall, multiple and covarying drivers have also been proposed for seasonal pattern of transmission and outbreaks of many water-associated diseases, including temperature, host demographic and biological characteristics [46] , [47] , [48] . However, due to lack of global information on seasonal patterns of outbreaks and the driving factors, temporal heterogeneity of outbreaks events, such as seasonality discussed here, was not included in the present study.
Using the best-fitted models we predicted global distributions of relative risks associated with each category of water-related infectious diseases, as shown in Figure 3 . Surprisingly, the risk maps show that west Europe and central Africa were all at relatively higher risk for water-borne diseases. A closer look at pathogens associated with the reported outbreaks indicated different dominant species in the two regions – in Africa reports of water-borne outbreaks were primarily associated with Vibrio cholerae , whereas in west Europe giardia, cryptosporidium were common in the water-borne outbreaks, with the latter being particularly related to accidental ingestions of contaminated water (e.g. in recreational settings) and, to some extent, mixed with infections of food-borne sources [49] , [50] , [51] .
Some limitations of the current study are recognized. Although possible reporting bias was adjusted for using publications for each country, the analysis may have missed countries/regions with outbreaks but no publications and/or reports. Second, only a few socio-environmental factors were considered in the present study and it is likely that some other factors might be associated with the outbreaks. In addition, significant prediction uncertainties were noted throughout the outbreak countries and regions, this was partly due to the temporal correlation of the outbreak events which was not considered in the analysis. The addition of such information (e.g. temporal trend of outbreaks in places where repeated outbreaks occurred) to the model may improve model prediction. In spite of these, we think that overall patterns of distribution and associated risk factors presented here are informative and offer insights into global distribution and risk factors associated with water-associated diseases, although further studies on other possible risk factors and modeling approaches to improving prediction are still needed.
In conclusion, our study, to our knowledge, is the first to describe global distribution of outbreaks caused by water-associated infectious diseases and explore possible risk factors underlying the distribution of these outbreaks at the global scale. The risk maps may offer insights for future studies and for prioritizing health resources.
Author Contributions
Conceived and designed the experiments: SL JL DA. Performed the experiments: KY SL. Analyzed the data: KY SL BL CKS. Wrote the paper: KY SL JL DA BL CKS.
- View Article
- Google Scholar
- 17. Cotruvo J, Dufour A, Rees G, Bartram J, Carr R, et al. (2004) Waterborne zoonoses: identification, causes, and control. IWA Publishing.
- DOI: 10.9734/ijtdh/2016/21895
- Corpus ID: 28760417
Water and Waterborne Diseases: A Review
- N. Forstinus , N. Ikechukwu , +1 author A. Christiana
- Published 10 January 2016
- Environmental Science, Medicine
- International Journal of Tropical Disease & Health
Tables from this paper
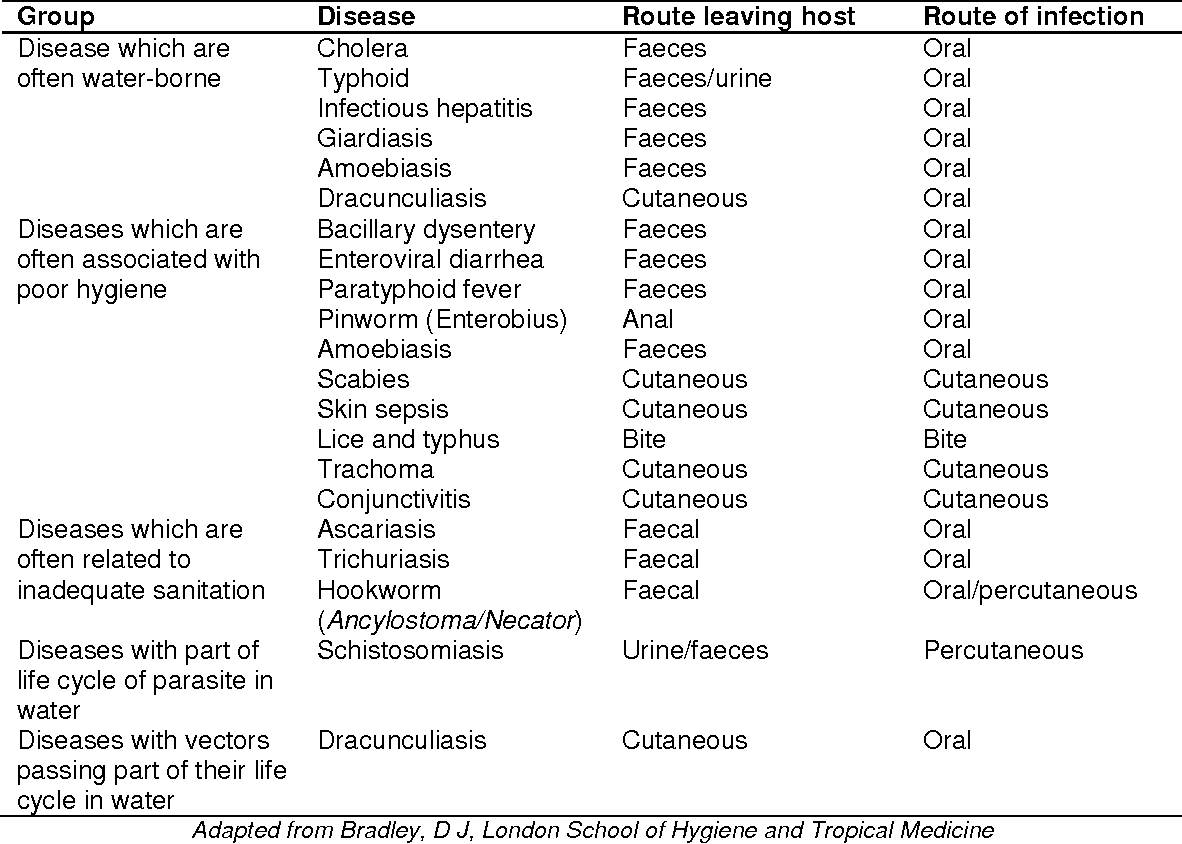
75 Citations
Health hazards of water contamination: an updated review among the covid-19 pandemic, prevalence of waterborne diseases in bade, nguru and machina local government areas of yobe state-nigeria.
- Highly Influenced
Microbial Indicators and Their Use for Monitoring Drinking Water Quality—A Review
Water borne diseases control in livestock through water troughs management system, monitoring of microbial contamination of groundwater in the upper choluteca river basin, honduras, a study on water sources and water borne diseases in kona community, jalingo lga taraba state, water sanitation problem in pakistan: a review on disease prevalence, strategies for treatment and prevention, improved quantitative microbial risk assessment (qmra) for drinking water sources in developing countries, assessment of drinking water quality in umoja innercore estate, nairobi, water for domestic animal drinking based on the categorization of species in an urban brazilian slum, 87 references, review on prevalence of waterborne diseases in nigeria, wastewater treatment plants as a source of microbial pathogens in receiving watersheds, assessment of groundwater quality in a typical rural settlement in southwest nigeria, enterococci in river ganga surface waters: propensity of species distribution, dissemination of antimicrobial-resistance and virulence-markers among species along landscape, occurrence of escherichia coli o 157 in a river used for fresh produce irrigation in nigeria, bacteriological quality of public water sources in shuni, tambuwal and sokoto towns in north-western nigeria., escherichia coli: the best biological drinking water indicator for public health protection, a simple field test for the detection of faecal pollution in drinking water., water quality assessment: surface water sources used for drinking and irrigation in zaria, nigeria are a public health hazard, municipal wastewater effluents as a source of listerial pathogens in the aquatic milieu of the eastern cape province of south africa: a concern of public health importance, related papers.
Showing 1 through 3 of 0 Related Papers
Water-Borne Diseases
- Living reference work entry
- First Online: 29 April 2019
- Cite this living reference work entry
- Kinga Szálkai 4
791 Accesses
2 Citations
Introduction
In the field of health security, adequate, safe, and accessible water supplies and satisfactory sanitation are of key importance. “Pure water is the world’s first and foremost medicine,” says a Slovakian proverb, and the sentence has a similarly important meaning in its reversed form: contaminated water as the transmitter of water-borne illnesses is one of the leading causes of death globally.
Between 1980 and 2015, the average annual number of deaths due to water unsafe for human health amounted to 780,000 – dwarfing the number of deaths as a consequence of natural disasters (63,000) and in conflicts (75,000) (Ligtvoet et al. 2018 , p. 38). According to the estimations of the World Health Organization (WHO, see chapter “World Health Organization (WHO)” ), the overall number of people who die in diarrheal diseases per year totals 1.5 million, among whom 502,000 people die of diseases related to contaminated drinking water. Cases related to the lack of sanitation and hand...
This is a preview of subscription content, log in via an institution to check access.
Access this chapter
Institutional subscriptions
Bartram, J., & Hunter, P. (2015). Bradley classification of disease transmission routes for water-related hazards. In J. Bartram et al. (Eds.), Routledge handbook of water and health (pp. 20–37). London/New York: Routledge.
Chapter Google Scholar
Batterman, S., et al. (2009). Sustainable control of water-related infectious diseases: A review and proposal for interdisciplinary health-based systems research. Environmental Health Perspectives , (7), 1023–1032.
Article Google Scholar
Baylis, M. (2017). Potential impact of climate change on emerging vector-borne and other infections in the UK. Environmental Health, 16 (Suppl. 1), 112. 45–51.
Delpla, I., et al. (2009). Impacts of climate change on surface water quality in relation to drinking water production. Environment International, 35 , 1225–1233.
EHP-NIEHS (Environmental Health Perspectives and the National Institute of Environmental Health Sciences). (2010). A human health perspective on climate change: A report outlining the research needs on the human health effects of climate change. The Interagency Working Group on Climate Change and Health. Retrieved 12 May 2018, from https://www.niehs.nih.gov/health/materials/a_human_health_perspective_on_climate_change_full_report_508.pdf
Eisenberg, J. N. S., Bartram, J., & Hunter, P. R. (2001). A public health perspective for establishing water-related guidelines and standards. In L. Fewtrell & J. Bartram (Eds.), Water quality: Guidelines, standards and health (World Health Organization (WHO)) (pp. 229–256). London: IWA Publishing.
Google Scholar
Filho, W. L., et al. (2018). Climate change and health: An analysis of causal relations on the spread of vector-borne diseases in Brazil. Journal of Cleaner Production, 177 , 589–596.
Gray, N. F. (2014). Chapter thirty-six: The implications of global warming and climate change on waterborne diseases. In S. L. Percival et al. (Eds.), Microbiology of waterborne diseases: Microbiological aspects and risks (pp. 653–666). London: Academic/Elsevier.
Hunter, P. R. (2003). Climate change and waterborne and vector-borne disease. Journal of Applied Microbiology, 94 , 37S–46S.
Jamul, J. (2007). Foodborne and waterborne infections. In S. W. Salyer (Ed.), Essential emergency medicine: For the healthcare practitioner (pp. 264–274). Philadelphia: Saunders Elsevier.
Ligtvoet, W., et al. (2018). The geography of future water challenges . The Hague: PBL Netherlands Environmental Assessment Agency.
Perkins, A., & Trimmier, M. (2017). Recreational waterborne illnesses: Recognition, treatment, and prevention. American Family Physician, 9 , 554–560.
Pond, K. (2005). Water recreation and disease plausibility of associated infections: Acute effects, sequelae and mortality (World Health Organization (WHO)). London: IWA Publishing.
Shankar, P., Mishra, J., & Singh, S. (2014). Hepatitis A and E in potable water: A threat to health. In P. P. Singh & V. Sharma (Eds.), Water and health (pp. 29–51). New Delhi: Springer.
Singh, P. P., & Sharma, V. (2014). Prelude. In P. P. Singh & V. Sharma (Eds.), Water and health (pp. vii–viii). New Delhi: Springer.
Webersik, C. (2014). Climate change, water and health: Current impacts and future challenges for human security. In V. I. Grover (Ed.), Impact of climate change on water and health (pp. 54–74). Boca Raton: CRC Press/Taylor & Francis Group.
White, G., Bradley, D., & White, A. (1972). Drawers of water . Chicago: University of Chicago Press.
WHO. (2011). Cause-specific mortality: Regional estimates for 2008 . Geneva: World Health Organization. Retrieved 21 June 2012, from http://www.who.int/healthinfo/global_burden_disease/estimates_regional/en/index.html
WHO. (2015). WHO estimates of the global burden of foodborne diseases . Geneva: World Health Organization. Retrieved 12 May 2018, from http://apps.who.int/iris/bitstream/handle/10665/199350/9789241565165_eng.pdf
WHO. (2018a). Drinking-water. Retrieved 12 May 2018, from http://www.who.int/en/news-room/fact-sheets/detail/drinking-water
WHO. (2018b). Water, sanitation, hygiene: Diseases and risks. Retrieved 12 May 2018, from http://www.who.int/water_sanitation_health/diseases-risks/en/
WHO. (2018c). Water-related diseases: Information sheets. Retrieved 12 May 2018, from http://www.who.int/water_sanitation_health/diseases-risks/en/
WHO. (2018d). Foodborne diseases. Retrieved 12 May 2018, from http://www.who.int/topics/foodborne_diseases/en/
WHO. (2018e). The top 10 causes of death. Retrieved 24 June 2018, from http://www.who.int/en/news-room/fact-sheets/detail/the-top-10-causes-of-death
Download references
Author information
Authors and affiliations.
Eötvös Loránd University, Budapest, Hungary
Kinga Szálkai
You can also search for this author in PubMed Google Scholar
Corresponding author
Correspondence to Kinga Szálkai .
Editor information
Editors and affiliations.
University of Alberta, Alberta, AB, Canada
Scott Romaniuk
University for Peace, San Jose, Costa Rica
Manish Thapa
Nemzetkozi Tanulmanyok Intezet, Rm 503, Corvinus Univ, Inst of Intl Studies, Budapest, Hungary
Péter Marton
Rights and permissions
Reprints and permissions
Copyright information
© 2019 The Author(s), under exclusive license to Springer Nature Switzerland AG
About this entry
Cite this entry.
Szálkai, K. (2019). Water-Borne Diseases. In: Romaniuk, S., Thapa, M., Marton, P. (eds) The Palgrave Encyclopedia of Global Security Studies. Palgrave Macmillan, Cham. https://doi.org/10.1007/978-3-319-74336-3_562-1
Download citation
DOI : https://doi.org/10.1007/978-3-319-74336-3_562-1
Received : 25 June 2018
Accepted : 06 February 2019
Published : 29 April 2019
Publisher Name : Palgrave Macmillan, Cham
Print ISBN : 978-3-319-74336-3
Online ISBN : 978-3-319-74336-3
eBook Packages : Springer Reference Political Science and International Studies Reference Module Humanities and Social Sciences Reference Module Business, Economics and Social Sciences
- Publish with us
Policies and ethics
- Find a journal
- Track your research
- Open access
- Published: 17 May 2022
Prevalence and predictors of water-borne diseases among elderly people in India: evidence from Longitudinal Ageing Study in India, 2017–18
- Pradeep Kumar 1 ,
- Shobhit Srivastava 2 ,
- Adrita Banerjee 3 &
- Snigdha Banerjee 3
BMC Public Health volume 22 , Article number: 993 ( 2022 ) Cite this article
18k Accesses
27 Citations
10 Altmetric
Metrics details
India suffers from a high burden of diarrhoea and other water-borne diseases due to unsafe water, inadequate sanitation and poor hygiene practices among human population. With age the immune system becomes complex and antibody alone does not determine susceptibility to diseases which increases the chances of waterborne disease among elderly population. Therefore the study examines the prevalence and predictors of water-borne diseases among elderly in India.
Data for this study was collected from the Longitudinal Ageing Study in India (LASI), 2017–18. Descriptive statistics along with bivariate analysis was used in the present study to reveal the initial results. Proportion test was applied to check the significance level of prevalence of water borne diseases between urban and rural place of residence. Additionally, binary logistic regression analysis was used to estimate the association between the outcome variable (water borne diseases) and the explanatory variables.
The study finds the prevalence of water borne disease among the elderly is more in the rural (22.5%) areas compared to the urban counterparts (12.2%) due to the use of unimproved water sources. The percentage of population aged 60 years and above with waterborne disease is more in the central Indian states like Chhattisgarh and Madhya Pradesh followed by the North Indian states. Sex of the participate, educational status, work status, BMI, place of residence, type of toilet facility and water source are important determinants of water borne disease among elderly in India.
Elderly people living in the rural areas are more prone to waterborne diseases. The study also finds state wise variation in prevalence of waterborne diseases. The elderly people might not be aware of the hygiene practices which further adhere to the disease risk. Therefore, there is a need to create awareness on basic hygiene among this population for preventing such bacterial diseases.
Peer Review reports
Introduction
The Sustainable Development Goal, 2017 aimed to ensure availability and sustainable management of water and sanitation for all by 2030 [ 1 ]. However globally 780 million people live without access to safe water and approximately 2.5 billion people in the developing world lived without access to adequate sanitation [ 2 , 3 ]. Polluted water and poor sanitation practices expose individuals to health risks. Emerging water-borne pathogens constitute a significant health hazard in both developed and developing nations [ 4 ] as they can spread rapidly and affect large sections of the population. Water-borne diseases are transmitted through contaminated drinking water with pathogen microorganisms such as protozoa, virus, bacteria, and intestinal parasites. According to the projection of Global Burden Disease report, the burden of water borne disease was the second highest reason for mortality in 1990 however, it was lower down in ninth most important reason for mortality in 2020 [ 5 ]. Around 829,000 people are estimated to die each year from diarrheal diseases majorly cholera, dysentery and typhoid fever due to unsafe drinking water and unhygienic sanitation practice [ 6 ]. Further, the WHO (2015) reported that about 6.3 per cent of deaths occur due to unsafe water, inadequate sanitation, and poor hygiene. Adequate, safe, and accessible water supplies as well as satisfactory sanitation are most required to have secure health status [ 7 ]. According to WHO (2015), nearly 4 percent of the global disease burden could be prevented by improving water supply, sanitation, and hygiene [ 8 ].
It is estimated that around 37.7 million Indians are affected by waterborne diseases annually; 1.5 million children are estimated to die of diarrhoea alone and 73 million working days are lost due to waterborne disease each year [ 9 ]. Water-borne diseases pose a high disease burden and significantly impact on country’s economic growth [ 10 ]. These diseases erupt every year during summer and rainy seasons as a result of improper management of water supply especially of drinking water and sanitation [ 11 , 12 ].
Poor urban governance, rapidly growing economies, highly dense population, poor housing and sanitation in slum areas of cities create environments rife for waterborne diseases [ 13 ]. One of the study in slum areas of Mumbai revealed that at least 30 per cent of all morbidity are due to water-related infections [ 14 ]. In rural areas, there are no proper water supply and sewerage systems. In the villages, water contamination can be attributed to infiltration, leaching, and surface run-off through pastures, lacking and leakage of sewerage disposal systems. Studies based on rural India revealed that lacking in knowledge, attitudes and practices (KAP) with regard to water handling, sanitation and defecation practices are common causes of waterborne diseases [ 15 , 16 ]. Water pollution, open defecation and poor hygiene practices are the main hindrances to achieving good health. Therefore, safe and readily available water is essential for public health whether used for drinking, domestic use, food production or recreational purposes. Adequate access to safe water, improving quality of water source, treating and storing household water and encouraging hygiene practices can prevent waterborne diseases. As the global population is increasing rapidly over time, water availability will lower down steadily [ 8 ]. Individuals with low immunity are more susceptible to water-borne diarrheal diseases, especially children andelderly, with the low immune system are most susceptible to pathogen-related water-borne diseases [ 17 ]. According to U.S. Environmental Protection Agency, elderly along with children and pregnant women, were recognized as the sensitive sub-populations for water-borne diseases [ 18 ].
India is currently in the third stage of demographic transition and with 8% of geriatric population India could well be called an ageing nation [ 19 ]. The elderly population of India is expected to increase three fold by 2050 [ 20 ]. Among the elderly, infections are often more severe due to the presence of multiple underlying medical conditions, low immune system, and frequent use of drugs [ 21 ]. People in India mostly are unaware of safe and hygienic practices and this is prevalent across all age groups. This in turn increases the risk of communicable diseases. Thus, the resources and policy attention should be focused on strengthening primary health care systems that address communicable diseases and reduce the underlying risk factors. The rising number of elderly with various health problems creates a pressure on the existing public health system in India. In order to focus on strengthening the health care system to serve the elderly population there is need to study the prevalence of various disease risk among the elderly population. The major objective of the study is to examine the prevalence and predictors of water borne diseases among elderly. The study aims to bridge the research gap as less attention has been paid on the water borne diseases among the elderly population. The results of the study would further help in embarking knowledge, attitude and practices related to water handling, sanitation and defecation practices among the elderly which might reduce to some extent the load of communicable disease risk among elderly.
Materials and methods
Study settings and population.
Cross-sectional data for this study was used from the Longitudinal Ageing Study in India (LASI), nationally representative survey conducted in the year 2017–18 and covered 72,000 elderly age 45 and above across all states and union territories of India [ 22 ].
Study design
Cross-sectional survey.
Sample size calculation and sampling procedure
LASI is a full-scale national survey of scientific investigation of the health, economic, and social determinants and consequences of population aging in India. The main objective of the LASI survey was to study the health status and the social and economic well-being of elderly in India. The survey adopted a multistage stratified area probability cluster sampling design to arrive at the eventual units of observation: elderly age 45 and above and their spouses irrespective of age.
Within each state, LASI Wave 1 adopted three-stage sampling design in rural areas and four-stage sampling design in urban areas. In each state/UTs, the first stage involved selection of Primary Sampling Units (PSUs), that is, sub-districts (Tehsils/Talukas), and the second stage involved the selection of villages in rural areas and wards in urban areas in the selected PSUs. In rural areas, households were selected from selected villages in the third stage. However, sampling in urban areas involved an additional stage. Specifically, in the third stage, one Census Enumeration Block (CEB) was randomly selected in each in urban area. In the fourth stage, households were selected from this CEB.
The present study is conducted on the eligible participant’s age 60 years and above. The total sample size for the present study is 31464 (for rural-20725 and urban-10739) elders aged 60 years and above [ 22 ].
Study variables
Outcome variable.
The outcome variable (water borne diseases) was binary in nature i.e. water borne diseases coded as no and yes. The variable was generated using the question “has any health professional diagnosed you with diarrhoea/gastroenteritis or typhoid or jaundice/hepatitis in last two years [ 23 ].
Explanatory variables
The control variables were selected after doing extensive literature review. The variables selected are as follows:
Age was recoded as 60–69, 70–79 and 80 + years.
Sex was recoded as male and female.
Education was recoded as no education/primary not completed, primary completed, secondary completed and higher and above.
Marital status was recoded as currently married, widowed and others. Others included separated/never married/divorced.
Working status was coded as currently working, retire and never worked.
Body mass index was recoded as underweight, normal and overweight/obese. The participants having a body mass index (BMI) of 25 and above were categorized as obese/overweight whereas participant who had BMI as 18.4 and less were coded as underweight [ 24 ]. BMI is calculated by dividing an individual’s weight (in kilograms) by the square of their height (in metres).
Type of toilet facility was recoded as unimproved and improved [ 25 ]. Improved toilet facility includes pour-flush latrines, ventilated improved pit latrines, and pit latrines with a slab/covered pit. Unimproved toilet facility includes Shared facilities of any type, no facilities (bush or field); flush or pour-flush to elsewhere (that is, not to piped sewer system, septic tank or pit latrine); pit latrines without slab / open pits, bucket systems; hanging toilet or hanging latrine.
Source of drinking water was recoded as unimproved and improved [ 25 ]. Improved source of drinking water includes piped water, public tap/standpipe, tube well or bore well, dug well, spring water and rain water. Unimproved water sources include tanker, cart with small tank, bottled water/pouch water, surface water and other sources of water.
Type of house was recoded as pucca, semi pucca and kutcha.
The monthly per capita expenditure (MPCE) was assessed using household consumption data. Sets of 11 and 29 questions on the expenditures on food and non-food items, respectively, were used to canvas the sample households. Food expenditure was collected based on a reference period of seven days, and non-food expenditure was collected based on reference periods of 30 days and 365 days. Food and non-food expenditures have been standardized to the 30-day reference period. The monthly per capita consumption expenditure (MPCE) is computed and used as the summary measure of consumption. The variable was then divided into five quintiles i.e., from poorest to richest [ 22 ].
Religion was recoded as Hindu, Muslim, Christian and Others.
Caste was recoded as Scheduled Tribe, Scheduled Caste, Other Backward Class, and others. The Scheduled Caste include “untouchables”; a group of the population that is socially segregated and financially/economically by their low status as per Hindu caste hierarchy. The Scheduled Castes (SCs) and Scheduled Tribes (STs) are among the most disadvantaged socio-economic groups in India. The OBC is the group of people who were identified as “educationally, economically and socially backward”. The OBC’s are considered low in the traditional caste hierarchy but are not considered untouchables. The “other” caste category is identified as having higher social status [ 26 ].
Place of residence was recoded as rural and urban area.
Region was recoded as North, Central, East, Northeast, West, and South.
Statistical analysis
Univariate along with bivariate analysis was used in present study to reveal the initial results. Proportion test [ 27 ] was applied to check the significance level of prevalence of water borne diseases between urban and rural place of residence. Additionally, binary logistic regression analysis [ 28 ] was used to estimate the association between the outcome variable (water borne diseases) and other explanatory variables.
The binary logistic regression model is usually put into a more compact form as follows:
The parameter \({\beta }_{0}\) estimates the log odds of water borne diseases for the reference group, while \(\beta\) estimates the maximum likelihood, the differential log odds of water borne diseases associated with a set of predictors X, as compared to the reference group, and \(\epsilon\) represents the residual in the model. The variance inflation factor (VIF) ( Additional file -Table-A 1 ) was used to check for the presence of multicollinearity and the test confirmed that there was no evidence of multicollinearity [ 29 ]. STATA 14 was used for the analysis purpose.
Socio-demographic and economic profile of elderly in India
Table 1 presents the socio-demographic and economic profile of the study participants. A similar proportion of elderly lived in rural and urban areas irrespective of age group. Only three per cent of elderly in rural areas had higher education and this percentage was five times in urban areas. In rural areas, about one-third of elderly were working whereas one-fifth of elderly in urban areas were working. Nearly one-third of older adults in rural and one in every ten older elderly in urban areas were underweight. Only one-third of elderly in rural areas were used improved toilet facility and eight in every 10 elderly in urban areas were used improved toilet facility. In rural areas, three fifth of elderly used improved source of drinking water whereas nine in every ten elderly from urban areas used improved source of drinking water. About four in every ten elderly in rural areas lived in pucca house and this proportion was almost double in urban areas.
Figure 1 shows the prevalence of diarrhoea/gastroenteritis or typhoid or jaundice/hepatitis. It was found that 14.8% (14.4–15.2) of elderly suffered from diarrhoea/gastroenteritis and 5.5% (5.2–5.7) suffered from typhoid and 2.5% (2.3–2.7) suffered from jaundice/hepatitis. The prevalence of water borne diseases among elderly was 19.5% (19.0–19.8).

Prevalence of diarrhoea/gastroenteritis or typhoid or jaundice/hepatitis among elderly in India, 2017–18
Prevalence of water borne diseases among elderly in India
Table 2 shows that there was a significant rural–urban difference in the prevalence of water borne diseases in India (difference: 10.2 percentage point). The prevalence of water borne disease among elderly in rural areas was 22.5% whereas in urban areas the prevalence was 12.2%. The rural–urban differences was highest among elderly who used unimproved toilet facility (difference: 17.1 percentage point), had 80 + years of age (difference: 14.4 percentage point), who belonged to other backward class (difference: 12.4 percentage point), richer elderly (difference: 12.3 percentage point), and those were not working (difference: 12.1 percentage point). Moreover, the prevalence of water borne diseases was higher among underweight elderly, and those who lived in kutcha houses irrespective to their place of residence.
Figure 2 shows state-wise prevalence of water borne diseases among elderly in India. The prevalence of water borne diseases was highest in Chhattisgarh (36.9 per cent), followed by Mizoram (35 per cent), Haryana (34.6 per cent), and Bihar (34 per cent). However, this prevalence was lowest in Kerala (3.5 per cent), followed by Goa (6.2 per cent), and Tamil Nadu (6.8 per cent).
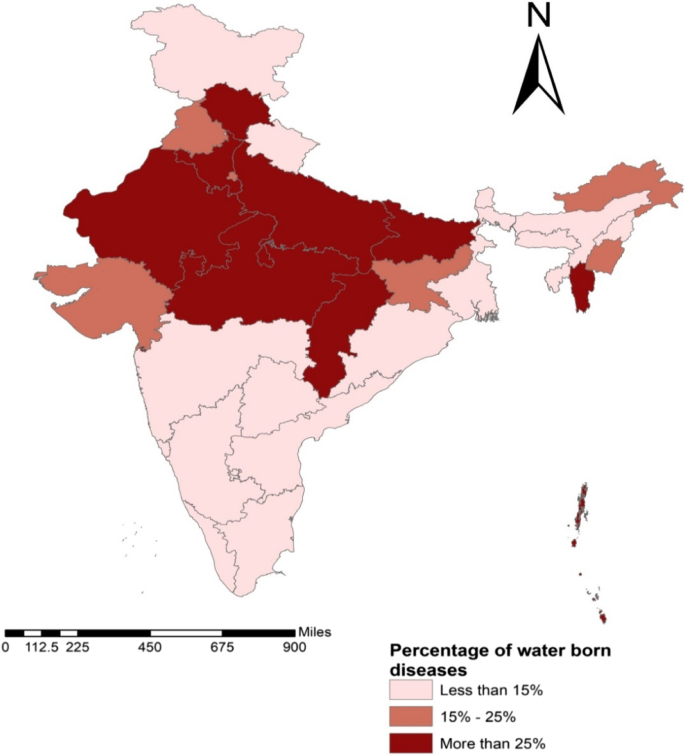
shows state-wise prevalence of water borne diseases among elderly in India
State-wise prevalence of water borne diseases in rural and urban areas in India
Table 3 presents the state-wise prevalence of water borne disease stratified by place of residence in India. In rural areas, the prevalence of water borne diseases was highest in Chhattisgarh (38.5 per cent) followed by Madhya Pradesh (36 per cent), Haryana (35.2 per cent), and Rajasthan (34.9 per cent) while for urban areas, water borne diseases was more prevalent in Bihar (36.3 per cent), followed by Mizoram (36.1 per cent), Himachal Pradesh (32.9 per cent), and Haryana (32.4 per cent) [Additional file Table A 2 ].
Estimates from logistic regression analysis for older adults who suffered from water borne diseases in India
Table 4 shows the adjusted odds ratio for elderly who suffered from water borne disease in India. It was revealed that the odds of water borne diseases was high in rural areas in reference to urban areas [AOR: 1.21; p < 0.05]. The likelihood of water borne diseases was significantly more among elderly female than male counterparts [AOR: 1.19; p < 0.05]. Moreover, the odds of water borne diseases were decreased with increase the level of education among elderly. The risk of water borne diseases was 12 per cent more among underweight elderly compared to overweight/obese elderly [AOR: 1.12; p < 0.05]. Similarly, elderly who used unimproved toilet facility [AOR: 1.22; p < 0.05] and unimproved source of drinking water [AOR: 1.37; p < 0.05] were 22 per cent and 37 per cent more likely to suffer from water borne diseases respectively, compared to their counterparts. The likelihood of water borne diseases was 27 per cent and 16 per cent more among scheduled tribe [AOR: 1.27; p < 0.05] and other backward class elderly [AOR: 1.16; p < 0.05] respectively, compared to scheduled caste elderly. With reference to elderly who belonged to North region, the likelihood of water borne diseases was 36 per cent more among elderly who belonged to Central region [AOR: 1.36; p < 0.05].
The present study tries to see the prevalence and predictors of water borne disease in India. The prevalence of water borne disease among the elderly is more in the rural (22.5%) areas compared to the urban counterparts (12.2%) with a significant absolute difference of about 10.2%. The percentage of elderly population with waterborne disease is more in the central Indian states like Chhattisgarh and Madhya Pradesh followed by the North Indian states. The result of logistic regression concludes that sex of the participant, educational status, working status, BMI, place of residence, type of toilet facility and water source are important determinants of water borne disease among elderly in India. The infectious disease distribution which includes water borne diseases involves complex social and demographic factors including human population density and behaviour, housing type and location, water supply, sewage and waste management systems, land use and irrigation systems, access to health care, and general environmental hygiene [ 30 ]. In the study the waterborne diseases include diarrhoea, typhoid and jaundice. Earlier studies have shown diarrhoea and its complication to be more among elderly people, particularly those who require long term care [ 31 ]. The study finding that waterborne diseases are more in the rural areas compared to the urban areas is also consistent with earlier studies which concluded diarrheal prevalence to be more in rural areas and also in Central part of the country [ 32 , 33 ]. A meta-analysis of typhoid prevalence in India concluded that this waterborne disease prevalence was more in the rural area with 0.09 lesser odds of having the disease in urban counterparts [ 34 ].
Our finding that waterborne disease prevalence vary with the anthropometric status as measured by BMI level with significantly higher odds of prevalence among the underweight compared to the overweight participants have theoretical justification as well. The relationship between malnutrition and the infection risk is bidirectional where infection adversely affects nutritional status through reductions in dietary intake and intestinal absorption, increased catabolism and sequestration of nutrients that are required for tissue synthesis and growth. On the other hand, malnutrition can predispose to infection because of its negative impact on the barrier protection afforded by the skin and mucous membranes and by inducing alterations in host immune function [ 35 , 36 , 37 ]. Earlier studies based on infectious disease risk among the children have indicated the educational status of mother as an important determinant with more infections among illiterate mothers [ 32 , 38 , 39 ]. The studies have debated that the disease risk is lesser among educated mothers because of hygiene practices, child feeding and caring practices, and improved living conditions. Similarly among the elderly participants as well educated people have a better understanding of the hygiene practices and feeding and caring habits and hence a reduced risk of waterborne infections.
The study finding that disease risk is more among population using unimproved sources of water and sanitation is consistent with earlier study which states drinking water, sanitation facilities and hygienic behaviour are the determining factors of health of household members [ 40 ]. A longitudinal study in the slums of Ethiopia shows sanitation facilities and hygienic condition of households were associated with acute diarrhoea [ 41 ]. Studies have also indicated improved water, sanitation and hygiene conditions of the households are accountable for diarrheal and other waterborne diseases [ 42 , 43 ]. Unimproved sources of drinking water, quality of drinking water, absences of sanitation facilities and garbage collection was associated with stomach problem in urban India [ 16 , 44 , 45 ].
India suffers from a higher burden of infectious disease particularly water bone disease due to a weak public drinking water distribution system [ 10 ]. The degraded water quality can contribute to water scarcity as it limits its availability for both human use and for the ecosystem. With more than 8% of elderly aged 60 years and above residing in India [ 19 ] it is important to see the prevalence of water borne disease among the increasing population of elderly as there is a need to protect the population since treatment cost is also not cheap. Moreover, studies indicating infectious disease among the elderly is very few [ 21 ]. Thus the present analysis is an important contribution in research related to health of the elder population.
Elderly living in rural areas are more prone to waterborne diseases. Use of unimproved water and absence of improved sanitation are major factors affecting waterborne disease among elderly. However the major limitation of the study is that the disease prevalence is based on self-reported morbidity status and lacks clinical verification, with a possibility of under reporting as well as over reporting and thus an underestimation or overestimation of the prevalence of the morbidities under study. However as is seen in various studies these self-reported measures or patient reported outcomes address issues that are of primary interest to the clinician and thus can be considered for measurement [ 23 ]. Consistent with findings from earlier literature regardless of whether there is under-reporting or over-reporting, the aforesaid socio-economic and demographic factors affect the pattern of morbidities associated with infections among elderly in India.
The elderly population might not be aware of the hygiene practices which adhere to the disease risk among this group. With age the antibody resistance falls and thus they might be well affected by the waterborne diseases. There is a need to focus on this population on preventing such bacterial diseases. This can be achieved by encouraging those aged 60 years and above as well as their caretakers to seek healthcare at early signs of infection. It also recommends making elderly aware of how to maintain the proper hygienic condition while availing the improved sanitation and water facilities provided to the people. The government should focus on providing safe water to the elderly population, train them to store water in a right and proper way.
Availability of data and materials
https://www.iipsindia.ac.in/content/LASI-data
United Nations. The Millennium Development Goals Report. United Nations 2015:72. 978-92-1-101320-7
Agingu JB. Levels and differentials of occurence of water borne diseases at Moi University. Kenya Acad Res Int. 2020;11:10–8.
Google Scholar
Bidhuri S, Taqi M, Khan MMA. Water-borne disease: Link between human health and water use in the Mithepur and Jaitpur area of the NCT of Delhi. J Public Heal. 2018;26:119–26. https://doi.org/10.1007/s10389-017-0835-y .
Article Google Scholar
Sharma S, Sachdeva P, Virdi JS. Emerging water-borne pathogens. Appl Microbiol Biotechnol. 2003;61(5-6):424-8. https://doi.org/10.1007/s00253-003-1302-y . Epub 2003 Apr 9.
Lopez AD, Murray CJ, eds., The global burden of disease: a comprehensive assessment of mortality and disability from diseases, injuries, and risk factors in 1990 and projected to 2020; summary. Harvard School of Public Health; 1996.
Prüss-Ustün A, Bartram J, Clasen T, Colford JM Jr, Cumming O, Curtis V, et al. Burden of disease from inadequate water, sanitation and hygiene in low-and middle-income settings: a retrospective analysis of data from 145 countries. Trop Med Int Heal. 2014;19:894–905.
Szálkai K. Water-Borne Diseases BT - The Palgrave Encyclopedia of Global Security Studies. In: Romaniuk S, Thapa M, Marton P, editors. Cham: Springer International Publishing; 2019. pp. 1–7. https://doi.org/10.1007/978-3-319-74336-3_562-1 .
WHO/UNICEF Joint Water Supply, & Sanitation Monitoring Programme. Progress on sanitation and drinking water: 2015 update and MDG assessment. World Health Organization; 2015.
UNICEF. Clean drinking water Ensuring survival and improved outcomes across all outcomes for every child. 2019.
Pathak H. Effect of Water Borne Diseases on Indian Economy…. Analele Univ Din Ordea, Ser Geogr. 2015;1:74–8.
Chabba APS. Water-Borne Diseases in India. 2013.
Oguntoke O, Aboderin OJ, Bankole AM. Association of water-borne diseases morbidity pattern and water quality in parts of Ibadan City. Nigeria. 2009;11:189–95.
CAS Google Scholar
Saravanan VS, Ayessa Idenal M, Saiyed S, Saxena D, Gerke S. Urbanization and human health in urban India: Institutional analysis of water-borne diseases in Ahmedabad. Health Policy Plan. 2016;31:1089–99. https://doi.org/10.1093/heapol/czw039 .
Article CAS PubMed Google Scholar
Karn SK, Harada H. Field survey on water supply, sanitation and associated health impacts in urban poor communities – a case from Mumbai City, India. Water Science and Technology. 2002;46(11-12):269-75.
Banda K, Sarkar R, Gopal S, Govindarajan J, Harijan BB, Jeyakumar MB, et al. Water handling, sanitation and defecation practices in rural southern India: a knowledge, attitudes and practices study. Trans R Soc Trop Med Hyg. 2007;101:1124–30. https://doi.org/10.1016/j.trstmh.2007.05.004 .
Article PubMed Google Scholar
Purohit BC. Health Impact of Water Borne Diseases and Regional Disparities in India. Int J Heal Sci Res Int J Heal Sci Res. 2012;2:1.
Pal M, Ayele Y, Hadush A, Panigrahi S, Jadhav VJ. Public Health Hazards Due to Unsafe Drinking Water. Air Water Borne Dis. 2018;7:1–6. https://doi.org/10.4172/2167-7719.1000138 .
Naumova EN, Egorov AI, Morris RD, Griffiths JK. The elderly and waterborne Cryptosporidium infection: Gastroenteritis hospitalizations before and during the 1993 Milwaukee outbreak. Emerg Infect Dis. 2003;9:418–25. https://doi.org/10.3201/eid0904.020260 .
Article PubMed PubMed Central Google Scholar
Office of the Registrar General & Census Commissioner of India. Census of India 2011 provisional population totals. Ministry of Home Affairs, Government of India. 2011.
Bhagat RB. "Ageing in India: The Class Demographics and Policy Issues." The Trajectory of India’s Middle Class. Economy, Ethics and Etiquette. 2015:248-263.
Steens A, Eriksen HM, Blystad H. What are the most important infectious diseases among those ≥65 years: a comprehensive analysis on notifiable diseases, Norway, 1993-2011. BMC Infect Dis. 2014;14:57. https://doi.org/10.1186/1471-2334-14-57 .
International Institute for Population Sciences (IIPS), NPHCE, MoHFW, et al. Longitudinal Ageing Study in India (LASI) Wave 1. Mumbai, India (2020).
McKenna SP. Measuring patient-reported outcomes: Moving beyond misplaced common sense to hard science. BMC Med. 2011;9:86. https://doi.org/10.1186/1741-7015-9-86 .
Fauziana R, Jeyagurunathan A, Abdin E, Vaingankar J, Sagayadevan V, Shafie S, et al. Body mass index, waist-hip ratio and risk of chronic medical condition in the elderly population: Results from the Well-being of the Singapore Elderly (WiSE) Study. BMC Geriatr. 2016;16:1–9. https://doi.org/10.1186/s12877-016-0297-z .
Kumar P, Patel R, Chauhan S, Srivastava S, Khare A, Kumar PK. Does socio-economic inequality in infant mortality still exists in India? An analysis based on National Family Health Survey 2005–06 and 2015–16. Clin Epidemiol Glob Heal. 2020. https://doi.org/10.1016/j.cegh.2020.07.010 .
Zacharias A, Vakulabharanam V. Caste Stratification and Wealth Inequality in India. World Dev. 2011. https://doi.org/10.1016/j.worlddev.2011.04.026 .
Koopman PAR. Confidence Intervals for the Ratio of Two Binomial Proportions. Biometrics. 1984. https://doi.org/10.2307/2531405 .
King J. Binary logistic regression. In Osborne, J. (Ed.), Best practices in quantitative methods (pp. 358-384). SAGE Publications, Inc.; 2008. https://dx.doi.org/10.4135/9781412995627 .
Lewis-Beck M, Bryman A, Futing LT. Variance Inflation Factors. SAGE Encycl Soc Sci Res Methods. 2012. https://doi.org/10.4135/9781412950589.n1067 .
Ramana Dhara V, Schramm PJ, Luber G. Climate change & infectious diseases in India: Implications for health care providers. Indian J Med Res. 2013;138:847–52.
PubMed PubMed Central Google Scholar
Adiga GU, Dharmarajan TS, Pitchumoni CS. Diarrhea in older adults. Pract Gastroenterol. 2005;29:63–82.
Paul P. Socio-demographic and environmental factors associated with diarrhoeal disease among children under five in India. BMC Public Health. 2020;20:1–11. https://doi.org/10.1186/s12889-020-09981-y .
Vijayan B, Ramanathan M. Prevalence and clustering of diarrhoea within households in India: some evidence from NFHS-4, 2015–16. J Biosoc Sci. 2013;53:108–20. https://doi.org/10.1017/s0021932020000073 .
John J, Van Aart CJC, Grassly NC. The Burden of Typhoid and Paratyphoid in India: Systematic Review and Meta-analysis. PLoS Negl Trop Dis. 2016;10:1–14. https://doi.org/10.1371/journal.pntd.0004616 .
Article CAS Google Scholar
Martorell R, Habicht JP, Yarbrough C, Lechtig A, Klein RE, Western KA. Acute morbidity and physical growth in rural Guatemalan children. Am J Dis Child. 1975;129:1296–301. https://doi.org/10.1001/archpedi.1975.02120480022007 .
Palmer DL, Koster FT, Alam AKMJ, Islam MR. Nutritional Status: A Determinant of Severity of Diarrhea in Patients with Cholera. J Infect Dis. 1976;134:8–14. https://doi.org/10.1093/infdis/134.1.8 .
Kenneth BH. Symposium: Nutrition and Infection, Prologue and Progress Since 1968: Diarrhea and Malnutrition. J Nutr. 2003;133:336–40.
Sarker AR, Sultana M, Mahumud RA, Sheikh N, Van Der Meer R, Morton A. Prevalence and Health Care-Seeking Behavior for Childhood Diarrheal Disease in Bangladesh. Glob Pediatr Health. 2016;3:2333794X16680901. https://doi.org/10.1177/2333794X16680901 .
Asfaha KF, Tesfamichael FA, Fisseha GK, Misgina KH, Weldu MG, Welehaweria NB, Gebregiorgis YS. Determinants of childhood diarrhea in Medebay Zana District, Northwest Tigray, Ethiopia: a community based unmatched case-control study. BMC Pediatr. 2018;18(1):120. https://doi.org/10.1186/s12887-018-1098-7 .
Troeger C, Forouzanfar M, Rao PC, Khalil I, Brown A, Reiner RC, et al. Estimates of global, regional, and national morbidity, mortality, and aetiologies of diarrhoeal diseases: a systematic analysis for the Global Burden of Disease Study 2015. Lancet Infect Dis. 2017;17:909–48. https://doi.org/10.1016/S1473-3099(17)30276-1 .
Adane M, Mengistie B, Kloos H, Medhin G, Mulat W. Sanitation facilities, hygienic conditions, and prevalence of acute diarrhea among underfive children in slums of Addis Ababa, Ethiopia: Baseline survey of a longitudinal study. PLoS ONE. 2017;12:1–18. https://doi.org/10.1371/journal.pone.0182783 .
Bakir H, Hadi M, Jurdi M. Towards a renewed public health regulatory and surveillance role in water, sanitation and hygiene. East Mediterr Health J. 2017;23(8):525-6.
Fan VYM, Mahal A. What prevents child diarrhoea? the impacts of water supply, toilets, and hand-washing in rural India. J Dev Eff. 2011;3:340–70. https://doi.org/10.1080/19439342.2011.596941 .
Kumar S, Vollmer S. Does access to improved sanitation reduce childhood diarrhea in rural India? Health Econ. 2013;22(4):410-27. https://doi.org/10.1002/hec.2809 . Epub 2012 Mar 22.
Patel SK., Pradhan MR, Patel S. Water, sanitation, and hygiene (WASH) conditions and their association with selected diseases in urban India. J Popul and Soc Stud [JPSS]. 2020;28(2):103-15.
Download references
Acknowledgements
Not applicable.
Accordance statement
This study is not based any experiments on humans data.
Author information
Authors and affiliations.
Indian Health Action Trust, Lucknow, India
Pradeep Kumar
Mamta Health Institute for Mother and Child, Delhi, India
Shobhit Srivastava
International Institute for Population Sciences, Mumbai, India
Adrita Banerjee & Snigdha Banerjee
You can also search for this author in PubMed Google Scholar
Contributions
Conception and design of study: PK, and SS; analysis and interpretation of data: PK and SS; drafting the manuscript: PK, SS, AB, SB; All the authors have read and approved the final manuscript.
Corresponding author
Correspondence to Snigdha Banerjee .
Ethics declarations
Ethics approval and consent to participate.
The data is freely available on request and survey agencies that conducted the field survey for the data collection have collected a prior consent from the respondent. The ethical clearance was provided by Indian Council of Medical Research (ICMR), India.
Consent for publication
Competing interests, additional information, publisher’s note.
Springer Nature remains neutral with regard to jurisdictional claims in published maps and institutional affiliations.
Supplementary Information
Additional file 1., rights and permissions.
Open Access This article is licensed under a Creative Commons Attribution 4.0 International License, which permits use, sharing, adaptation, distribution and reproduction in any medium or format, as long as you give appropriate credit to the original author(s) and the source, provide a link to the Creative Commons licence, and indicate if changes were made. The images or other third party material in this article are included in the article's Creative Commons licence, unless indicated otherwise in a credit line to the material. If material is not included in the article's Creative Commons licence and your intended use is not permitted by statutory regulation or exceeds the permitted use, you will need to obtain permission directly from the copyright holder. To view a copy of this licence, visit http://creativecommons.org/licenses/by/4.0/ . The Creative Commons Public Domain Dedication waiver ( http://creativecommons.org/publicdomain/zero/1.0/ ) applies to the data made available in this article, unless otherwise stated in a credit line to the data.
Reprints and permissions

About this article
Cite this article.
Kumar, P., Srivastava, S., Banerjee, A. et al. Prevalence and predictors of water-borne diseases among elderly people in India: evidence from Longitudinal Ageing Study in India, 2017–18. BMC Public Health 22 , 993 (2022). https://doi.org/10.1186/s12889-022-13376-6
Download citation
Received : 25 November 2021
Accepted : 28 April 2022
Published : 17 May 2022
DOI : https://doi.org/10.1186/s12889-022-13376-6
Share this article
Anyone you share the following link with will be able to read this content:
Sorry, a shareable link is not currently available for this article.
Provided by the Springer Nature SharedIt content-sharing initiative
- Water-borne diseases
- Older adults
- Rural–urban divide
BMC Public Health
ISSN: 1471-2458
- General enquiries: [email protected]
Prevalence of water-borne diseases in western India: dependency on the quality of potable water and personal hygiene practices
- Journal of Water Sanitation and Hygiene for Development 11(11)

- Lund University

- National Environmental Engineering Research Institute

- Topiwala National Medical College & B. Y. L. Nair Charitable Hospital
Abstract and Figures

Discover the world's research
- 25+ million members
- 160+ million publication pages
- 2.3+ billion citations

- N. C. Ghosh

- Benjamin F. Arnold

- PLOS NEGLECT TROP D

- Stephanie Ogden

- Jürg Utzinger
- Lanakila McMahan

- Jorge Reyes

- John O. Rawlings
- Sastry G. Pantula

- G. S. Watson
- Recruit researchers
- Join for free
- Login Email Tip: Most researchers use their institutional email address as their ResearchGate login Password Forgot password? Keep me logged in Log in or Continue with Google Welcome back! Please log in. Email · Hint Tip: Most researchers use their institutional email address as their ResearchGate login Password Forgot password? Keep me logged in Log in or Continue with Google No account? Sign up
Volume 29, Number 7—July 2023
Estimating Waterborne Infectious Disease Burden by Exposure Route, United States, 2014

Cite This Article
More than 7.15 million cases of domestically acquired infectious waterborne illnesses occurred in the United States in 2014, causing 120,000 hospitalizations and 6,600 deaths. We estimated disease incidence for 17 pathogens according to recreational, drinking, and nonrecreational nondrinking (NRND) water exposure routes by using previously published estimates. In 2014, a total of 5.61 million (95% credible interval [CrI] 2.97–9.00 million) illnesses were linked to recreational water, 1.13 million (95% CrI 255,000–3.54 million) to drinking water, and 407,000 (95% CrI 72,800–1.29 million) to NRND water. Recreational water exposure was responsible for 36%, drinking water for 40%, and NRND water for 24% of hospitalizations from waterborne illnesses. Most direct costs were associated with pathogens found in biofilms. Estimating disease burden by water exposure route helps direct prevention activities. For each exposure route, water management programs are needed to control biofilm-associated pathogen growth; public health programs are needed to prevent biofilm-associated diseases.
Waterborne infectious diseases substantially affect public health in the United States, despite widespread treatment and disinfection of drinking water systems and recreational water venues such as swimming pools and hot tubs. Cases of waterborne infections are estimated at >7.15 million annually in the United States, causing 120,000 hospitalizations and 6,600 deaths ( 1 ). Pathogens found in biofilms, such as nontuberculous mycobacteria (NTM) and Legionella bacteria, are predominant causes of hospitalizations and deaths from waterborne diseases in the United States. Waterborne pathogen exposure routes include swimming, drinking water, bathing, or breathing in aerosolized water.
Before widespread application of drinking water disinfection treatments, cholera and typhoid were major causes of death in the United States. Supplying treated, safe drinking water dramatically reduced the incidence of those diseases; in the past 50 years, outbreaks from large public drinking water systems have occurred less frequently ( 2 – 4 ), likely because of operational regulations and improvements. However, aging infrastructures and climate change negatively affect drinking water systems ( 5 ). Furthermore, 43 million US residents are served by private wells or domestic water systems that are not regulated by the Environmental Protection Agency Safe Drinking Water Act, leaving homeowners responsible for maintaining and monitoring water quality in their wells ( 6 ). During 1971–2008, one third of reported disease outbreaks from drinking water were linked to private wells ( 7 ). The complexity of water distribution has also increased; 6 million miles of plumbing inside buildings (known as premise plumbing) support drinking water, sanitation, hygiene, cooling, and heating needs in the United States ( 8 , 9 ). Premise plumbing water quality can be compromised when water is stagnant or disinfectant concentrations are reduced, thereby promoting microbial pathogen growth and biofilm formation ( 10 ). Exposure to biofilm-related pathogens can occur through contact with, ingestion of, or aerosol inhalation of contaminated water from different sources, such as showerheads, hot tubs, building cooling towers, or decorative fountains.
Filtration and chlorination of water in swimming pools to remove and inactivate pathogens were introduced in the early 1900s. Illnesses caused by recreational water contact still occur, partly because of lack of national standards across the United States for treated recreational water venues, such as pools, hot tubs, and splash pads, and design innovations that have increased venue sizes and complexity and have changed how persons are exposed (e.g., increased hot tub water aerosols).
Swimming in untreated recreational water venues (lakes, rivers, and oceans) can cause outbreaks predominantly linked to norovirus, Shiga toxin–producing Escherichia coli , Cryptosporidium spp., and Shigella spp. ( 11 , 12 ). Those enteric pathogens can be introduced into untreated recreational water through human feces or vomit, stormwater runoff, sewage or septic system malfunctions, or animal waste and can then be transmitted to persons who ingest the contaminated water.
Annual incidences of illness associated with drinking water in community drinking water systems have been estimated at 4–20 million ( 13 – 16 ). Illnesses associated with recreational contact with untreated or natural waters have also been estimated ( 17 , 18 ), and 1 study reported 90 million illnesses annually attributable to recreational water exposure. We previously estimated the overall burden of waterborne diseases in the United States ( 1 ). In this study, we quantified exposure-specific diseases from 3 water sources—recreational, drinking, and nonrecreational nondrinking (NRND) water—by using a multiplier model of surveillance data from 2014. Our goals were to estimate disease burdens by water exposure route, help guide disease prevention measures, determine key prevention partners, and prioritize limited resources.
We apportioned waterborne diseases to recreational, drinking, or NRND water categories as previously described in a structured expert judgment study ( 19 ) ( Appendix Table 1). Recreational water is defined as water used for recreational activities, such as aquatic venues or natural bodies of water. Drinking water is used primarily for drinking but can include other domestic uses, such as washing or showering. Drinking water can come from a public water system, private well, or commercially bottled sources. NRND water is used for purposes other than recreation or consumption, such as agriculture, manufacturing or cooling equipment, or medical treatment; this category also includes backcountry streams and flood waters. Examples of diseases transmitted by NRND water include Legionnaires’ disease associated with a cooling tower, giardiasis transmitted by drinking untreated water from a stream, and Vibrio spp. wound infections after wading through flood waters.
We estimated the total number of cases for 17 diseases or syndromes in 2014 in the United States ( Appendix ): acute otitis externa, campylobacteriosis, cryptosporidiosis, giardiasis, Legionnaires’ disease, NTM infection, norovirus infection, Pseudomonas pneumonia, Pseudomonas septicemia, salmonellosis (nontyphoidal), Shiga toxin–producing Escherichia coli infection with an O157 or non-O157 serogroup, shigellosis, and vibriosis caused by Vibrio alginolyticus , V . parahaemolyticus , V . vulnificus , and other non– V . cholerae spp. ( 1 ) ( Appendix Table 2). We divided total waterborne disease estimates previously reported ( 1 ) by structured expert judgment study estimates ( 19 ) to produce 2014 burden estimates for recreational, drinking, and NRND water exposure ( Appendix Table 3). We quantified direct healthcare costs of treat-and-release emergency department (ED) visits and hospitalizations, measured by insurers and out-of-pocket payments, by using MarketScan data, as previously described ( 1 ).
We used anonymized administrative surveillance and literature data from 2000–2015 and based all estimates on the 2014 US population (318.6 million persons); at the time of analysis, 2014 was the most recent year for which all data were available. Data sources have been described previously ( 1 ). We estimated values by using probabilistic multiplier models ( Appendix ); each model input had an associated uncertainty estimate represented by a distribution of plausible values ( Appendix Figure). Uncertainty in final estimates for each type of water exposure was a cumulative effect, incorporating the uncertainties of each model input ( 1 , 19 ). We obtained count distributions by using 100,000 iterations to generate point estimates of means and corresponding 95% credible intervals (CrIs; the 2.5th–97.5th percentiles of empirical distribution). We generated all-disease totals for each outcome by sampling from the distributions generated for each disease. We used SAS version 9.4 (SAS Institute, https://www.sas.com ) and R version 3.5.1 (The R Project for Statistical Computing, https://www.r-project.org ) for analyses.
In 2014, a total of 5.61 million (95% CrI 2.97–9.00 million) domestically-acquired infectious waterborne illnesses were associated with recreational water, 1.13 million (95% CrI 255,000–3.54 million) with drinking water, and 407,000 (95% CrI 72,800–1.29 million) with NRND water ( Table 1 ). Acute otitis externa caused most illnesses associated with recreational water (79%) and NRND water (27%), and norovirus infection was the leading illness associated with drinking water (53%).
Recreational water exposure was linked to ≈552,000 (95% CrI 320,000–808,000) ED visits, drinking water to 31,600 (95% CrI 4,070–133,000) visits, and NRND water exposure to 17,200 (95% CrI 951–69,400) visits ( Table 2 ). Acute otitis externa caused the most ED visits for each water exposure; 97% of those associated with recreational water, 48% associated with drinking water, and 78% associated with NRND water.
Hospitalizations
Recreational water was linked to ≈42,300 (95% CrI 26,500–63,000), drinking water to 47,700 (95% CrI 24,600–72,800), and NRND water to 27,900 (95% CrI 13,200–48,900) hospitalizations ( Table 3 ). Acute otitis externa caused most (52%) hospitalizations associated with recreational water exposure; NTM infections caused most hospitalizations associated with drinking water (73%) and NRND water (37%) exposures.
Recreational water exposure was linked to ≈1,290 (95% CrI 591–2,520) deaths, drinking water to 3,300 (95% CrI 1,630–5,180) deaths, and NRND water exposure to 2,040 (95% CrI 909–3,690) deaths ( Table 4 ). NTM infections caused most deaths for each water exposure type; 37% of NTM-related deaths were associated with recreational water, 78% with drinking water, and 37% with NRND water.
Direct healthcare costs for ED visits and hospitalizations
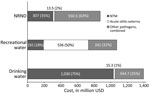
Figure . Direct healthcare costs for emergency department visits and hospitalizations in study estimating waterborne infectious disease burden by exposure route, United States, 2014. Estimated combined costs are shown in US dollars...
Illnesses associated with drinking water represented the largest portion (42%) of combined costs, totaling $1.39 billion (95% CrI $364.00 million–$4.81 billion) ( Figure ; Appendix Tables 4, 5). Illnesses associated with recreational water exposures represented 32%, totaling $1.07 billion (95% CrI $439.00 million–$2.67 billion) of combined costs; illnesses associated with NRND exposures made up 26%, totaling $871.00 million (95% CrI $240.00 million–$2.64 billion) of combined costs. Four infection types accounted for 89% of $3.33 billion total costs; NTM infection was responsible for 46%, acute otitis externa for 17%, Pseudomonas pneumonia for 14%, and Legionnaires’ disease for 12% of combined costs ( Appendix Tables 4, 5).
Of the estimated 7.15 million infectious waterborne illnesses in 2014 in the United States, 78% of illnesses were attributed to recreational water, 16% to drinking water, and 6% to NRND water. Drinking water exposure caused 40% of hospitalizations and 50% of deaths, recreational water exposure 36% of hospitalizations and 20% of deaths, and NRND exposure 24% of hospitalizations and 30% of deaths. ED visits and hospitalizations from exposure to drinking or recreational water exposure cost >$2 billion annually.
Acute otitis externa, which can be acutely painful and cause itchiness, drainage, and swelling, was the most common recreational water–associated illness. Risks for acute otitis externa have been correlated with water quality measures, levels of Pseudomonas and other pathogens in water, and increased bather load in recreational water venues in some studies but not others ( 20 – 23 ). Water can displace the protective coating of cerumen (ear wax) in the ear canal, leaving the outer ear more vulnerable to infection by endogenous flora. Whether otitis externa is caused by endogenous flora or contamination introduced by water remains unclear. In the structured expert judgment study ( 19 ), experts were asked to consider this question while estimating the proportion of otitis externa transmitted through water versus other pathways. They estimated 81% (95% uncertainty interval 67%–95%) of Pseudomonas otitis externa was transmitted through water ( 19 ). In response to the absence of national standards for recreational water venues, the Centers for Disease Control and Prevention (CDC) participated in the development and updating of the Model Aquatic Health Code (MAHC) ( 24 ). For treated water venues, the MAHC includes guidance for health authorities and aquatics sector to minimize risks for acute otitis externa and other illnesses and injuries ( 24 , 25 ). For both treated and untreated venues, risk for acute otitis externa can be minimized by keeping ears as dry as possible when swimming and ensuring ears are dry after swimming. Recreational swimmers and parents of young swimmers can educate themselves about steps to minimize the risks for infection from enteric pathogens at trusted sites, such as the CDC’s healthy swimming webpage ( 26 ). Signage at treated and untreated recreational water venues ( 12 ) and adoption of CDC’s MAHC for treated public recreational water venues can further improve prevention. The number of illnesses and ED visits for acute otitis externa caused by drinking water exposure might seem counterintuitive, but drinking water was defined as water used for drinking, bathing, or showering.
Cryptosporidium and Legionella spp. infections in humans were identified in the late 1970s, and outbreaks associated with those pathogens in treated recreational water venues were identified soon after. In subsequent decades, reported incidences of treated recreational water–associated outbreaks increased substantially; Cryptosporidium , Legionella , and Pseudomonas spp. were the predominant etiologies ( 27 ). P. aeruginosa is a major cause of acute otitis externa and folliculitis or hot tub rash in addition to pneumonia and septicemia ( 28 ). Infectious Cryptosporidium sp. oocysts have tough outer shells that provide extreme chlorine tolerance, whereas Legionella and Pseudomonas spp. thrive in biofilms, where they are protected from chlorine inactivation.
Reported infectious disease outbreaks associated with public drinking water systems have decreased since the 1970s, likely because of federal management and treatment regulations that address enteric pathogens ( 3 ). However, outbreaks associated with individual water systems, such as private wells, and premise plumbing deficiencies have not decreased ( 3 ). Our analysis affirms that most hospitalizations and deaths associated with drinking water exposure were linked to biofilm-associated pathogens, not to pathogens causing enteric disease. Legionella bacteria are biofilm-associated pathogens and have become the most frequently reported cause of disease outbreaks associated with drinking water. Our analysis revealed that NTM infections were the leading cause of drinking water–associated illnesses, ED visits, hospitalizations, and deaths; NTM infections were the most common cause of death associated with all 3 water exposure routes. However, NTM infections are not nationally notifiable diseases; thus, cases are not consistently reported to public health authorities and outbreaks might remain undetected. Improving NTM illness reporting could lead to greater outbreak detection and inform disease prevention strategies for water systems. The Council of State and Territorial Epidemiologists has a standardized case definition for extrapulmonary NTM infections (opportunistic infections of wounds, soft tissue, or joints) to increase reportability, ensure consistency in reporting, and help identify outbreaks ( 29 ). Thus far, the state of Oregon has adopted this definition for their surveillance program ( 30 ), and other sites are piloting NTM surveillance programs ( 31 ).
NRND water includes water used for agriculture, such as for irrigation or livestock; industry, such as manufacturing or cooling equipment; medical procedures, such as medical devices, washing surgical tools and equipment, and hydrotherapy; and backcountry streams or floodwaters ( 19 ). NRND water made up 87% of the 322 billion gallons of water used each day in the United States in 2015; of that volume, 2 billion gallons were used for livestock, 118 billion gallons for irrigation, 7.55 billion gallons for aquaculture, and 133 billion gallons for thermoelectric power ( 6 ). NRND water is associated with a small portion of reported waterborne illnesses but is responsible for one quarter of waterborne disease–associated hospitalizations and one third of associated deaths.
Waterborne pathogens, such as NTM, Pseudomonas spp., and Legionella spp., occur naturally in freshwater and can colonize other environments, particularly large complex equipment that is not properly maintained. Those pathogens are aerosolized in water droplets produced during use, which can be inhaled. Water management programs are recommended to minimize growth and spread of pathogens in engineered systems ( 32 – 34 ). Resources can be found on the CDC Environmental Health Services safe water program webpage ( 35 ), guide for developing a water management program to reduce Legionella growth and spread in buildings ( 36 ), Legionella control toolkit ( 37 ), and guide for reducing risk from water ( 38 ). Water management programs in healthcare facilities are also critical for protecting vulnerable patient populations, staff, and visitors and might require specific considerations for a wide range of biofilm-associated pathogens, including NTM, Pseudomonas , and Legionella spp. ( 39 – 42 ). Interdisciplinary collaboration is needed for planning future management of complex water systems and investigating illnesses and outbreaks.
Reducing risks for illness from biofilms in water systems is difficult. Biofilms can persist despite standard water treatment processes. Biofilms are complex ecosystems; control is complicated by structural issues (e.g., pipe characteristics), system operational issues (e.g., water age, temperature, and residual disinfectants), and water end-user behaviors. Changes designed to reduce prevalence of 1 microbial constituent can sometimes produce unintended consequences, such as proliferation of NTM, if a comprehensive approach to biofilm control is not considered ( 43 ). NTM are persistent pathogens; despite standard treatment, NTM species have been recovered from surface water treatment plants, biofilms in sand filters, ozonated water, biofilms from granular activated carbon filters, and activated carbon-filtered water. Even municipal systems that incorporate full treatment chains, including 2 filtration and 2 disinfection stages, might not effectively prevent NTM growth ( 44 ). Because climate change continues to create extreme weather events and increase water temperatures, more stress will be placed on aging water infrastructure; biofilm-associated pathogens will continue to proliferate ( 5 ), highlighting the need for biofilm-focused control programs.
Prevention of biofilm-associated organisms is an emerging field, and more scientific evidence is needed to determine best practices for public water systems and premise plumbing and identify effective prevention strategies for homeowners and building managers. Persons can reduce their risk for illness from a biofilm-associated pathogen by flushing rarely used faucets and showerheads, flushing water heaters, and maintaining specific water heater temperatures. Resources for risk reduction can be found on CDC’s Preventing Waterborne Germs at Home webpage ( 45 ).
Most previous waterborne infectious disease estimates are not directly comparable with results from this study because of differing methods used to generate estimates. Many studies have attempted to estimate a burden for 1 disease or aspect of drinking or recreational water exposure. Two studies from a workshop convened by CDC and the Environmental Protection Agency used 2 different methods to estimate the number of cases of acute gastrointestinal illness in public drinking water systems only. Those studies estimated 4–16 million acute gastrointestinal illness cases per year ( 13 , 16 ). A 2008 study estimated all illnesses associated with public drinking water systems at 19 million cases per year ( 14 ). Previous publications have used cohort studies to estimate gastrointestinal illness from water recreational activities and percentages of economic burden from recreational water exposure ( 17 , 46 ). In Canada, rates for waterborne disease per 100,000 persons were estimated by using literature, clinical input, and administrative data ( 47 ). Our estimates differ from previous work because we focused on specific pathogens, including those causing nongastrointestinal diseases, and we provide estimates for recreational water, drinking water, and NRND water exposure routes.
The first limitation of our study is that the estimates relied on a series of multipliers. Although we attempted to account for uncertainty in the multiplier estimates, biased multipliers will yield biased estimates. We used attribution estimates for proportions of disease caused by each water exposure route, derived from a structured expert judgment study. Structured expert judgment is used when data are sparse and relies on expert opinion. Statistical techniques are used to combine expert opinions into a single estimate with an uncertainty interval. Various weighting techniques can be used to increase accuracy or informativeness (narrowness of the uncertainty interval), but tradeoffs between accuracy and informativeness occur. For some individual estimates, including norovirus infection, NTM infection, and acute otitis externa, uncertainty intervals were wide, reflecting uncertainty about the proportion of disease transmitted via water. Second, as with previous waterborne disease burden estimates ( 1 ), we included estimates of 17 infectious diseases that had adequate data available. Other waterborne infectious diseases were excluded, such as Pseudomonas folliculitis or viral gastroenteritis not caused by norovirus. We also excluded noninfectious diseases caused by exposure to contaminated water, such as from lead or harmful algae-derived toxins, and did not evaluate long-term chronic effects of infectious or noninfectious diseases. In addition, we excluded foodborne illnesses, such as salmonellosis from lettuce or norovirus disease from shellfish, where contaminated water might have been responsible. Third, this study used data from hospital and ED billing databases. Billing records are not medical records and might reflect diagnoses that result in reimbursement from insurance companies rather than true assessments by clinicians. Fourth, those billing databases use International Classification of Diseases, 9th Revision, Clinical Modification, coding to classify diagnoses; some illnesses might not perfectly match existing codes. Fifth, we provide estimates for 2014, and illness burdens, healthcare costs, and clinical practices have likely changed since then. Sixth, we assumed that severity of illness was independent of exposure route. For example, if salmonellosis was more severe when transmitted by drinking water than by recreational water, the multipliers used in calculating estimates did not account for this. Seventh, many diseases in this analysis might be more severe in persons with compromised immunity, but we did not attempt to apportion the burden of illness between waterborne illnesses and immune status. Finally, reliably distinguishing between illnesses linked to premise plumbing water exposure and distribution system exposure was difficult, which was reflected in 95% CrI widths for drinking water and NRND estimates for some pathogens, including NTM. More research is needed in this area because federal regulations for distribution systems differ from building codes and state and local laws that regulate premise plumbing.
In conclusion, quantifying the burden of illness linked to drinking, recreational, and NRND water is an essential factor for addressing biofilm-associated pathogens, because prevention measures and vital partners differ for each exposure route. Estimating disease burden by water exposure route will help direct prevention activities and prioritize limited resources. Measures for preventing illnesses from recreational water use, such as those in the MAHC for treated recreational water venues, could reduce disease burden associated with recreational water exposure. Incorporating water management programs and control measures for systems that are common sources of biofilm pathogen exposure into building and public health codes is crucial for reducing risks for biofilm-associated pathogen exposure in drinking water and NRND water systems ( 34 ). In addition, improved surveillance and reporting of biofilm-associated illnesses could lead to the timely detection and investigation of outbreaks and inform disease prevention activities. Interdisciplinary partnerships among public health agencies and industries that use NRND water are pivotal to reduce incidences of waterborne diseases. For drinking water and NRND water systems, addressing factors that promote biofilm growth (stagnation, temperature, or lack of disinfection) could reduce growth of biofilm-associated pathogens. Facilities that serve persons at increased risk for infections, such as healthcare facilities, should review water system design and operations to decrease risks from biofilm-associated pathogens. Additional research on the roles of pipe material, water chemistry, and optimal water distribution system management could provide insights into improving prevention actions. As climate change continues to place increased stress on water systems, improved prevention and maintenance strategies should be developed to prevent illness from all types of water exposure.
Ms. Gerdes is an epidemiologist in the Division of Foodborne, Waterborne, and Environmental Diseases, National Center for Emerging and Zoonotic Infectious Diseases, Centers for Disease Control and Prevention, Atlanta, Georgia, USA. Her research interests focus on data analysis and translating epidemiologic findings and works to improve surveillance systems for waterborne disease and wastewater.
Acknowledgment
We thank Jennifer Collins, Patricia M. Griffin, Noelle-Angelique Molinari, and Logan C. Ray for reviewing this manuscript and for their subject matter expertise.
- Collier SA , Deng L , Adam EA , Benedict KM , Beshearse EM , Blackstock AJ , et al. Estimate of burden and direct healthcare cost of infectious waterborne disease in the United States. Emerg Infect Dis . 2021 ; 27 : 140 – 9 . DOI PubMed Google Scholar
- Benedict KM , Reses H , Vigar M , Roth DM , Roberts VA , Mattioli M , et al. Surveillance for waterborne disease outbreaks associated with drinking water—United States, 2013–2014. MMWR Morb Mortal Wkly Rep . 2017 ; 66 : 1216 – 21 . DOI PubMed Google Scholar
- Craun GF , Brunkard JM , Yoder JS , Roberts VA , Carpenter J , Wade T , et al. Causes of outbreaks associated with drinking water in the United States from 1971 to 2006. Clin Microbiol Rev . 2010 ; 23 : 507 – 28 . DOI PubMed Google Scholar
- Cutler D , Miller G . The role of public health improvements in health advances: the twentieth-century United States. Demography . 2005 ; 42 : 1 – 22 . DOI PubMed Google Scholar
- US Global Change Research Program . The impacts of climate change on human health in the United States. A scientific assessment, 2016 . Chapter 6. Climate impacts on water-related illness [ cited 2023 Feb 7 ]. https://health2016.globalchange.gov/high/ClimateHealth2016_FullReport.pdf
- US Geological Survey . Estimated use of water in the United States in 2015. Circular 1441, 2018 [ cited 2023 Jan 15 ]. https://pubs.er.usgs.gov/publication/cir1441
- Wallender EK , Ailes EC , Yoder JS , Roberts VA , Brunkard JM . Contributing factors to disease outbreaks associated with untreated groundwater. Ground Water . 2014 ; 52 : 886 – 97 . DOI PubMed Google Scholar
- Environmental Protection Agency . Community water system survey 2000 . Volume 1: overview [ cited 2022 Dec 11 ]. https://nepis.epa.gov/Exe/ZyPDF.cgi?Dockey=20001ZK5.txt
- National Research Council . Drinking water distribution systems: assessing and reducing risks. Washington: National Academies Press; 2006 .
- Falkinham JO III , Hilborn ED , Arduino MJ , Pruden A , Edwards MA . Epidemiology and ecology of opportunistic premise plumbing pathogens: Legionella pneumophila, Mycobacterium avium , and Pseudomonas aeruginosa. Environ Health Perspect . 2015 ; 123 : 749 – 58 . DOI PubMed Google Scholar
- Graciaa DS , Cope JR , Roberts VA , Cikesh BL , Kahler AM , Vigar M , et al. Outbreaks associated with untreated recreational water—United States, 2000–2014. MMWR Morb Mortal Wkly Rep . 2018 ; 67 : 701 – 6 . DOI PubMed Google Scholar
- Vanden Esschert KL , Mattioli MC , Hilborn ED , Roberts VA , Yu AT , Lamba K , et al. Outbreaks associated with untreated recreational water—California, Maine, and Minnesota, 2018–2019. MMWR Morb Mortal Wkly Rep . 2020 ; 69 : 781 – 3 . DOI PubMed Google Scholar
- Messner M , Shaw S , Regli S , Rotert K , Blank V , Soller J . An approach for developing a national estimate of waterborne disease due to drinking water and a national estimate model application. J Water Health . 2006 ; 4 ( Suppl 2 ): 201 – 40 . DOI PubMed Google Scholar
- Reynolds KA , Mena KD , Gerba CP . Risk of waterborne illness via drinking water in the United States. Rev Environ Contam Toxicol . 2008 ; 192 : 117 – 58 . PubMed Google Scholar
- Verhougstraete M , Reynolds KA , Pearce-Walker J , Gerba C . Cost-benefit analysis of point-of-use devices for health risks reduction from pathogens in drinking water. J Water Health . 2020 ; 18 : 968 – 82 . DOI PubMed Google Scholar
- Colford JM Jr , Roy S , Beach MJ , Hightower A , Shaw SE , Wade TJ . A review of household drinking water intervention trials and an approach to the estimation of endemic waterborne gastroenteritis in the United States. J Water Health . 2006 ; 4 ( Suppl 2 ): 71 – 88 . DOI PubMed Google Scholar
- DeFlorio-Barker S , Wing C , Jones RM , Dorevitch S . Estimate of incidence and cost of recreational waterborne illness on United States surface waters. Environ Health . 2018 ; 17 : 3 . DOI PubMed Google Scholar
- Dorevitch S , Pratap P , Wroblewski M , Hryhorczuk DO , Li H , Liu LC , et al. Health risks of limited-contact water recreation. Environ Health Perspect . 2012 ; 120 : 192 – 7 . DOI PubMed Google Scholar
- Beshearse E , Bruce BB , Nane GF , Cooke RM , Aspinall W , Hald T , et al. Attribution of illnesses transmitted by food and water to comprehensive transmission pathways using structured expert judgment, United States. Emerg Infect Dis . 2021 ; 27 : 182 – 95 . DOI PubMed Google Scholar
- van Asperen IA , de Rover CM , Schijven JF , Oetomo SB , Schellekens JF , van Leeuwen NJ , et al. Risk of otitis externa after swimming in recreational fresh water lakes containing Pseudomonas aeruginosa. BMJ . 1995 ; 311 : 1407 – 10 . DOI PubMed Google Scholar
- Hajjartabar M . Poor-quality water in swimming pools associated with a substantial risk of otitis externa due to Pseudomonas aeruginosa. Water Sci Technol . 2004 ; 50 : 63 – 7 . DOI PubMed Google Scholar
- Wade TJ , Sams EA , Beach MJ , Collier SA , Dufour AP . The incidence and health burden of earaches attributable to recreational swimming in natural waters: a prospective cohort study. Environ Health . 2013 ; 12 : 67 . DOI PubMed Google Scholar
- Calderon R , Mood EW . A epidemiological assessment of water quality and “swimmer’s ear”. Arch Environ Health . 1982 ; 37 : 300 – 5 . DOI PubMed Google Scholar
- Centers for Disease Control and Prevention . Model Aquatic Health Code, 3rd edition. 2018 [ cited 2022 Dec 20 ]. https://www.cdc.gov/mahc/editions/previous.html
- Hlavsa MC , Aluko SK , Miller AD , Person J , Gerdes ME , Lee S , et al. Outbreaks associated with treated recreational water—United States, 2015–2019. MMWR Morb Mortal Wkly Rep . 2021 ; 70 : 733 – 8 . DOI PubMed Google Scholar
- Centers for Disease Control and Prevention . Healthy swimming. April 28, 2022 [ cited 2022 May 16 ]. https://www.cdc.gov/healthywater/swimming/index.html
- Hlavsa MC , Cikesh BL , Roberts VA , Kahler AM , Vigar M , Hilborn ED , et al. Outbreaks associated with treated recreational water—United States, 2000–2014. MMWR Morb Mortal Wkly Rep . 2018 ; 67 : 547 – 51 . DOI PubMed Google Scholar
- Centers for Disease Control and Prevention (CDC) . Estimated burden of acute otitis externa—United States, 2003-2007. MMWR Morb Mortal Wkly Rep . 2011 ; 60 : 605 – 9 . PubMed Google Scholar
- Centers for Disease Control and Prevention . Healthcare-associated infections (HAIs). August 12, 2019 [ cited 2022 Jun 16 ]. https://www.cdc.gov/hai/organisms/ntm/health-departments.html
- Shih DC , Cassidy PM , Perkins KM , Crist MB , Cieslak PR , Leman RL . Extrapulmonary nontuberculous mycobacterial disease surveillance—Oregon, 2014–2016. MMWR Morb Mortal Wkly Rep . 2018 ; 67 : 854 – 7 . DOI PubMed Google Scholar
- Grigg C , Jackson KA , Barter D , Czaja CA , Johnston H , Lynfield R , et al. Epidemiology of pulmonary and extrapulmonary nontuberculous mycobacteria infections at 4 US emerging infections program sites: a six-month pilot. Clin Infect Dis. 2023 Apr 21 [Epub ahead of print]. PubMed Google Scholar
- American Society of Heating, Refrigerating and Air-Conditioning Engineers . ANSI/ASHRAE standard 188–2018, legionellosis: risk management for building water systems. Peachtree Corners (Georgia, USA) . Society . 2018 .
- Centers for Disease Control and Prevention . Healthcare-associated infections. Reduce risk from water. September 11, 2019 [ cited 2022 Nov 29 ]. https://www.cdc.gov/hai/prevent/environment/water.html
- Clopper BR , Kunz JM , Salandy SW , Smith JC , Hubbard BC , Sarisky JP . A methodology for classifying root causes of outbreaks of Legionnaires’ disease: deficiencies in environmental control and water management. Microorganisms . 2021 ; 9 : 89 . DOI PubMed Google Scholar
- Centers for Disease Control and Prevention . Safe water program. 2022 [ cited 2022 Sep 13 ]. https://www.cdc.gov/nceh/ehs/water/index.html
- Centers for Disease Control and Prevention . Developing a water management program to reduce Legionella growth and spread in buildings. A practical guide to implementing industry standards. June 24, 2021 [ cited 2022 May 19 ]. https://www.cdc.gov/legionella/downloads/toolkit.pdf
- Centers for Disease Control and Prevention . Toolkit for controlling Legionella in common sources of exposure. 2021 [ cited 2022 Dec 3 ]. https://www.cdc.gov/legionella/wmp/control-toolkit/index.html
- Centers for Disease Control and Prevention . Reduce risk from water, from plumbing to patients [ cited 2023 Mar 16 ]. https://www.cdc.gov/hai/prevent/environment/water.html
- Kanamori H , Weber DJ , Rutala WA . Healthcare outbreaks associated with a water reservoir and infection prevention strategies. Clin Infect Dis . 2016 ; 62 : 1423 – 35 . DOI PubMed Google Scholar
- Gamage SD , Ambrose M , Kralovic SM , Roselle GA . Water safety and health care: preventing infections caused by opportunistic premise plumbing pathogens. Infect Dis Clin North Am . 2021 ; 35 : 667 – 95 . DOI PubMed Google Scholar
- Decker BK , Palmore TN . The role of water in healthcare-associated infections. Curr Opin Infect Dis . 2013 ; 26 : 345 – 51 . DOI PubMed Google Scholar
- Williams MM , Armbruster CR , Arduino MJ . Plumbing of hospital premises is a reservoir for opportunistically pathogenic microorganisms: a review. Biofouling . 2013 ; 29 : 147 – 62 . DOI PubMed Google Scholar
- Donohue MJ , Vesper S , Mistry J , Donohue JM . Impact of chlorine and chloramine on the detection and quantification of Legionella pneumophila and Mycobacterium species. Appl Environ Microbiol . 2019 ; 85 : e01942 – 19 . DOI PubMed Google Scholar
- Loret JF , Dumoutier N . Non-tuberculous mycobacteria in drinking water systems: A review of prevalence data and control means. Int J Hyg Environ Health . 2019 ; 222 : 628 – 34 . DOI PubMed Google Scholar
- Centers for Disease Control and Prevention . Preventing waterborne germs at home. February 11, 2022 [ cited 2022 May 19 ]. https://www.cdc.gov/healthywater/drinking/preventing-waterborne-germs-at-home.html
- DeFlorio-Barker S , Wade TJ , Jones RM , Friedman LS , Wing C , Dorevitch S . Estimated costs of sporadic gastrointestinal illness associated with surface water recreation: a combined analysis of data from NEEAR and CHEERS studies. Environ Health Perspect . 2017 ; 125 : 215 – 22 . DOI PubMed Google Scholar
- Greco SL , Drudge C , Fernandes R , Kim J , Copes R . Estimates of healthcare utilisation and deaths from waterborne pathogen exposure in Ontario, Canada. Epidemiol Infect . 2020 ; 148 : e70 . DOI PubMed Google Scholar
- Figure . Direct healthcare costs for emergency department visits and hospitalizations in study estimating waterborne infectious disease burden by exposure route, United States, 2014. Estimated combined costs are shown in US...
- Table 1 . Number of cases of selected domestically acquired illnesses from different water sources in study estimating waterborne infectious disease burden by exposure route, United States, 2014
- Table 2 . Number of emergency department visits for selected domestically acquired illnesses from different water sources in study estimating waterborne infectious disease burden by exposure route, United States, 2014
- Table 3 . Number of hospitalizations for selected domestically acquired illnesses from different water sources in study estimating waterborne infectious disease burden by exposure route, United States, 2014
- Table 4 . Number of deaths from selected illnesses domestically acquired from different water sources in study estimating waterborne infectious disease burden by exposure route, United States, 2014
DOI: 10.3201/eid2907.230231
1 These first authors contributed equally to this article.
Table of Contents – Volume 29, Number 7—July 2023
EID Search Options |
---|
– Search articles by author and/or keyword. |
– Search articles by the topic country. |
– Search articles by article type and issue. |
Please use the form below to submit correspondence to the authors or contact them at the following address:
Megan E. Gerdes, Centers for Disease Control and Prevention, 1600 Clifton Rd NE, Mailstop H24-9, Atlanta, GA 30329-4027, USA
Comment submitted successfully, thank you for your feedback.
There was an unexpected error. Message not sent.
Article Citations
Highlight and copy the desired format.
EID | Gerdes ME, Miko S, Kunz JM, Hannapel EJ, Hlavsa MC, Hughes MJ, et al. Estimating Waterborne Infectious Disease Burden by Exposure Route, United States, 2014. Emerg Infect Dis. 2023;29(7):1357-1366. https://doi.org/10.3201/eid2907.230231 |
---|---|
AMA | Gerdes ME, Miko S, Kunz JM, et al. Estimating Waterborne Infectious Disease Burden by Exposure Route, United States, 2014. . 2023;29(7):1357-1366. doi:10.3201/eid2907.230231. |
APA | Gerdes, M. E., Miko, S., Kunz, J. M., Hannapel, E. J., Hlavsa, M. C., Hughes, M. J....Collier, S. A. (2023). Estimating Waterborne Infectious Disease Burden by Exposure Route, United States, 2014. , (7), 1357-1366. https://doi.org/10.3201/eid2907.230231. |
Metric Details
Article views: 5781.
Data is collected weekly and does not include downloads and attachments. View data is from .
What is the Altmetric Attention Score?
The Altmetric Attention Score for a research output provides an indicator of the amount of attention that it has received. The score is derived from an automated algorithm, and represents a weighted count of the amount of attention Altmetric picked up for a research output.
EDITORIAL article
Editorial: impact and control of food- and waterborne protozoan parasites.
- 1 Global Health and Tropical Medicine, Instituto de Higiene e Medicina Tropical, Universidade Nova de Lisboa, Lisboa, Portugal
- 2 Environmental Health Institute, Faculdade de Medicina, Universidade de Lisboa, Lisboa, Portugal
- 3 Department of Basic and Clinical Sciences, University of Nicosia School of Medicine, Medical School, Nicosia, Cyprus
- 4 Université de Rouen Normandie, Laboratory of Parasitology and Mycology, UR7510 ESCAPE, University Hospital of Rouen, Rouen, France
Editorial on the Research Topic Impact and control of food- and waterborne protozoan parasites
Protozoan parasites: the invisible menace
The rise of food—and waterborne diseases caused by protozoan parasites, including Cryptosporidium, Cyclospora, Giardia , and Toxoplasma gondii , increasingly threatens food safety.
Cyclospora is a causative agent of gastrointestinal outbreaks, primarily associated with fresh produce such as soft fruits and leafy vegetables. Also, the contamination of vegetables and fruits with Cryptosporidium has been reported in many countries worldwide. In addition, while infection with Toxoplasma gondii is usually mild or asymptomatic, this can present a serious health problem in immunocompromised persons and infections in pregnant women can present a severe risk to the baby. T. gondii can be transmitted to humans by consuming water and/or fresh vegetables and fruit contaminated with oocysts and raw or undercooked meat infected with tissue cysts. These parasites pose a significant public health hazard, demanding urgent attention from the scientific community and the food industry. A comprehensive, multifaceted approach is essential to safeguard our food supply and protect vulnerable populations.
In their paper titled “ Prevalence and risk factors associated with gastrointestinal parasites in goats (Capra hircus) and sheep (Ovis aries) from three provinces (Jiangsu, Shaanxi and Hunan) of China ,” Cai et al. discuss significant shifts in food consumption patterns in China. One notable change is the consumers' growing popularity of goats and sheep due to their low fat and cholesterol content, high protein, and appealing flavor. Gastrointestinal (GI) parasites infecting goats and sheep include helminths and protozoa, among which nematodes and coccidia are the most common.
Although the species Cryptosporidium, Giardia , and Toxoplasma were not investigated in the study, it was shown that the protozoan Eimeria was detected in a large majority of the samples ( n = 1,081), accounting for 71.0% (767/1,081). The prevalence of helminths was 56.2% (607/1,081). The dominant species were E. alijevi in goats (67.3%, 562/835) and E. parva in sheep (30.1%, 74/246). The ground feeding mode, autumn season, and regions were relevant risk factors that significantly influenced the occurrence of GI parasites in goats and sheep. Investigating these GI parasites is pivotal, as it contributes to developing prevention strategies to minimize economic losses in small ruminant production and mitigate zoonotic parasite infections in humans.
Detection and prevention: a scientific imperative
There is a critical need for rapid and standardized detection methods to address the growing threat of protozoan parasites in our food supply. Advances in molecular biology and diagnostic technologies offer promising avenues for more accurate and timely identification of these pathogens. Research into the parasitic load and prevalence in various foodstuffs, including fresh produce, meat, fish, and shellfish, is vital for understanding the scope of contamination and developing targeted interventions.
In their study titled “ First application of a droplet digital PCR to detect Toxoplasma gondii in mussels ”, Mancusi et al. applied and validated a droplet digital polymerase chain reaction (ddPCR) protocol on mussels to obtain a more sensitive diagnostic tool to detect and quantify T. gondii DNA. Bivalve molluscs, such as clams, cockles, mussels, and oysters, can filter significant quantities of water, thereby accumulating chemical and biological contaminants, including T. gondii oocysts. Furthermore, these molluscs can excrete the parasite in their feces several days after ingestion. Consequently, consuming raw or undercooked bivalve molluscs represents a substantial risk to human health. The ddPCR targeting the 529 bp repeated element gene of T. gondii demonstrated efficient DNA amplification of up to 8 genomic copies/μL. Analysis of the ddPCR data revealed distinct segregation between negative and positive droplets with minimal interface droplets, thus underscoring the technique's elevated specificity and efficacy. The ddPCR exhibited 100% sensitivity and specificity (95% confidence interval = 94.3–99.9). This study reported no significant intra-laboratory variance in results. The ddPCR holds promise for prompt, sensitive detection of low DNA concentrations of T. gondii , thus rendering it suitable for standardized food inspection across diverse matrices and mitigating the public health risks associated with this parasite.
In humans, the first-line point-of-care (POC) commercial tests for Toxoplasma are designated as Toxoplasma ICT IgG-IgM. These tests integrate the tachyzoites total lysate antigen (TLA) derived from mouse proliferation or in vitro tissue cultures. Although these tests provide 100% sensitivity and specificity in the USA, they have shown different sensitivity and specificity rates of 97 and 96%, respectively, in France, as per Chapey et al. (2017) . Many efforts have been made to replace TLA with recombinant antigens (rAgs) to diagnose toxoplasmosis in humans and animals.
In commercial diagnostic test kits, the detection of IgG demonstrates high specificity and sensitivity, whereas IgM detection exhibits lower sensitivity ( Khan and Noordin, 2020 ). The scientific community is actively involved in identifying novel antigens targeting IgM to enhance early infection diagnostics. This collective effort could pave the way for developing more performant tools. Nguyen et al. , in their paper “ Identification of novel biomarkers for anti-Toxoplasma gondii IgM detection and the potential application in rapid diagnostic fluorescent tests ,” identified three novel antigens—EF1γ, PGKI, and GAP50—that specifically target IgM. Of the three antigens examined, GAP50 exhibited higher sensitivity in detecting IgM in rodent samples when used to fabricate a rapid strip test coupled with a fluorochrome (FITC) compared to TLA-based ELISA. The authors emphasize the distinctive immunoreactivity of GAP50, emphasizing its potential as a specific diagnostic biomarker to augment the sensitivity of FITC in IgM detection. The authors propose its suitability as a candidate antigen for integration into POC testing to detect IgM associated with T. gondii infections in patient samples.
The last study was titled “ Development of a targeted amplicon sequencing method for genotyping Cyclospora cayetanensis from fresh produce and clinical samples with enhanced genomic resolution and sensitivity ”. Leonard et al. addresses the issue of Cyclospora cayetanensis , for which no tools are currently available for genotyping contaminated fresh produce or environmental samples despite the increasing number of cyclosporiasis cases. The Centers for Disease Control and Prevention (CDC) employ eight markers for multi-locus sequencing typing (MLST), which involve individual conventional PCRs for each marker: CDS1, CDS2, CDS3, CDS4, HC378, HC360i2, MSR, and MT-junction. To achieve successful genetic clustering, genotyping data for a sample must be available for at least five markers or the three specific markers—HC378, HC360i2, and MSR, as per Nascimento et al. (2020) . Leonard et al. developed a targeted amplicon sequencing (TAS) assay, combined with a bait-capture technique, to achieve the necessary sensitivity for genotyping C. cayetanensis in contaminated fresh produce samples. This TAS assay targets 52 loci, 49 located in the nuclear genome, and covers 396 currently known single nucleotide polymorphism (SNP) sites. The TAS assay facilitates the haplotyping of more markers, thereby capturing more significant genomic diversity from fecal samples with low parasite loads. It enhances genetic resolution and enables sequencing from artificially contaminated romaine lettuce and salad mix. Even at low contamination levels of 10 oocysts in 25 g of leafy greens, the assay can yield sequences for a minimum of 24 markers. As a molecular surveillance tool, this method may investigate the dispersion of C. cayetanensis in various environments.
A call to action
The ongoing battle against food- and waterborne protozoan parasites necessitates collaboration among researchers, policymakers, and industry stakeholders to develop and implement effective detection, prevention, and treatment methods. As our understanding of these parasites and their impact on food safety improves, it is crucial to translate scientific discoveries into practical solutions that can be readily applied across food production and distribution networks.
In summary, protozoan parasites are increasingly prevalent in our food and water sources, posing significant risks. We can mitigate these pathogenic threats through interdisciplinary cooperation and research investment. The time to act is now before these threats become overwhelming challenges.
Author contributions
OM: Writing – review & editing, Visualization, Validation, Supervision. PK: Writing – review & editing, Visualization, Validation, Supervision. RR: Writing – review & editing, Writing – original draft, Visualization, Validation, Supervision.
The author(s) declare that no financial support was received for the research, authorship, and/or publication of this article.
Acknowledgments
We sincerely thank all the authors and reviewers who participated in this Research Topic.
Conflict of interest
The authors declare that the research was conducted in the absence of any commercial or financial relationships that could be construed as a potential conflict of interest.
Publisher's note
All claims expressed in this article are solely those of the authors and do not necessarily represent those of their affiliated organizations, or those of the publisher, the editors and the reviewers. Any product that may be evaluated in this article, or claim that may be made by its manufacturer, is not guaranteed or endorsed by the publisher.
Chapey, E., Wallon, M., and Peyron, F. (2017). Evaluation of the LDBIO point of care test for the combined detection of toxoplasmic IgG and IgM. Clin. Chim. Acta 464, 200–201. doi: 10.1016/j.cca.2016.10.023
PubMed Abstract | Crossref Full Text | Google Scholar
Khan, A. H., and Noordin, R. (2020). Serological and molecular rapid diagnostic tests for toxoplasma infection in humans and animals. Eur. J. Clin. Microbiol. Infect. Dis . 39, 19–30. doi: 10.1007/s10096-019-03680-2
Nascimento, F. S., Barratt, J., Houghton, K., Plucinski, M., Kelley, J., Casillas, S., et al. (2020). Evaluation of an ensemble-based distance statistic for clustering MLST datasets using epidemiologically defined clusters of cyclosporiasis. Epidemiol. Infect . 148:e172. doi: 10.1017/S0950268820001697
Keywords: foodborne pathogens, protozoa, foodborne parasites, waterborne, contamination
Citation: Matos O, Karanis P and Razakandrainibe R (2024) Editorial: Impact and control of food- and waterborne protozoan parasites. Front. Microbiol. 15:1466883. doi: 10.3389/fmicb.2024.1466883
Received: 18 July 2024; Accepted: 24 July 2024; Published: 06 August 2024.
Edited and reviewed by: Aldo Corsetti , University of Teramo, Italy
Copyright © 2024 Matos, Karanis and Razakandrainibe. This is an open-access article distributed under the terms of the Creative Commons Attribution License (CC BY) . The use, distribution or reproduction in other forums is permitted, provided the original author(s) and the copyright owner(s) are credited and that the original publication in this journal is cited, in accordance with accepted academic practice. No use, distribution or reproduction is permitted which does not comply with these terms.
*Correspondence: Romy Razakandrainibe, romy.razakandrainibe@univ-rouen.fr
Disclaimer: All claims expressed in this article are solely those of the authors and do not necessarily represent those of their affiliated organizations, or those of the publisher, the editors and the reviewers. Any product that may be evaluated in this article or claim that may be made by its manufacturer is not guaranteed or endorsed by the publisher.
Information
- Author Services
Initiatives
You are accessing a machine-readable page. In order to be human-readable, please install an RSS reader.
All articles published by MDPI are made immediately available worldwide under an open access license. No special permission is required to reuse all or part of the article published by MDPI, including figures and tables. For articles published under an open access Creative Common CC BY license, any part of the article may be reused without permission provided that the original article is clearly cited. For more information, please refer to https://www.mdpi.com/openaccess .
Feature papers represent the most advanced research with significant potential for high impact in the field. A Feature Paper should be a substantial original Article that involves several techniques or approaches, provides an outlook for future research directions and describes possible research applications.
Feature papers are submitted upon individual invitation or recommendation by the scientific editors and must receive positive feedback from the reviewers.
Editor’s Choice articles are based on recommendations by the scientific editors of MDPI journals from around the world. Editors select a small number of articles recently published in the journal that they believe will be particularly interesting to readers, or important in the respective research area. The aim is to provide a snapshot of some of the most exciting work published in the various research areas of the journal.
Original Submission Date Received: .
- Active Journals
- Find a Journal
- Proceedings Series
- For Authors
- For Reviewers
- For Editors
- For Librarians
- For Publishers
- For Societies
- For Conference Organizers
- Open Access Policy
- Institutional Open Access Program
- Special Issues Guidelines
- Editorial Process
- Research and Publication Ethics
- Article Processing Charges
- Testimonials
- Preprints.org
- SciProfiles
- Encyclopedia
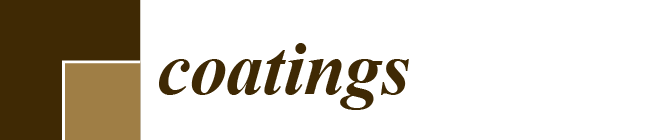
Article Menu
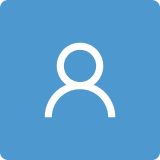
- Subscribe SciFeed
- Recommended Articles
- Google Scholar
- on Google Scholar
- Table of Contents
Find support for a specific problem in the support section of our website.
Please let us know what you think of our products and services.
Visit our dedicated information section to learn more about MDPI.
JSmol Viewer
Optimization of preparation process for chitosan-coated pomelo peel flavonoid microcapsules and its effect on waterborne paint film properties, 1. introduction, 2. materials and methods, 2.1. materials, 2.2. method of preparing microcapsules, 2.3. preparation of waterborne paint films with different contents of microcapsules, 2.4. performance test, 2.4.1. the microcapsule yield and coverage rate, 2.4.2. the morphology and chemical composition, 2.4.3. the antibacterial performance, 2.4.4. the optical performance, 2.4.5. the mechanical performance, 3. results and discussion, 3.1. the microcapsules’ yield and coverage rate, 3.2. the morphological and chemical composition of the microcapsules, 3.3. the morphology of the paint film, 3.4. the chemical composition of the paint film, 3.5. the antibacterial properties of the paint film, 3.6. the optical properties of the paint film, 3.7. the mechanical properties of the paint film, 4. conclusions, author contributions, institutional review board statement, informed consent statement, data availability statement, conflicts of interest.
- Hu, J.; Liu, Y.; Xu, W. Impact of cellular structure on the thickness and light reflection properties of structural color layers on diverse wood surfaces. Wood Mater. Sci. Eng. 2024 . [ Google Scholar ] [ CrossRef ]
- Wu, S.S.; Zhou, J.C.; Xu, W. A convenient approach to manufacturing lightweight and high-sound-insulation plywood using furfuryl alcohol/multilayer graphene oxide as a shielding layer. Wood Mater. Sci. Eng. 2024 . [ Google Scholar ] [ CrossRef ]
- Hu, W.; Yu, R. Study on the strength mechanism of the wooden round-end mortise-and-tenon joint using the digital image correlation method. Holzforschung 2024 . [ Google Scholar ] [ CrossRef ]
- Wang, C.; Zhang, C.Y.; Zhu, Y. Reverse design and additive manufacturing of furniture protective foot covers. BioResources 2024 , 19 , 4670–4678. [ Google Scholar ] [ CrossRef ]
- Wang, C.; Yu, J.H.; Jiang, M.H.; Li, J.Y. Effect of selective enhancement on the bending performance of fused deposition methods 3D-printed PLA models. BioResources 2024 , 19 , 2660–2669. [ Google Scholar ] [ CrossRef ]
- Zhang, N.; Xu, W.; Tan, Y. Multi-attribute hierarchical clustering for product family division of customized wooden doors. Bioresources 2023 , 18 , 7889–7904. [ Google Scholar ] [ CrossRef ]
- Hu, J.; Liu, Y.; Xu, W. Influence of cell characteristics on the construction of structural color layers on wood surfaces. Forests 2024 , 15 , 676. [ Google Scholar ] [ CrossRef ]
- Mai, C.; Schmitt, U.; Niemz, P. A brief overview on the development of wood research. Holzforschung 2022 , 76 , 102–119. [ Google Scholar ] [ CrossRef ]
- Xue, J.X.; Xu, W.; Zhou, J.C.; Mao, W.G.; Wu, S.S. Effects of High-Temperature Heat Treatment Modification by Impregnation on Physical And Mechanical Properties of Poplar. Materials 2022 , 15 , 7334. [ Google Scholar ] [ CrossRef ]
- Weng, M.Y.; Zhu, Y.T.; Mao, W.G.; Zhou, J.C.; Xu, W. Nano-Silica/Urea-Formaldehyde Resin-Modified Fast-Growing Lumber Performance Study. Forests 2023 , 14 , 1440. [ Google Scholar ] [ CrossRef ]
- Wu, S.S.; Tao, X.; Xu, W. Thermal Conductivity of Poplar Wood Veneer Impregnated with Graphene/Polyvinyl Alcohol. Forests 2021 , 12 , 777. [ Google Scholar ] [ CrossRef ]
- Liu, Q.Q.; Gao, D.; Xu, W. Effect of paint process on the performance of modified poplar wood antique. Coatings 2021 , 11 , 1174. [ Google Scholar ] [ CrossRef ]
- Xu, W.; Chen, P.; Yang, Y.; Wang, X.; Liu, X. Effects of freezing and steam treatments on the permeability of populus tomentosa. Materialwiss. Werkst. 2021 , 52 , 907–915. [ Google Scholar ] [ CrossRef ]
- Tao, M.X.; Liu, X.; Xu, W. Effect of the vacuum impregnation process on water absorption and nail-holding power of silica sol-modified Chinese Fir. Forests 2024 , 15 , 261. [ Google Scholar ] [ CrossRef ]
- Singh, A.P.; Kim, Y.S.; Chavan, R.R. Relationship of wood cell wall ultrastructure to bacterial degradation of wood. IAWA J. 2019 , 40 , 845–870. [ Google Scholar ] [ CrossRef ]
- Xu, W.; Fang, X.Y.; Han, J.T.; Wu, Z.H. Effect of Coating Thickness on Sound Absorption Property of Four Wood Species Commonly Used for Piano Soundboards. Wood Fiber Sci. 2020 , 52 , 28–43. [ Google Scholar ] [ CrossRef ]
- Zhang, H.Q.; Feng, X.H.; Wu, Y.; Wu, Z.H. Factors influencing the properties of UV-cured self-matting film. Prog. Org. Coat. 2024 , 189 , 108241. [ Google Scholar ] [ CrossRef ]
- Zhang, H.Q.; Feng, X.H.; Wu, Y.; Wu, Z.H. Self-matting waterborne polyurethane acrylate wood coating by 222 nm far-UVC irradiation in ambient air. Prog. Org. Coat. 2024 , 189 , 108305. [ Google Scholar ] [ CrossRef ]
- Liu, Q.Q.; Gao, D.; Xu, W. Effect of sanding processes on the surface properties of modified poplar coated by primer compared with mahogany. Coatings 2020 , 10 , 856. [ Google Scholar ] [ CrossRef ]
- Liu, Q.Q.; Gao, D.; Xu, W. Effect of polyurethane non-transparent coating process on paint film performance applied on modified poplar. Coatings 2022 , 12 , 39. [ Google Scholar ] [ CrossRef ]
- Jiang, G.F.; Li, X.F.; Che, Y.L.; Lv, Y.; Liu, F.; Wang, Y.Q.; Zhao, C.C.; Wang, X.J. Antibacterial and anticorrosive properties of CuZnO@RGO waterborne polyurethane coating in circulating cooling water. Environ. Sci. Pollut. R. 2019 , 26 , 9027–9040. [ Google Scholar ] [ CrossRef ] [ PubMed ]
- Wang, L.; Wang, T.; Hao, R.D.; Wang, Y.M. Construction strategy and mechanism of a novel wood preservative with excellent antifungal effects. Molecules 2024 , 29 , 1313. [ Google Scholar ] [ CrossRef ] [ PubMed ]
- Vainio-Kaila, T.; Harju, A.; Rohumaa, A.; Paajanen, O.; Venäläinen, M.; Seppä, J.; Veijalainen, A.M.; Pasanen, P. The effect of surface treatment on the antibacterial properties of wood and the possibility to detect the antibacteriality with fluorescence method. Forests 2023 , 14 , 23. [ Google Scholar ] [ CrossRef ]
- Chang, Y.J.; Wu, Z.H. Synthesized high performance UV-cured wood wax oil using Irgacure 2959 modified thistle oil and linseed oil. Ind. Crop. Prod. 2024 , 218 , 118952. [ Google Scholar ] [ CrossRef ]
- Veiko, A.G.; Olchowik-Grabarek, E.; Sekowski, S.; Roszkowska, A.; Lapshina, E.A.; Dobrzynska, I.; Zamaraeva, M.; Zavodnik, I.B. Antimicrobial activity of quercetin, naringenin and catechin: Flavonoids inhibit staphylococcus aureus-induced hemolysis and modify membranes of bacteria and erythrocytes. Molecules 2023 , 28 , 1252. [ Google Scholar ] [ CrossRef ] [ PubMed ]
- Wang, L.; Li, W.J.; Li, X.Y.; Liu, J.C.; Chen, Y. Antimicrobial activity and mechanisms of walnut green husk extract. Molecules 2023 , 28 , 7981. [ Google Scholar ] [ CrossRef ]
- Tran, N.D.N.; Bui, T.H.; Nguyen, A.P.; Nguyen, T.T.; Nguyen, V.M.; Duong, N.L.; Nguyen, T. The ability of silver-biochar green-synthesized from Citrus maxima peel to adsorb pollutant organic compounds and antibacterial activity. Green Chem. Lett. Rev. 2022 , 15 , 16–25. [ Google Scholar ] [ CrossRef ]
- Irkni, R.; Esmer, O.K. Novel food packaging systems with natural antimicrobial agents. J. Food Sci. Tech. Mys. 2015 , 52 , 6095–6111. [ Google Scholar ] [ CrossRef ]
- Van, C.K.; Nguyen, P.T.N.; Nguyen, T.T.T.; Bach, L.G. Microencapsulation of Citrus latifolia peel essential oil by spray-drying using maltodextrin: Characterization, antimicrobial activities, and release profile. Lwt-Food Sci. Technol. 2024 , 197 , 115825. [ Google Scholar ] [ CrossRef ]
- Zhang, Q.Y.; Yang, A.; Tan, W.H.; Yang, W.C. Development, Physicochemical Properties, and Antibacterial Activity of Propolis Microcapsules. Foods 2023 , 12 , 3191. [ Google Scholar ] [ CrossRef ]
- Huang, N.; Yan, X.X. Preparation of aloe-emodin microcapsules and its effect on antibacterial and optical properties of water-based coating. Polymers 2023 , 15 , 1728. [ Google Scholar ] [ CrossRef ] [ PubMed ]
- Zhang, Z.; Zhang, S.S.; Su, R.R.; Xiong, D.; Feng, W.; Chen, J.P. Controlled release mechanism and antibacterial effect of layer-by-layer self-assembly thyme oil microcapsule. J. Food Sci. 2019 , 84 , 1427–1438. [ Google Scholar ] [ CrossRef ] [ PubMed ]
- Shilpa, V.S.; Shams, R.; Dash, K.K.; Pandey, V.K.; Dar, A.H.; Mukarram, S.A.; Harsányi, E.; Kovács, B. Phytochemical properties, extraction, and pharmacological benefits of naringin: A review. Molecules 2023 , 28 , 5623. [ Google Scholar ] [ CrossRef ] [ PubMed ]
- Chen, S.; Wang, X.J.; Cheng, Y.; Gao, H.S.; Chen, X.H. A review of classification, biosynthesis, biological activities and potential applications of flavonoids. Molecules 2023 , 28 , 4982. [ Google Scholar ] [ CrossRef ] [ PubMed ]
- Shangguan, Y.C.; Ni, J.; Jiang, L.L.; Hu, Y.; He, C.B.; Ma, Y.; Wu, G.H.; Xiong, H.J. Response surface methodology-optimized extraction of flavonoids from pomelo peels and isolation of naringin with antioxidant activities by Sephadex LH20 gel chromatography. Cur. Res. Food Sci. 2023 , 7 , 100610. [ Google Scholar ] [ CrossRef ] [ PubMed ]
- Lopes, L.A.A.; Rodrigues, J.B.D.; Magnani, M.; de Souza, E.L.; de Siqueira, J.P. Inhibitory effects of flavonoids on biofilm formation by Staphylococcus aureus that overexpresses efflux protein genes. Microb. Pathog. 2017 , 107 , 193–197. [ Google Scholar ] [ CrossRef ] [ PubMed ]
- Liu, Z.Y.; Pan, Y.R.; Li, X.S.; Jie, J.X.; Zeng, M.Y. Chemical composition, antimicrobial and anti-quorum sensing activities of pummelo peel flavonoid extract. Ind. Crop. Prod. 2017 , 109 , 862–868. [ Google Scholar ] [ CrossRef ]
- Valle, J.A.B.; Valle, R.D.S.C.; Bierhalz, A.C.K.; Bezerra, F.M.; Hernandez, A.L.; Arias, M.J.L. Chitosan microcapsules: Methods of the production and use in the textile finishing. J. Appl. Polym. Sci. 2021 , 138 , e50482. [ Google Scholar ] [ CrossRef ]
- Zhou, J.C.; Xu, W. An aesthetic transparent wood resistant to Escherichia coli based on interface optimization. Eur. J. Wood Wood Prod. 2023 , 81 , 1569–1579. [ Google Scholar ] [ CrossRef ]
- Chen, K.L.; Ni, Y.Z.; Shi, X.; Jia, Z.H.; Qiu, H.; Portale, G. Green fabrication of chitosan microcapsules via double emulsion-simple coacervation and their application in fabrics. Cellulose 2023 , 30 , 11861–11873. [ Google Scholar ] [ CrossRef ]
- Utami, P.D.; Setianingsih, H.; Tirto Sari, D.R. Microencapsulation, Physicochemical Characterization, and Antioxidant, Antibacterial, and Antiplasmodial Activities of Holothuria atra Microcapsule. Scientifica 2024 , 2024 , 5559133. [ Google Scholar ] [ CrossRef ] [ PubMed ]
- Comini, S.; Mandras, N.; Iannantuoni, M.R.; Menotti, F.; Musumeci, A.G.; Piersigilli, G.; Allizond, V.; Banche, G.; Cuffini, A.M. Positive and Negative Ions Potently Inhibit the Viability of Airborne Gram-Positive and Gram-Negative Bacteria. Microbiol. Spectr. 2021 , 9 , e00651. [ Google Scholar ] [ CrossRef ] [ PubMed ]
- Monteiro, A.; Cardoso, J.; Guerra, N.; Ribeiro, E.; Viegas, C.; Verde, S.C.; Sousa-Uva, A. Exposure and Health Effects of Bacteria in Healthcare Units: An Overview. Appl. Sci.-Basel 2022 , 12 , 1958. [ Google Scholar ] [ CrossRef ]
- Tehrani, E.; Amiri, S. Synthesis and characterization PVA electro-spun nanofibers containing encapsulated vitamin C in chitosan microspheres. J. Text. I. 2022 , 113 , 212–223. [ Google Scholar ] [ CrossRef ]
- Deng, J.Z.; Ding, T.T.; Yan, X.X. Effect of Two Pomelo Peel Flavonoid Microcapsules on the Performance of Waterborne Coatings on the Surface of Poplar Boards. Coatings 2024 , 14 , 937. [ Google Scholar ] [ CrossRef ]
- GB/T 21866-2008 ; Test Method and Effect for Antibacterial Capability of Paints Film. Standardization Administration of the People’s Republic of China: Beijing, China, 2008.
- GB/T 4789.2-2022 ; National Food Safety Standard Food Microbiological Examination: Aerobic Plate Count. Standardization Administration of the People’s Republic of China: Beijing, China, 2022.
- GB/T 11186.3-1989 ; Methods for Measuring the Colour of Paint Films. Part III: Calculation of Colour Differences. Standardization Administration of the People’s Republic of China: Beijing, China, 1990.
- GB/T 4893.6-2013 ; Test of Surface Coatings of Furniture. Standardization Administration of the People’s Republic of China: Beijing, China, 2013.
- Zhang, H.; Zhou, L.M.; Shehzad, H.; Farooqi, Z.H.; Sharif, A.; Ahmed, E.; Habiba, U.; Qaisar, F.; Fatima, N.-E.; Begum, R.; et al. Innovative free radical induced synthesis of WO 3 -doped diethyl malonate grafted chitosan encapsulated with phosphorylated alginate matrix for UO 2 2+ adsorption: Parameters optimisation through response surface methodology. Sep. Purif. Technol. 2024 , 353 , 128455. [ Google Scholar ] [ CrossRef ]
- Ding, T.T.; Yan, X.X. Preparation process optimization for melamine resin-covered pomelo peel flavonoid antibacterial microcapsules and their effect on waterborne paint film performance. Coatings 2024 , 14 , 654. [ Google Scholar ] [ CrossRef ]
Click here to enlarge figure
Material | Molecular Formula | CAS No. |
---|---|---|
Chitosan | (C H NO ) | 9012-76-4 |
Acetic acid | CH COOH | 64-19-7 |
Tween-80 | C H O | 9005-65-6 |
Sodium tripolyphosphate | Na P O | 7758-29-4 |
Anhydrous ethanol | C H O | 64-17-5 |
Staphylococcus aureus | - | - |
Escherichia coli | - | - |
Nutrient agar medium | - | - |
Nutritious broth | - | - |
Equipment | Model | Manufacturing |
---|---|---|
Optical microscope (OM) | AX10 | Carl Zeiss AG. Oberkochen, Germany |
Scanning electron microscope (SEM) | Quanta-200 | Thermo Fisher Scientific, Waltham, MA, USA |
Magnetic stirrer | DF-101Z | Nanbei Scientific Instrument Technology Co., Ltd., Beijing, China |
Fourier Transform Infrared (FTIR) spectrometer | VERTEX 80V | Bruker Corporation, Karlsruhe, Germany |
Humidity chamber | HWS-50 | Shanghai Shangyi Instrument Equipment Co., Ltd., Shanghai, China |
Freeze-dryer | YTLG-10A | Shanghai Yetuo Technology Co., Ltd., Shanghai, China |
Colony counter | XK97-A | Hangzhou Qiwei Instrument Co., Ltd., Hangzhou, China |
Portable colorimeter | SC-10 | Zhuhai Tianchuang Instrument Co., Ltd., Zhuhai, China |
Gloss meter | HG268 | Shenzhen ThreeNH Technology Co., Ltd., Shenzhen, China |
Ultraviolet spectrophotometer | U-3900 | Hitachi Scientific Instruments (Suzhou) Co., Ltd., Suzhou, China |
Universal mechanical testing machine | AGS-X | Shimadzu Manufacturing Co., Ltd., Kyoto, Japan |
Fine roughness tester | JB-4C | Suliang Instrument Technology Co., Ltd., Suzhou, China |
Level | Factor A pH Value | Factor B m(Core Material):m(Wall Material) | Factor C Concentration of Emulsifier (%) |
---|---|---|---|
1 | 6 | 1.0:1 | 1 |
2 | 8 | 1.2:1 | 3 |
Sample | pH | m(Core Material):m(Wall Material) | Concentration of Emulsifier (%) |
---|---|---|---|
1 | 6 | 1.0:1 | 1 |
2 | 6 | 1.2:1 | 3 |
3 | 8 | 1.0:1 | 3 |
4 | 8 | 1.2:1 | 1 |
Test | Sample | Chitosan (g) | 1% Acetic Acid Solution (g) | Pomelo Peel Flavonoids (g) | Anhydrous Ethanol (g) | Emulsifier (g) | Deionized Water (mL) | STPP (g) |
---|---|---|---|---|---|---|---|---|
Orthogonal test | 1 | 0.80 | 39.20 | 0.80 | 7.20 | 0.72 | 71.28 | 0.80 |
2 | 0.80 | 39.20 | 0.96 | 8.64 | 2.11 | 68.29 | 0.80 | |
3 | 0.80 | 39.20 | 0.80 | 7.20 | 2.10 | 69.90 | 0.80 | |
4 | 0.80 | 39.20 | 0.96 | 8.64 | 0.70 | 69.70 | 0.80 | |
One-factor test | 5 | 0.80 | 39.20 | 0.80 | 7.20 | 0.72 | 71.28 | 0.80 |
6 | 0.80 | 39.20 | 0.80 | 7.20 | 0.72 | 71.28 | 0.80 | |
7 | 0.80 | 39.20 | 0.80 | 7.20 | 0.72 | 71.28 | 0.80 | |
8 | 0.80 | 39.20 | 0.80 | 7.20 | 0.72 | 71.28 | 0.80 | |
9 | 0.80 | 39.20 | 0.80 | 7.20 | 0.72 | 71.28 | 0.80 |
Content of Microcapsules (%) | Mass of Microcapsules (g) | Mass of Waterborne Paint (g) |
---|---|---|
0 | 0 | 1.00 |
3.0 | 0.03 | 0.97 |
6.0 | 0.06 | 0.94 |
9.0 | 0.09 | 0.91 |
12.0 | 0.12 | 0.88 |
15.0 | 0.15 | 0.85 |
Category | Sample | Factor A pH Value | Factor B m(Core Material):m(Wall Material) | Factor C Concentration of Emulsifier (%) | Yield (%) |
---|---|---|---|---|---|
Range | 1 | 6 | 1.0:1 | 1 | 20 |
2 | 6 | 1.2:1 | 3 | 20 | |
3 | 8 | 1.0:1 | 3 | 22 | |
4 | 8 | 1.2:1 | 1 | 21 | |
Mean value 1 | 20.000 | 21.000 | 20.500 | ||
Mean value 2 | 21.500 | 20.500 | 21.000 | ||
R | 1.500 | 0.500 | 0.500 | ||
Factor primary and secondary levels | A > B = C | ||||
Optimal level | A2 | B1 | C2 | ||
Optimal solution | A2 B1 C2 | ||||
Variance | Sum of squared deviations | 2.250 | 0.250 | 0.250 | |
Degree of freedom | 1 | 1 | 1 | ||
F | 2.455 | 0.273 | 0.273 | ||
F | 10.100 | 10.100 | 10.100 | ||
Significance |
Category | Sample | Factor A pH Value | Factor B m(Core Material):m(Wall Material) | Factor C Concentration of Emulsifier (%) | Coverage Rate (%) |
---|---|---|---|---|---|
Range | 1 | 6 | 1.0:1 | 1 | 45 |
2 | 6 | 1.2:1 | 3 | 31 | |
3 | 8 | 1.0:1 | 3 | 53 | |
4 | 8 | 1.2:1 | 1 | 51 | |
Mean value 1 | 38.000 | 49.000 | 48.000 | ||
Mean value 2 | 52.000 | 41.000 | 42.000 | ||
R | 14.000 | 8.000 | 6.000 | ||
Factor primary and secondary levels | A > B > C | ||||
Optimal level | A2 | B1 | C1 | ||
Optimal solution | A2 B1 C1 | ||||
Variance | Sum of squared deviations | 196.000 | 64.000 | 36.000 | |
Degree of freedom | 1 | 1 | 1 | ||
F | 1.986 | 0.649 | 0.365 | ||
F | 10.100 | 10.100 | 10.100 | ||
Significance |
Sample | pH Value | Yield Rate (%) | Coverage Rate (%) |
---|---|---|---|
5 | 5.5 | 24 | 38 |
6 | 6.5 | 20 | 41 |
7 | 7.5 | 22 | 50 |
8 | 8.5 | 22 | 47 |
9 | 9.5 | 23 | 44 |
Content of Microcapsules (%) | Average Number of Recovered Escherichia coli (CFU·Piece ) | Antibacterial Rate against Escherichia coli (%) | Average Number of Recovered Staphylococcus aureus (CFU·Piece ) | Antibacterial Rate against Staphylococcus aureus (%) |
---|---|---|---|---|
0 | 190 | - | 432 | - |
3.0 | 134 | 29.5 ± 0.6 | 289 | 33.1 ± 0.8 |
6.0 | 102 | 46.3 ± 1.6 | 187 | 56.7 ± 1.5 |
9.0 | 73 | 61.6 ± 0.9 | 126 | 70.8 ± 1.2 |
12.0 | 51 | 73.2 ± 1.4 | 74 | 82.9 ± 2.7 |
15.0 | 32 | 83.2 ± 1.1 | 36 | 91.7 ± 1.7 |
Item | SS | d | MS | F | p-Value | F Crit |
---|---|---|---|---|---|---|
Content of microcapsules | 10,537.29 | 5 | 2107.457 | 243.8058 | 0.00 | 5.050329 |
Antibacterial species | 142.83 | 1 | 142.83 | 16.5236 | 0.009682 | 6.607891 |
Error | 43.22 | 5 | 8.644 | |||
Total | 10,723.34 | 11 |
Content of Microcapsules (%) | L | a | b | ΔE |
---|---|---|---|---|
0 | 81.91 | 1.73 | −2.27 | - |
3.0 | 44.93 | 0.97 | 0.50 | 37.09 |
6.0 | 43.50 | 0.97 | 1.30 | 38.58 |
9.0 | 43.00 | 0.90 | 1.57 | 39.11 |
12.0 | 42.20 | 0.87 | 1.60 | 39.91 |
15.0 | 41.40 | 0.63 | 1.87 | 40.74 |
Content of Microcapsules (%) | 20° (%) | 60° (%) | 85° (%) | Gloss Loss Rate (%) |
---|---|---|---|---|
0 | 6.10 | 17.45 | 31.17 | - |
3.0 | 4.47 | 14.13 | 14.93 | 19.1 |
6.0 | 2.40 | 10.30 | 7.27 | 41.0 |
9.0 | 2.13 | 10.17 | 4.10 | 41.7 |
12.0 | 2.10 | 10.17 | 3.40 | 41.7 |
15.0 | 1.77 | 8.10 | 3.27 | 53.6 |
Content of Microcapsules (%) | Visible Light Transmittance (%) |
---|---|
0 | 89.89 |
3.0 | 93.25 |
6.0 | 90.40 |
9.0 | 90.47 |
12.0 | 87.94 |
15.0 | 85.54 |
Content of Microcapsules (%) | Roughness (%) |
---|---|
0 | 0.27 |
3.0 | 0.46 |
6.0 | 1.46 |
9.0 | 2.60 |
12.0 | 2.65 |
15.0 | 2.70 |
Content of Microcapsules (%) | Elongation at Break (%) |
---|---|
0 | 18.9 |
3.0 | 22.3 |
6.0 | 21.5 |
9.0 | 16.1 |
12.0 | 10.5 |
15.0 | 7.9 |
The statements, opinions and data contained in all publications are solely those of the individual author(s) and contributor(s) and not of MDPI and/or the editor(s). MDPI and/or the editor(s) disclaim responsibility for any injury to people or property resulting from any ideas, methods, instructions or products referred to in the content. |
Share and Cite
Deng, J.; Ding, T.; Yan, X. Optimization of Preparation Process for Chitosan-Coated Pomelo Peel Flavonoid Microcapsules and Its Effect on Waterborne Paint Film Properties. Coatings 2024 , 14 , 1003. https://doi.org/10.3390/coatings14081003
Deng J, Ding T, Yan X. Optimization of Preparation Process for Chitosan-Coated Pomelo Peel Flavonoid Microcapsules and Its Effect on Waterborne Paint Film Properties. Coatings . 2024; 14(8):1003. https://doi.org/10.3390/coatings14081003
Deng, Jinzhe, Tingting Ding, and Xiaoxing Yan. 2024. "Optimization of Preparation Process for Chitosan-Coated Pomelo Peel Flavonoid Microcapsules and Its Effect on Waterborne Paint Film Properties" Coatings 14, no. 8: 1003. https://doi.org/10.3390/coatings14081003
Article Metrics
Article access statistics, further information, mdpi initiatives, follow mdpi.
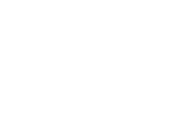
Subscribe to receive issue release notifications and newsletters from MDPI journals

An official website of the United States government
The .gov means it’s official. Federal government websites often end in .gov or .mil. Before sharing sensitive information, make sure you’re on a federal government site.
The site is secure. The https:// ensures that you are connecting to the official website and that any information you provide is encrypted and transmitted securely.
- Publications
- Account settings
Preview improvements coming to the PMC website in October 2024. Learn More or Try it out now .
- Advanced Search
- Journal List
- Elsevier - PMC COVID-19 Collection

Ubiquitous waterborne pathogens
D.n. magana-arachchi.
1 Molecular Microbiology & Human Diseases Unit, National Institute of Fundamental Studies, Kandy, Sri Lanka
R.P. Wanigatunge
2 Department of Plant and Molecular Biology, University of Kelaniya, Kelaniya, Sri Lanka
Water is one of the most important substances on earth and without it life cannot exist. However, poor water quality in many parts of the world has increased the number of water-related diseases, making it the leading cause of disease and death globally for both young and old. Waterborne pathogens cause diseases in humans through two major exposure pathways: drinking water and recreational waters. This chapter on waterborne pathogens will be starting with an introduction, followed by descriptions on classical waterborne pathogens; bacteria, viruses, protozoans, and helminths placing emphasis on the World Health Organization guidelines. Further to conventional waterborne pathogens, fresh organisms and new strains from already known pathogens are being identified and that present important additional challenges to both the water and public health sectors. Hence later part of the chapter focuses on the potential waterborne pathogens and will conclude with a summary of the content.
1. Introduction
In July 2010, the United Nations General Assembly (UNGA) univocally recognized the human right to water and sanitation and acknowledged that clean drinking water and sanitation are essential to the realization of all human rights ( UNGA, 2010 ). However, due to inadequacy, unsafe, inaccessibility, and unaffordability of water, most of the people globally are deprived of this universal right. According to the Joint Monitoring Program (JMP) report, some 3 in 10 people worldwide, or 2.1 billion, lack access to safe, readily available water at home and 6 in 10, or 4.5 billion, lack safely managed sanitation ( WHO and UNICEF, 2017 ). Due to the global efforts, billions of people have gained access to basic drinking water and sanitation services since 2000, but people in many countries are still lacking clean water and proper sanitation in their homes, healthcare facilities, and schools. Hence health of all these people is at a risk, affecting mainly the infants and young children. Water, sanitation, and hygiene were responsible for 829,000 deaths from diarrheal disease in 2016. It is estimated that every year, 361,000 children under 5 years of age die because of diarrhea. In addition, poor sanitation and contaminated water are also linked to transmission of waterborne diseases such as cholera, dysentery, hepatitis A, and typhoid ( WHO and UNICEF, 2017 ).
In September 2015, Member States of the United Nations adopted the 2030 Agenda for Sustainable Development ( UNSD, 2015 ) and Goal 6 of Sustainable Development Goals is to “ Ensure availability and sustainable management of water and sanitation for all .” Targets were set by considering the freshwater cycle as a whole. Member States try to achieve these targets by improving the standard of water, sanitation, and hygiene (WASH) services; increasing treatment, recycling, and reuse of wastewater; improving efficiency and ensuring sustainable withdrawals; and protecting water-related ecosystems as part of an integrated approach to water resources management. They also address the means of implementation for achieving these development outcomes ( WHO and UNICEF, 2017 ).
A pathogen means an agent that causes disease to a host, and waterborne pathogens are the causative agents (usually living organisms) for diseases that are being transmitted through water. Water pollution can occur due to chemical and/or biological contaminants. These waterborne pathogens thrive in polluted waters, especially contaminated with human feces or/and urine. People could get exposed to these microorganisms while drinking water, by eating food prepared with contaminated water, bathing, during recreational activities, or even sometimes in healthcare facilities during dialysis. This exposure could be limited to an individual or it can be a community outbreak. The morbidity and mortality caused by contaminated water are enormous and it could only be controlled by providing microbiologically safe and toxin-free water for drinking, cooking, and other recreational activities.
Surface waters in most countries are polluted with pathogens and this is widely recorded in the developing world. Consumption of these waters leads to waterborne disease outbreaks (WBDOs) ( Patel et al., 2016 ). A recent study from China has shown that potentially pathogenic bacteria were ubiquitous across all of the 16 urban sampled surface waters, and Proteobacteria and Bacteroidetes were the most commonly detected phyla accounting for 21.9%–78.5% and 19.1%–74.7% of sequences, respectively ( Jin et al., 2018 ). Intermittent water supply (IWS) is being practiced throughout low- to middle-income countries. A study was conducted by Bivins et al. (2017) with existing data using reference pathogens Campylobacter , Cryptosporidium , and rotavirus (RV) as conservative risk proxies for infections via bacteria, protozoa, and viruses, respectively. Their findings indicated that the median daily risk of infection is 1 in 23,500 for Campylobacter , 1 in 5,050,000 for Cryptosporidium , and 1 in 118,000 for RV. Based on these risks, IWS may account for 17.2 million infections causing 4.52 million cases of diarrhea, 109,000 diarrheal disability-adjusted life years (DALYs), and 1560 deaths each year. The WHO health-based normative guideline for drinking water is 10 −6 DALYs per person per year and it is likely that the value of diarrheal disease associated with IWS will be surpassing the WHO value.
When ensuring clean water for drinking and other activities, proper management guidelines are needed to be followed. Preparation of these guidelines is not an easy task and it needs thorough understanding about the pathogenic nature of the organisms considering their shape, size, composition, and structure, their survival and behavior, and how they transmit in different waterbodies. Many countries use indicator organisms to assess the microbiological quality of drinking water. Most widely used bacteria are the enteric bacterial coliforms belonging to the family Enterobacteriaceae. In addition to being able to identify the microbial contamination of drinking water with human waste, these organisms are capable of identifying the fecal contaminations in waters used for recreational activities as well as in shellfish production. The presence of these pathogenic organisms in waterbodies is being monitored regularly in most developed countries as new tools are available to them due to the advances made in medical and scientific research. Modern technologies have also been incorporated into the water treatment plants for the effective removal or deactivation of these waterborne pathogens, thereby minimizing the outbreaks and the risk due to exposure.
In this chapter we will be focusing on the ubiquitous waterborne pathogens which cause deadly diseases and outbreaks affecting young and old globally. Etiological agents for substantial amount of waterborne diseases are “classical” waterborne pathogens. However, fresh organisms and new strains from already known pathogens are being identified and that present important additional challenges to both the water and public health sectors. Hence we will outline the potential waterborne pathogens including Helicobacter pylori , Tsukamurella , Cystoisospora belli , and Microsporidia and also Bacillus species and toxic cyanobacteria that needed to be paid attention to supply clean water, prevent mishaps, and protect and improve public health. Listed pathogens for this chapter were selected from the WHO Guidelines for drinking water quality, fourth edition ( 2011 ) and from Global Waterborne Pathogen Project (GWPP) ( Rusinol and Girones, 2017 ). Readers could gain extra knowledge on these organisms by referring to the original articles which are being included in the references. Furthermore, descriptive diagrams of these organisms can be found in the book chapter by Bridle (2013) .
2. Waterborne pathogens
This first section is based on ubiquitous waterborne pathogens including bacteria, viruses, protozoa, and helminths, which will be discussed in chronological order, and the causative diseases and mode of transmission are summarized schematically in Fig. 2.1 for the readers' benefit.

Generalized overview of ubiquitous waterborne pathogens, route of transmission, and sites of infection.
2.1. Waterborne bacteria
Bacterial pathogens are classical etiological agents of waterborne diseases globally. These organisms can occur ubiquitously in many aquatic habitats and humid soils. They are an important part of the biocenosis in various substrates or water systems, especially in their preferred habitats, the biofilms. According to WHO, from the mortality of water-associated diseases, more than 50% are due to microbial intestinal infections. There are limitations in many of the established methods used in water quality assessments, and new approaches to health-related monitoring are being introduced by WHO that can overcome many of the weaknesses in current methods and provide additional tools for reducing disease risks ( WHO, 2003 ).
2.1.1. The genus Vibrio
Vibrios are small, curved-shaped or rods, facultative anaerobes with a single polar flagellum, belonging to family Vibrionaceae of order Vibrionales that are non–spore-forming and Gram-negative with a size of approximately 1.5–3.0 μm × 0.5 μm. Cells of Vibrio cholerae, Vibrio parahaemolyticus , and Vibrio vulnificus possess pili (fimbriae) structures comprising of protein TcpA. This TcpA formation is co-regulated with cholera toxin expression and is a key determinant of in vivo colonization.
These are primarily aquatic bacteria, common in marine and estuarine environments, living free or on the surfaces and in the intestinal contents of marine animals. Around 12 Vibrio species can infect humans. V. cholerae is, by far, the most important among them. Vibrio fluvialis , Grimontia hollisae (formerly Vibrio hollisae ), and Vibrio mimicus can cause diarrhea or infections of the gastrointestinal tract. Vibrio furnissii has been isolated from a few individuals with diarrhea, but there is no evidence that it can actually cause this pathology.
Cholera is a well-known disease since 19 th century and it is topping the list of microbial waterborne diseases. V. cholerae is a very diverse bacterial species. It has more than 200 serovarieties, characterized by the structure of the lipopolysaccharide (O antigens). Only serovarieties O1 and O139 are involved in true cholera ( Weintraub, 2003 ). Some other serovarieties can cause gastroenteritis. The serovariety O1 is subdivided into classical and El Tor biotypes based on their biochemical properties and phage susceptibilities. Only toxigenic strains have the CTXΦ segment (7–9.7 kb) of the chromosome and this carries at least six genes which have the potential to encode cholera toxin. During chromosome replication, the CTXΦ fragment is able to make an autonomous copy creating an independent plasmid. The plasmid produces virus-like particles, the CTXΦ bacteriophages, which in turn infect nontoxigenic strains ( Cabral, 2010 ). Epidemic and pandemic strains of V. cholerae contain another chromosomal segment designated as Vibrio Pathogenicity Island (VPI). VPI is 39.5 kb in size and contains two ToxR-regulated genes: a regulator of virulence genes (ToxT) and a gene cluster containing colonization factors, including the toxin co-regulated pili. Pathogen can be transmitted by the contaminated water or food via the fecal–oral route. V. cholerae O1 or O139 strains are common in estuaries being isolated from estuarine animals, such as birds, frogs, fishes, and shellfish, and are able to survive and multiply on the surface of phytoplankton and zooplankton cells.
2.1.2. The genus Salmonella
Salmonellae are rod-shaped, motile by peritrichous flagella, belonging to family Enterobacteriaceae of order Enterobacteriales that are non–spore-forming, Gram-negative bacteria with a size of a rod being 0.7–1.5 μm by 2.2–5.0 μm producing colonies approximately 2–4 mm in diameter. Salmonellae have several endotoxins: antigens O, H, and Vi.
Salmonella enterica subsp. enteric serovar Enteritidis is the most frequently isolated serovar from humans all over the world. However, other serovars can be predominant and each outbreak had been associated with a different serotype: Mbandaka, Livingstone, and Typhi Vi+. The major habitat of Salmonella is the intestinal tract of humans and animals and is frequently found in environmental samples because they are excreted by humans as well as animals. Municipal sewage, agricultural waste, and storm water runoff are the main sources of these pathogens in natural waters and they do not multiply much in natural environments but can survive several weeks in water and soil when environmental factors are favorable. Paratyphi or non-Typhi serovars of Salmonellae are more common in the environment. From environmental sources, 73% of the isolates were from tap water in which commonly observed organisms being serovars: Corvallis, Enteritidis, and Anatum ( Aissa et al., 2007 ). A study reported a total of 19 Salmonella serotypes in a comparative study carried out in rivers Aliakmon and Axios, in northern Greece ( Arvanitidou et al., 2005 ).
2.1.3. The genus Shigella
Shigellae are rod-shaped and nonmotile, belonging to family Enterobacteriaceae of order Enterobacteriales that are non–spore-forming, Gram-negative with a size of a cell being 0.4–0.6 μm by 1.0–3.0 μm long. There are four serogroups in Shigella : Shigella dysenteriae (serogroup A) with 1–15 serotypes, Shigella flexneri (serogroup B) serotypes 1–8 with 9 subtypes, Shigella boydii (serogroup C) with serotypes 1–19, and Shigella sonnei (serogroup D) with one serotype. The four serogroups differ in their epidemiology and outbreaks have been attributed to the community water supplies which were not properly chlorinated.
Shigella has a complex antigenic pattern and the serogrouping is based on their somatic O antigens. Shigella emerged from Escherichia coli during evolution. The acquisition and evolution of the pathogenicity island, which encodes all of the genes required for cell invasion and phagolysosomal lysis, permitted a major alteration in pathogenesis. Shigella is the causative agent for the disease shigellosis or bacillary dysentery, naturally spread by fecal-contaminated drinking water or food or by direct contact with an infected person and considered as a disease affecting the under developed displaced people who are lacking the basic hygienic facilities.
2.1.4. The genus Escherichia
Bacteria in genus Escherichia are rod-shaped, non–spore-forming, Gram-negative bacteria belonging to family Enterobacteriaceae of order Enterobacteriales. Commonly found E. coli have a size of 2.0–0.5 μm in diameter. E. coli is a natural and essential part of the bacterial flora in the gut of humans and animals. Most E. coli strains inhabiting colon are nonpathogenic, but certain serotypes have a role in intestinal and extraintestinal diseases, such as urinary tract infections.
There are six different groups of E. coli strains isolated from intestinal diseases based on epidemiological evidence, phenotypic traits, clinical features of the disease, and specific virulence factors. Among them, enterotoxigenic E. coli O148, enterohemorrhagic E. coli O157, and enteroinvasive E. coli O124 serotypes are major disease-causing organisms and can be transmitted through contaminated water.
Enterotoxigenic E. coli (ETEC) serotypes can cause infantile gastroenteritis. Disease is caused due to consumption of ETEC-contaminated food or water and is characterized by profuse watery diarrhea continuing for several days leading to dehydration and malnutrition in young children. ETEC serotype 148 is one of the causative agents of “travelers' diarrhea” that affects individuals who are involved in global traveling.
Shiga toxin-producing E. coli O157:H7 is considered as food and waterborne pathogen that causes diarrhea, hemorrhagic colitis, and hemolytic uremic syndrome (HUS) in humans in both sporadic cases and outbreaks ( Wasey and Salen, 2019 ). The incubation period is 3–4 days, and the symptoms last for 7–10 days. HUS associated with Shiga toxin-producing E . coli O157:H7 cause acute renal failure mostly in children. These bacteria are naturally not a concern in treated drinking water, but outbreaks related to consumption of contaminated water or use of surface water for recreational activities have been documented ( Bruneau et al., 2004 ). Enterohemorrhagic E . coli have also been isolated from ponds, streams, wells, and water troughs, and they can survive for months in manure and water-trough sediments. Personal contacts are an important mode of transmission and disease spread through the oral–fecal route.
Enteroinvasive E. coli act as same as Shigella . They are capable of invading and multiplying in the intestinal epithelial cells of the distal large bowel in humans. The illness is characterized by abdominal cramps, diarrhea, vomiting, fever, chills, a generalized malaise, and the appearance of blood and mucus in the stools of infected individuals. E. coli O124 had been isolated from cases of gastroenteritis, enterocolitis, and dysentery. Food prepared by using water contaminated with human waste could cause the disease in humans.
2.1.5. The genus Burkholderia
Bacteria in the genus Burkholderia are straight or slightly curved, rod-shaped, non–spore-forming and Gram-negative, and motile due to a single or multiple polar flagella except in one species, belonging to family Burkholderiaceae of order Burkholderiales. The genus comprises of 60 species of obligatory aerobes that are ubiquitous in nature and are waterborne pathogens. Clinically relevant species include Burkholderia cepacia complex species, Burkholderia pseudomallei , Burkholderia mallei , Burkholderia gladioli . B . pseudomallei is with a diameter of 0.8 μm, and a length of 1.5 μm can be present in soil or water whether it is surface or ground. Melioidosis is a life-threatening disease caused by B. pseudomallei and is endemic to southeast Asians and to northern Australians and randomly affects people living close to the equator. It is more common during the monsoon season than in dry months and literature confirms that it became more prevalent after 2004 Tsunami ( Currie et al., 2008 ).
Melioidosis has been reported from Thailand and the disease is highly endemic to the northeast. Most infected community is agricultural farmers with repeated environmental exposure ( Limmathurotsakul et al., 2013 ). Water supply–related melioidosis has also been documented and the disease could be acute or chronic. Signs and symptoms may include pain in the chest, bones, or joints; cough; skin infections, lung nodules, and pneumonia, which is a life-threatening infection that is estimated to account for nearly 89,000 deaths per year worldwide ( Wiersinga et al., 2018 ). Melioidosis is an emerging disease in Sri Lanka ( Corea et al., 2012 ).
2.1.6. The genus Campylobacter
The bacteria in genus Campylobacter are Gram-negative, 0.5–8 μm long, and 0.2–0.5 μm wide with characteristically curved, spiral, or S-shaped cells belonging to the family Campylobacteraceae in order Campylobacterales. This genus consists of 29 species and 12 subspecies. The most important Campylobacter species in human gastroenteritis is Campylobacter jejuni followed by Campylobacter coli , Campylobacter lari , and Campylobacter fetus . Campylobacter enteritis was the causative agent for 8.5% of the total burden of diarrheal disease, standing fourth after RV, cryptosporidiosis, and E. coli diarrhea (combined enterotoxigenic and enteropathogenic E. coli infections) ( Murray et al., 2012 ).
Disparities have been observed between developed and developing countries in the epidemiology and demography of Campylobacter infections. In developing countries, symptomatic disease is most commonly seen only during the first 2 years of life, and symptomatic illness in adults is scarce because of the endemic nature. In developed world, the most common symptoms include an acute, self-limiting gastroenteritis, with an incubation period of 2–5 days, whereas in developing nations, watery diarrhea is mostly observed. This is considered also as a zoonotic disease and wide variety of animals, especially poultry, wild birds, cattle, and sheep carry high numbers of C. jejuni and C. coli as commensals in their intestines. Fecal contamination of food, recreational water, and drinking water contributes to human infections and the fecal material of infected persons spread the organisms back to environment through sewage plants and toilets.
2.1.7. The genus Francisella
The genus Francisella is 0.7–1.7 μm in size, nonmotile, Gram-negative, strictly aerobic, and facultative intracellular coccobacilli species. The type species of the genus is the Francisella tularensis , which contains four subspecies, i.e., tularensis , holarctica , mediasiatica , and novicida . F. tularensis is a highly infectious bacterium causing disease in mammals including humans and a potential bioterror weapon ( Colquhoun et al., 2014 ). Tularemia is a zoonotic infection caused by F. tularensis mainly transmitted to humans through arthropod bites, direct contact with infected animals, and inhalation or ingestion of contaminated water. The organism can persist in water or mud at least for 1 year and that indicates the environment may be important reservoirs for this pathogen. Recent and historical outbreaks indicate that environmental exposure to the organism is a significant source of morbidity.
2.1.8. The genus Legionella
Legionellae are rod-shaped, Gram-negative bacteria being the only genus in family Legionellaceae. The genus includes 52 validated species with 71 serotypes out of which 24 Legionella species are described as occasional human pathogens. Legionella pneumophila cause Legionnaires' disease, which is a sever type of pneumonia occurring worldwide. The transmission can occur via inhalation of contaminated aerosols generated by cooling towers, bath tubs, whirlpools, ornamental fountains, and showers. In nature, Legionella live in freshwater and rarely cause illness. Outbreaks of Legionnaires' disease are often associated with man-made water settings, with large or complex water systems. Most outbreaks have been due to L. pneumophila , serogroup 1, and although this may be due to increased virulence, it may simply reflect the greater prevalence of this particular organism ( Yu et al., 2002 ). Biofilms and free-living amebae are considered to serve as main environmental reservoirs for L. pneumophila and represent a potential source of drinking water contamination, resulting in a potential health risk for humans.
2.1.9. Mycobacterium avium complex
The genus Mycobacterium belongs to family Mycobacteriaceae of order Actinomycetales. The Mycobacterium avium complex (MAC) consists of 28 serovars of two distinct species: Mycobacterium avium and Mycobacterium intracellulare. MAC has been recovered from drinking water systems both before and after treatment, hot water heaters, freshwater, brackish, sea water, and wastewater, occasionally being high in numbers, and the infection to humans occurs through the inhalation of aerosolized droplets containing M. avium cells.
The organisms of MAC have the ability to survive and grow under diverse and extreme conditions. Hence mycobacteria are highly resistant to chlorine and other chemical disinfectants as such standard drinking water treatments will not completely eliminate MAC organisms but can minimize the risk. The symptoms encountered with MAC infections result from colonization of either the respiratory or the gastrointestinal tract, with possible dissemination to other locations in the body.
2.2. Waterborne viruses
Diarrheal disease and WBDOs from drinking, recreational, and groundwaters are often caused by waterborne viruses, which tend to be more persistent in the environment than bacteria ( Gibson, 2014 ). WHO has classified adenovirus (AdV), astrovirus (AstV), hepatitis A and E viruses, RV, norovirus, and other caliciviruses and enteroviruses, including coxsackieviruses and polioviruses as water-transmitted viral pathogens having a moderate to high health significance ( WHO, 2011 ). Also, polyomaviruses and cytomegalovirus that are excreted through urine can potentially be spread through water. Influenza and coronaviruses have been proposed as organisms that can be transmitted through drinking water, but evidences are lacking. These viruses are mostly associated with gastroenteritis, which can cause diarrhea as well as other symptoms including abdominal cramping, vomiting, and fever. Some of these same viruses could also cause more severe illnesses including encephalitis, meningitis, myocarditis (enteroviruses), cancer (polyomavirus), and hepatitis (hepatitis A and E viruses) ( WHO, 2011 ).
2.2.1. Adenoviruses
AdVs, belonging to the family Adenoviridae, genus Mastadenovirus , have over 51 serotypes differentiated to six subgroups (A to F), which are the causative agents of many human diseases. They are 80–90 nm in size containing double-stranded linear DNA and a nonenveloped icosahedral shell that has slender projections from each of its 12 vertices. They can infect many organs in the body including the eye, upper respiratory tract, lower respiratory tract, gastrointestinal tract (gastroenteritis and intussusception), urinary bladder, central nervous system, and genitalia. The enteric adenoviruses types 31, 40, 41, and subgenus F are responsible for the majority of adenovirus-mediated cases of gastroenteritis. Pathogenicity of the virus varies with the species and serotype, and organ specificity and disease patterns appear to be serotype-dependent.
Human adenoviruses are transmitted by the fecal–oral route and through inhalation of water droplets. They are listed as pathogens of childhood gastroenteritis as most affected are being children of under 5 years of age. AdV resistance to purification and disinfection processes (i.e., UV inactivation) and the virus's ability to survive in the environment have increased the importance of monitoring AdVs in water ( Jiang, 2006 ). Theses adenoviruses are being documented everywhere in the world, without any seasonal variability and could be found in drinking water if not properly treated, in raw sewage, polluted waters such as rivers and dams, swimming pools, and even in shellfish.
2.2.2. Astroviruses
AstVs are nonenveloped, icosahedral viruses belonging to family Astroviridae . They are 28–41 nm in size, containing positive-sense, single-stranded RNA, genome of approximately 7 kb in size. They have been classified into two genera: Mamastrovirus and Avastrovirus . Genera Mamastrovirus and Avastrovirus cause infection to mammalians and avian, respectively. Three divergent groups of human astroviruses (HAstVs) have been identified and according to research, the classic AstV group contains eight serotypes accounting for less than 10% of all acute nonbacterial gastroenteritis in children worldwide without any geographical boundaries. Children get infected in the first few years of life regardless of their level of hygiene, quality of water, food or sanitation, or type of behavior. Although children are vulnerable, there are reports of disease in normal healthy adults ( Pager and Steele, 2002 ) and also immunocompromised individuals ( González et al., 1998 ). This infection induces mild, watery diarrhea that lasts 2–3 days, associated with vomiting, fever, anorexia, and abdominal pain. In comparison to RV or calicivirus infection, infections due to AstVs have a longer incubation period.
These viruses can be transmitted by the contaminated water via the fecal–oral route, and higher incidence has been recorded in cold months. In temperate regions, most AstV infections are during winter, whereas in tropics, infections occur during rainy months. These viruses are being detected both in surface and groundwaters which are being used as drinking water sources, freshwater, and marine waters as well as in wastewater effluents. Moreover, waterborne transmission of HAstVs has been suggested as a risk of digestive morbidity for the general population ( Gofti-Laroche et al., 2003 ). Chlorine and other disinfectants are effective for the inactivation of these viruses in water.
2.2.3. Caliciviruses
Caliciviruses are nonenveloped, 27–40 nm single-stranded RNA viruses in the family Caliciviridae. They are an important group of human viruses capable of causing gastrointestinal disease in humans that may be found in waters intended by humans for drinking, recreation, and shellfish growing. The International Committee on Taxonomy of Viruses changed the calicivirus nomenclature and classified into four genera: Vesivirus , Lagovirus , Norovirus , and Sapovirus . Sapoviruses (SaVs) and noroviruses (NoVs) are included in the latest US Drinking Water Contaminant Candidate List (CCL) ( Rusinol and Girones, 2017 ). They get spread by the fecal–oral route and are found in contaminated surface and groundwaters. The presence of caliciviruses in groundwaters is an important consideration, as a number of outbreaks have been linked to these drinking water sources (often from shallow wells and springs) ( Schaub and Oshiro, 2000 ).
2.2.4. Noroviruses
Noroviruses (NoVs) (formerly Norwalk virus) were first identified following an outbreak of enteric illness among children and adults in the town of Norwalk, Ohio ( Adler and Zickl, 1969 ). They are a group of nonenveloped, single-stranded RNA viruses with an icosahedral symmetry classified into the genus Norovirus of the family Caliciviridae with a size of 27–32 nm. Most norovirus genomes contain three open reading frames (ORFs). They have rough, nondistinct borders and lack the calyx appearance. Noroviruses are divided into five genogroups (GI to GV), three of which (GI, GII, and GIV) cause human disease. This virus is extremely infectious and humans are the only known reservoir for human norovirus. NoVs cause acute onset of projectile vomiting and diarrhea, sometimes with low-grade fever, headache, and malaise. Symptoms are usually self-limited, lasting for 24–72 h. The incubation period is usually 24–48 h, but onset of symptoms as soon as 10 h after exposure has been reported. Disease outbreaks have been associated with consumption of these viruses in drinking water and also in contaminated shellfish ( Maunula et al., 2005 , Boxman et al., 2006 ).
2.2.5. Sapoviruses
Sapovirus (SaV) is one of the etiological agents of human gastroenteritis, is named after the Japanese city Sapporo, where it was first discovered ( Chiba et al., 1979 ). SaV is an RNA virus with a nonsegmented, positive-sense, single-stranded RNA molecule of approximately 7.3–7.5 kb, belonging to the family Caliciviridae. Genome organization of SaVs differs to NoV and contains only two ORFs instead of three. SaVs have a nonenveloped viral capsid with icosahedral symmetry and display a characteristic surface that has cup-shaped depressions on the surface, formed by the 32 cups or “calices,” which is a typical calicivirus morphology. SaVs show a high level of diversity in their genomes and are currently divided into at least five genetically distinct genogroups. Infections in humans are caused by viruses of genogroup GI, GII, GIV, and GV, and at present human SaV genogroups are classified into 16 genotypes. The disease outbreaks are reported in all age groups including the elderly people ( Lee et al., 2012 ). SaVs are transmitted from person to person via fecal–oral routes and through contaminated foods and water.
2.2.6. Enteroviruses
The waterborne polioviruses, coxsackieviruses, echoviruses, hepatoviruses, and certain unclassified enteroviruses together as a group named as enteroviruses are belonging to the family Picornaviridae. They are small in size, 22–30 nm in diameter, and nonenveloped, and the virions are relatively simple, consisting of a protein capsid surrounding a single-stranded, positive-sense RNA genome and it is assumed that only reservoir for enteroviruses are humans. They are present mainly in sewage contaminated with human feces but can be found in groundwaters, coastal river and marine waters, sewage treatment plants and from solid waste landfills, and insufficiently treated drinking water.
These have been identified as sensitive to formaldehyde, hydroxylamine, UV, ionizing irradiations, and also to ozone but cannot be inactivated with changing pH or with usual chlorination. It is assumed that infections from enteroviruses are associated with poverty and poor hygienic conditions.
2.2.7. Hepatovirus A
Hepatovirus A (HAV) is a nonenveloped virus with an icosahedral capsid of about 27–32 nm, single-stranded having an RNA genome of approximately 7.5 kb and belongs to the family Picornaviridae. HAV is the causative agent of type A viral hepatitis and only one serotype has been reported ( Cristina and Costa-Mattioli, 2007 ). Virus transmission occurs through the fecal–oral route by direct contact with an infected person or exposure to contaminated water or consumption of contaminated food.
The incidence of HAV shows distinct patterns of geographic distribution and being related to standards of hygiene and sanitation, demographic factors and socioeconomic conditions of the population. Most of the infections occur in Africa and Asia, followed by Central and South America, Eastern Europe are considered as areas of intermediate endemicity. Children are more vulnerable to disease in highly endemic areas while adolescents and adults are susceptible to infection in intermediate endemic areas ( WHO, 2012 ).
2.2.8. Hepatovirus E
Hepatovirus E (HEV) is a nonenveloped, positive-sense, single-stranded RNA genome of 7.2 kb in length and belongs to the family Hepeviridae. Family Hepeviridae contains two genera: Orthohepevirus and Piscihepevirus . Four main genotypes of HEV (HEV-1, HEV-2, HEV-3, and HEV-4) belonging to the Orthohepevirus A species are able to infect humans. HEV is primarily transmitted by fecal–oral routes through contaminated foods and water in endemic areas. Also, zoonotic and person-to-person transmission is possible. HEV causes acute hepatitis E in human and an infection is considered to be endemic in many developing countries in Africa and Asia. HEV genotypes 1 and 2 cause epidemic and endemic diseases in developing countries, mainly through contaminated drinking water, while genotypes 3 and 4 cause autochthonous infections mainly in developed countries through a unique zoonotic foodborne transmission ( Khuroo et al., 2016 ).
2.2.9. Rotaviruses
RVs are nonenveloped, double-stranded RNA viruses, belonging to the family Reoviridae. RV is composed of the outer capsid, inner capsid, and core, and genome is composed of 11 segments of double-stranded RNA, which code for six structural and five nonstructural proteins. RV is mainly classified into seven groups (A–G) based on the antigenicity of the inner capsid protein VP6 and genomic characteristics. Among them, rotavirus group A (RVA) strains with distinct G-genotype and P-genotype are the major etiological agents in humans worldwide. Infection with RVA is the most common cause of diarrheal disease among infants and young children and one of the common causes of death in children under 5 years of age ( Walker et al., 2013 ). Virus transmission occurs through the fecal–oral route by direct contact with an infected person and possibly by the respiratory route. RV causes an estimated 2 million hospitalizations and 450,000 deaths among children annually, and the majority of deaths are reported from developing countries in Asia and Africa ( Wang et al., 2014 , Liu et al., 2015 ). The WHO has recommended that the use of RV vaccines in routine immunization programs worldwide to reduce the burden of disease ( WHO, 2009 ).
2.3. Waterborne protozoa
Protozoan parasites were the most frequently identified etiologic agents in WBDOs in 1990s. Further from 1978 through 1991, Giardia lamblia was the most commonly identified pathogen, while in 1992, the numbers of outbreaks reported for giardiasis and cryptosporidiosis were matching. Naegleria fowleri, Acanthamoeba spp., and Entamoeba histolytica are also considered as etiologic agents in WBDOs. Since the potential threat of infection via the waterborne route is being recognized for many of these protozoans, it is crucial that the water industry pays its attention to finding ways to detect these emerging and well-recognized protozoan pathogens in water ( Marshall et al., 1997 ).
2.3.1. The genus Cryptosporidium
Cryptosporidia are zoonotic protozoan parasites with worldwide distribution, consisting of 27 species and more than 60 genotypes. Among Cryptosporidium species identified, Cryptosporidium hominis and Cryptosporidium parvum are the major disease-causing organisms in human. They cause cryptosporidiosis which is a gastrointestinal illness that can last for several days to several weeks. This infection is commonly found in children, immunocompromised individuals, and workers who are frequently exposed to wastewater. The major routes of transmission are not only water and food but also person-to-person contact and respiratory transmission is possible.
2.3.2. The genus Giardia
Giardia are flagellated protozoan parasites belonging to the phylum Metamonada that cause giardiasis, a diarrheal disease in humans and other mammals throughout the world. Since 1920, six Giardia species have been described; Giardia duodenalis (syn. Giardia intestinalis and G . lamblia ) is the major disease-causing organisms in human. Risk from Giardia can be through occupational, accidental, or recreational exposure to surface waters. Brodsky et al. (1974) reported that water contaminated with G. lamblia cysts causes travel-related giardiasis in tourists in certain areas of the world. Giardia species have two major stages in their life cycle, i.e., rapidly multiplying trophozoites and cysts. Cysts are excreted with feces and survive in a variety of environmental conditions. They can be transmitted through contaminated water, food, or direct fecal–oral route.
2.3.3. Entamoeba histolytica
E . histolytica belongs to the family Entamoebidae, an invasive, pathogenic protozoan causing amebiasis while other two species Entamoeba dispar and Entamoeba moshkovskii are nonpathogenic. Life cycle of this E . histolytica includes trophozoite, precyst, cyst, metacyst, and metacystic trophozoite stages. Mature cysts have four nuclei and average 20 μm in diameter, while the motile form trophozoite has a size range of 10–60 μm. The cyst form is the infective form for humans, which can survive in water and food. Infections due to E. histolytica have been recorded globally and it is suggested that from the infected persons around 10% show clinical symptoms. According to literature except for the two parasites, plasmodia and schistosomes, most deaths have been assigned to E. histolytica than any other parasite. In developed countries, risk groups include travelers, immigrants, migrant workers, and immunocompromised individuals. Transmission of this protozoan by water is common in developing countries, where much of the water supply for drinking is untreated and fecally contaminated ( Marshall et al., 1997 ).
2.4. Waterborne helminths
The helminths, generally known as parasitic worms, are invertebrates with elongated, flat, or round bodies which belong to Kingdom Animalia. The major parasitic helminths include in the phylum Nematoda (roundworms) and the phylum Platyhelminthes (trematodes). Helminth parasites infect a large number of people and animals worldwide, mainly in developing countries due to lack of water, sanitation, and hygiene facilities. Dracunculus medinensis (Guinea worm) and Fasciola spp. ( Fasciola hepatica and Fasciola gigantica ) (liver flukes) are the major helminths which can be transmitted through drinking water.
2.4.1. The genus Dracunculus
The genus Dracunculus belongs to the phylum Nematoda and family Dracunculidae, which is parasite of mammals and reptiles. There are 14 valid species in this genus but D. medinensis has been well-studied because of human infections. Dracunculiasis or Guinea-worm disease (GWD) is an avoidable waterborne disease caused by the parasite D. medinensis which affect the populations in rural parts of South Asia and Africa. Reported cases worldwide annually have declined from an estimated 3.5 million cases in 1986 to only 28 cases in 2018 ( WHO, 2019 ). GWD is now restricted to some communities in remote parts of Africa. Humans get exposed to the disease through consumption of drinking water containing Cyclops spp. carrying infectious D. medinensis larvae. After ingestion, larvae are released, penetrate the intestinal and peritoneal walls, and inhabit the subcutaneous tissues.
2.4.2. The genus Fasciola
The genus Fasciola belongs to the phylum Platyhelminthes and family Fasciolidae, which causes fasciolosis in human and ruminants. The main pathogenic species are F . hepatica (temperate fluke) and F . gigantica (tropical fluke). It is estimated that more than 17 million people are infected worldwide and about 180 million people living in endemic areas are at risk to infection ( Cwiklinski et al., 2016 ). Human infection generally occurs through consumption of aquatic vegetables such as watercress, drinking water contaminated with encysted cercariae, or washing utensils with contaminated water.
The above-discussed and the most important waterborne pathogens belonging to the four categories bacteria, viruses, protozoa, and helminths, their diseases, and mode of transmission are summarized in Table 2.1 .
Table 2.1
The waterborne pathogens.
Pathogen | Disease | Mode of transmission |
---|---|---|
Bacteria | ||
serovarieties O1 and O139 | Cholera, gastroenteritis | Fecal–oral route |
sp. | Salmonellosis, gastroenteritis | Fecal–oral route |
sp. | Shigellosis | Fecal–oral route |
, serotype O157:H7 | Diarrhea, hemorrhagic colitis, hemolytic uremic syndrome | Fecal–oral route |
Melioidosis | Direct contact with contaminated soil and surface waters | |
sp. | Diarrhea, gastroenteritis | Consumption of contaminated food |
Tularemia | Arthropod bites, direct contact with infected animals, and inhalation or ingestion of contaminated water | |
Acute respiratory illness, pneumonia (legionellosis) | Inhalation of contaminated aerosols | |
complex (MAC) | Pulmonary disease, skin infection | Inhalation of contaminated aerosols |
Viruses | ||
Adenovirus | Gastroenteritis, respiratory, ocular, and urinary tract infections | Inhalation of contaminated aerosols, fecal–oral route |
Astrovirus | Gastroenteritis, respiratory infections | Fecal–oral route |
Norovirus | Gastroenteritis, diarrhea | Fecal–oral route |
Sapoviruses | Acute viral gastroenteritis | Fecal–oral route |
Hepatitis A and E viruses | Hepatitis | Fecal–oral route |
Rotavirus | Diarrhea, gastroenteritis | Fecal–oral route |
Protozoa | ||
sp. | Cryptosporidiosis | Fecal–oral route |
Giardiasis | Fecal–oral route | |
Amebiasis | Fecal–oral transmission | |
Toxoplasmosis | Ingestion of water contaminated with oocysts | |
Helminths | ||
Dracunculiasis | Consumption of contaminated water | |
Fascioliasis | Consumption of aquatic plants with metacercariae |
3. Potential waterborne pathogens
In 1997 , WHO defined emerging pathogens as those that have appeared in a human population for the first time or have occurred previously but are increasing in incidence or expanding into geographical areas where they have not previously been reported. Reemerging pathogens are those whose occurrence is increasing as a result of long-term changes in their underlying epidemiology ( WHO, 2003 ). By these criteria, 175 species of infectious agent from 96 different genera were classified as emerging pathogens in 1970s and from this group, 75% were zoonotic species. However, currently several of this microorganism from environmental sources, including water, have been confirmed as pathogens, including Cryptosporidium , Legionella , E. coli O157, RV, hepatitis E virus, and norovirus. H . pylori is an example of a recently emerged pathogen that may be transmitted through water ( WHO, 2003 ).
3.1. Potential waterborne bacteria
3.1.1. helicobacter pylori.
Bacteria of genus Helicobacter are Gram-negative, curved, or spiral-shaped belonging to the family Helicobacteraceae and class Epsilonproteobacteria . H . pylori is a helix-shaped bacterium, 3 μm long with a diameter about 0.5 μm. Genus Helicobacter contains more than 40 described species and 4 Candidatus species, a designation of provisional status by International Committee on Systematic Bacteriology for incompletely described prokaryotes, and is divided according to their major colonization sites as gastric or lower intestinal tract–associated bacterial species. It is a genetically diverse gastric pathogen, carrying a range of antibiotic resistance patterns, and varies in geographic occurrence.
These are considered as major etiologic agent for gastritis and are also connected to pathogenesis of peptic and duodenal ulcer disease and gastric carcinoma. But most individuals remain asymptomatic. Approximately 70%–90% of persons in developing countries and 25%–50% of those in developed countries are colonized by H. pylori and it is transmitted mainly by fecal–oral or oral–oral routes, with water and food as the sources ( Doyle, 2012 ). Epidemiological studies have associated the H. pylori infection with lack of access to potable drinking water and proper sanitation H. pylori in drinking water biofilms, change their morphology, and persist for more than 1 month, with densities exceeding 10 6 cells/cm 2 ( Giao et al., 2008 ).
3.1.2. Aeromonas hydrophila
Aeromonas are straight, coccobacillary to bacillary, belonging to family Aeromonadaceae of order Aeromonadales who are non–spore-forming, facultative anaerobic, Gram-negative bacteria with cells having a size of 0.3–1.0 × 1.0–3.5 μm. Although Aeromonas hydrophila is usually the dominant species, other aeromonads, such as Aeromonas caviae and Aeromonas sobria , have also been isolated from human feces and water sources.
A. hydrophila has been recognized as an opportunistic pathogen being identified as a potential agent of gastroenteritis, septicemia, meningitis, and in wound infections. It plays a significant role in intestinal disorders in children under 5 years old, the elderly, and immunosuppressed people. Ubiquitous in nature, it is frequently isolated from food, drinking water, and aquatic environments. In surface waters, mainly rivers and lakes, concentrations of Aeromonas spp. are high but groundwaters generally contain lesser numbers. Drinking water immediately leaving the treatment plant could contain between 0 and 10 2 CFU/mL and these waters can display higher Aeromonas concentrations, due to the growth in biofilms ( Chauret et al., 2001 ). A. hydrophila is resistant to standard chlorine treatments and it is assumed that they survive by being within the biofilms. The common routes of infection are the ingestion of contaminated water or food or through skin. No person-to-person transmission has been reported.
3.1.3. The genus Leptospira
The genus Leptospira belongs to family Leptospiraceae of the phylum Spirochaete and currently contains 20 species including 9 pathogenic, 6 saprophytic, and 5 being intermediate. They are thin, tightly coiled, motile spirochetes, generally 6–20 mm in length, but during culturing they may produce much longer cells. The surface structure of the Leptospira shows both Gram-negative and Gram-positive characteristics. The disease leptospirosis is one of the most widespread zoonotic diseases, infecting both human and animals caused by the Leptospira . The major route of exposure to the pathogen is indirect contact with contaminated water or moist soil. In developing countries from tropics, leptospirosis is an occupational infection, most affected being the people who are engaged in farming, sharecropping, and in animal husbandry ( Levett, 2001 ). Furthermore, there is a significant risk of exposure during recreational activities. Leptospirosis is essentially waterborne infection, as several outbreaks of disease have been recorded during rainy season. Both pathogenic and saprophytic strains of leptospirosis have been isolated from water sources including rivers and lakes as they are able to survive in moist soil and freshwater for long periods of time ( Pal and Hadush, 2017 ).
3.1.4. The genus Tsukamurella
Bacteria belonging to genus Tsukamurella of order Actinomycetales are Gram-positive, nonmotile, obligate aerobic, irregular, and rod-shaped. The genus includes 11 species and out of which 9 Tsukamurella species have been isolated from human infections. Most of Tsukamurella species exist as environmental saprophytes present in soil, arthropods, water, sludge foam, and sponges. Some species have been detected in drinking water supplies, but there is no evidence of correlation between the presence of organisms and the disease. They are opportunistic pathogens and can transmit through clinical instruments such as catheters or lesions. Tsukamurella cause various infections in humans, including pulmonary and cutaneous infections and meningitis and most vulnerable are immunocompromised individuals.
3.1.5. The genus Bacillus
Bacteria belonging to genus Bacillus of phylum Firmicutes are rod-shaped, Gram-positive, strictly aerobic, or facultatively anaerobic and are capable of endospore formation. Bacillus species are commonly found in soil and water. They have been detected in drinking water supplies even after disinfection processes, but waterborne transmission is not yet confirmed. Only few Bacillus species are pathogenic to human. With Bacillus cereus causing bacteremia in immunocompromised patients, B. anthracis causes anthrax in humans and animals. In a study conducted by Taylor et al. (2005) , strains of Bacillus megaterium, Bacillus firmus , Bacillus simplex , and B. cereus were found to produce heat-stable toxins, with varying levels of toxicity.
3.1.6. Cyanobacteria and cyanotoxins
Cyanobacteria are a phylum with an estimated 150 genera of cyanobacteria containing approximately 2000 species, of which around 46 have been reported as being toxigenic. They are oxygenic, photosynthetic, Gram-negative bacteria that inhabit a large variety of terrestrial and aquatic habitats, showing a wide diversity in morphology and their average cell size ranges from 0.5 to 60 μm. In 1998, cyanobacteria were included as a microbial contaminant to CCL because of their potential for transmission through drinking water. Furthermore, microcystin-LR, cylindrospermopsin, and anatoxin-a produced by several species of cyanobacteria are also included in the CCL. These cyanotoxins have been reported from water reservoirs around the world which had caused acute and chronic illnesses in animals and humans ( Liyanage et al., 2016 ). Exposure to cyanotoxins can be through contaminated drinking water, ingestion and dermal skin contact during recreational activities ( Fig. 2.2 ), inhalation of aerosols, medical treatments (dialysis), or through algal food supplements.

A lake in a developing country where people are engaged in recreational activities. (A) Lake Gregory, Sri Lanka; (B) recreational activity area; (C) people engaged in boat riding; potential toxic cyanobacteria; (D) Anabaena sp.; (E) Oscillatoria sp.; (F) Microcystis sp. ( Magana-Arachchi et al., 2011 ).
3.2. Potential waterborne viruses
In 2017, GWPP reported 10 emerging viruses with potential for waterborne transmission including genera Alphatorquevirus , Cyclovirus , Erythroparvovirus , Bocaparvovirus , Protoparvovirus , Alphapapillomavirus , Betapapillomavirus , Picobirnavirus , Betapolyomavirus , and Alphapolyomavirus ( Rusinol and Girones, 2017 ) ( Table 2.2 ).
Table 2.2
The potential waterborne pathogens.
Pathogen | Disease | Mode of transmission |
---|---|---|
Bacteria | ||
Gastritis, peptic and duodenal ulcer disease, and gastric carcinoma | Oral–oral or fecal–oral transmission | |
Gastroenteritis, septicemia, meningitis, and wound infections, intestinal disorders in children | Ingestion of contaminated water or food, through skin | |
sp. | Leptospirosis | Through water contaminated by urine from infected animals |
sp. | Pulmonary and cutaneous infections, meningitis | Through clinical instruments such as catheters or lesions |
sp. | Diarrhea | Through drinking water |
Cyanobacteria and cyanotoxins | Gastrointestinal symptoms, skin rashes, kidney disease | Through drinking water, bathing in contaminated water |
Viruses | ||
Asymptomatic. May be associated with hepatitis, pulmonary diseases, hematologic disorders, myopathy, and lupus | Fecal–oral route | |
Systemic infections may play a role in development of paraplegia | Fecal–oral and foodborne transmission | |
Fifth disease in children, arthropathy, hepatitis | Respiratory route | |
Gastroenteritis, related to respiratory infections | Respiratory and fecal–oral routes | |
Gastroenteritis | Respiratory and fecal–oral routes | |
Cervix, penis, anus, and vulva cancers | Direct skin-to-skin or skin-to-mucosa contact | |
Related to genital warts | Direct skin-to-skin or skin-to-mucosa contact | |
May be implicated in gastroenteritis | Fecal–oral route | |
Progressive multifocal encephalopathy | Fecal–oral route | |
Associated to Merkel cell carcinoma | Fecal–oral route | |
Protozoa | ||
Microsporidiosis | Fecal–oral transmission | |
Diarrheal illness, gastroenteritis | Fecal–oral transmission | |
Cystoisosporiasis | Fecal–oral transmission | |
Helminths | ||
sp. | Schistosomiasis, liver and kidney damage | Penetrate the skin during contact with infested water |
3.3. Potential waterborne protozoa
3.3.1. microsporidia.
Microsporidia belonging to the phylum Microspora include over 140 genera and 1200 species that are parasitic in all major animal groups. They are obligate intracellular, spore-forming protists. The spore is the only stage that can survive outside the host cell in their life cycle and it contains a characteristic coiled polar filament for injecting the sporoplasm into a host cell to initiate infection. After infection, a complex process of multiplication takes place within an infected cell and new spores are produced and released to feces, urine, respiratory secretions, or other body fluids, depending on the type of species and the site of infection. Among 14 human pathogenic Microsporidia species, two species, Enterocytozoon bieneusi and Encephalitozoon intestinalis , are the most prevalent species which associated with gastrointestinal disease in humans. Person-to-person contact and ingestion of spores in water and food contaminated with human feces or urine are considered as important routes of exposure. A study by Dowd et al. (1998) showed that 7 out of 14 water concentrates tested were contaminated with E. intestinalis , E. bieneusi , and Vittaforma corneae which represent human pathogenic microsporidia species. Their study is the first species level confirmation of human pathogenic microsporidia in water, indicating that these human pathogenic microsporidia possibly be waterborne pathogens. Microsporidiosis is an emerging disease in immunosuppressed persons with AIDS, but microsporidia have the ability to cause disease even in immunologically normal hosts.
3.3.2. Cystoisospora belli
Cystoisospora (formerly Isospora ) are coccidian parasites, belonging to the phylum Apicomplexa, found mainly in tropical and subtropical areas. Many Cystoisospora species can infect animals, but human is the only known host for C . belli ( Lindsay et al., 1997 ) . C. belli infects the epithelial cells of the small intestine of human, and immunocompromised individuals are more susceptible to the infection. The immature form of the parasite is known as oocytes; they are passed out with feces and then mature outside the body in 2–3 days, depending on environmental conditions. It can be transmitted through contaminated water and food. However, direct person-to-person transmission is unlikely. The improved practice of personal hygiene and sanitation may help in preventing transmission of disease.
3.3.3. Cyclospora cayetanensis
Cyclospora cayetanensis belongs to the family Eimeriidae, 7.5–10 μm in diameter, cyst-forming, and unsporulated when passed in feces. It is an apicomplexa coccidia closely related to Eimeria species, recognized as an emerging protist that causes diarrheal illness and significantly contributes to the burden of gastroenteritis worldwide.
3.4. Potential waterborne helminths
3.4.1. the genus schistosoma.
Schistosomes are trematode parasites which cause schistosomiasis (or bilharzia) in human. The main human pathogenic species are Schistosoma mansoni , Schistosoma japonicum , and Schistosoma haematobium . Schistosomiasis is a waterborne disease mostly seen in the tropics and subtropics. The humans get exposed when their skin comes into contact with infested freshwater, into which the cercariae of the parasite are released by freshwater snails. It is understood that for each of the human schistosomes, the presence of a specific genus of snail is necessary for transmission to occur. This disease has been considered as a disease due to poverty, and controlling of this disease has been a problem because of the lack of clean water available to people living in the developing countries.
The most important potential waterborne pathogens as described by WHO belonging to the four categories bacteria, viruses, protozoa, and helminths, their diseases, and mode of transmission are summarized in Table 2.2 .
This chapter provides a general description on current waterborne pathogens as well as emerging and potential pathogens which could be categorized into bacteria, viruses, protozoans, and helminths. Most of these microorganisms are ubiquitous in waters regardless of ground, surface, fresh, or marine. People utilize these waters for drinking, cooking, and other domestic actions, bathing, medically, and also for recreations. The water sources become polluted due to the mixing of fecal matter from human and animal and also waste generated by other direct and indirect anthropogenic activities. As a result, waters become reservoirs for the pathogens making it unsafe for human consumption causing many waterborne diseases. In addition, with the increase in global population, changes in climatic patterns, and the presence of antibiotic resistant bacteria in waste waters, it is predicted that there will be a rise in waterborne diseases especially diarrhea and cholera. Therefore, still the global populations infants, young, or old are at a risk from waterborne diseases and outbreaks whether the countries are developed or developing or in tropics or temperate in geographical distribution. Hence to minimize the adverse effects from these waterborne pathogens and to improve the water quality, regular monitoring of water sources is essential with advanced but cost-effective detection techniques, precise disinfectant procedures with proper management.
Acknowledgment
We are expressing our sincere gratitude to Ms. Chanusha Weralupitiya for technical support in preparation of Fig. 2.1 .
- Adler L., Zickl R. Winter vomiting disease. J. Infect. Dis. 1969; 119 (6):668–673. [ PubMed ] [ Google Scholar ]
- Aissa R., Al-Gallas N., Troudi H., Belhadj N., Belhadj A. Trends in Salmonella enterica serotypes isolated from human, food, animal, and environment in Tunisia, 1994–2004. J. Infect. 2007; 55 (4):324–339. [ PubMed ] [ Google Scholar ]
- Arvanitidou M., Kanellou K., Vagiona D. Diversity of Salmonella spp. and fungi in northern Greek rivers and their correlation to fecal pollution indicators. Environ. Res. 2005; 99 (2):278–284. [ PubMed ] [ Google Scholar ]
- Bivins A., Sumner T., Kumpel E., Howard G., Cumming O., Ross I., Nelson K., Brown J. Estimating infection risks and the global burden of diarrheal disease attributable to intermittent water supply using QMRA. Environ. Sci. Technol. 2017; 51 (13):7542–7551. [ PubMed ] [ Google Scholar ]
- Boxman L., Tilburg J., TeLoeke A., Vennema H., Jonker K., De Boer E., Koopmans M. Detection of noroviruses in shellfish in The Netherlands. Int. J. Food Microbiol. 2006; 108 (3):391–396. [ PubMed ] [ Google Scholar ]
- Bridle H. Overview of waterborne pathogens. In: Bridle H., editor. Waterborne Pathogens: Detection Methods and Applications. Academic Press; 2013. [ Google Scholar ]
- Brodsky E., Spencer C., Schultz G. Giardiasis in American travelers to the Soviet Union. J. Infect. Dis. 1974; 130 (3):319–323. [ PubMed ] [ Google Scholar ]
- Bruneau A., Rodrigue H., Ismael J., Dion R., Allard R. Outbreak of E. coli O157:H7 associated with bathing at a public beach in the Montreal-Centre region. Can. Commun. Dis. Rep. 2004; 30 (15):133–136. [ PubMed ] [ Google Scholar ]
- Cabral J. Water microbiology. Bacterial pathogens and water. Int. J. Environ. Res. Public Health. 2010; 7 (10):3657–3703. [ PMC free article ] [ PubMed ] [ Google Scholar ]
- Chauret C., Volk C., Creason R., Jarosh J., Robinson J., Warnes C. Detection of Aeromonas hydrophila in a drinking-water distribution system: a field and pilot study. Can. J. Microbiol. 2001; 47 (8):782–786. [ PubMed ] [ Google Scholar ]
- Chiba S., Sakuma Y., Kogasaka R., Akihara M., Horino K., Nakao T., Fukui S. An outbreak of gastroenteritis associated with calicivirus in an infant home. J. Med. Virol. 1979; 4 (4):249–254. [ PubMed ] [ Google Scholar ]
- Colquhoun J., Larsson P., Duodu S., Forsman M. The family Francisellaceae . In: Rosenberg E., DeLong E.F., Lory S., Stackebrandt E., Thompson F., editors. The Prokaryotes. Springer; Berlin, Heidelberg: 2014. [ Google Scholar ]
- Corea E., Thevanesam V., Perera S., Jayasinghe I., Ekanayake A., Masakorala J., Inglis T. Melioidosis in Sri Lanka: an emerging infection. Sri Lankan J. Infect. Dis. 2012; 1 (2):2–8. [ Google Scholar ]
- Cristina J., Costa-Mattioli M. Genetic variability and molecular evolution of hepatitis A virus. Virus Res. 2007; 127 (2):151–157. [ PubMed ] [ Google Scholar ]
- Currie B., Dance D., Cheng A. The global distribution of Burkholderia pseudomallei and melioidosis: an update. Trans. R. Soc. Trop. Med. Hyg. 2008; 102 (S1):S1–S4. [ PubMed ] [ Google Scholar ]
- Cwiklinski K., O'neill S.M., Donnelly S., Dalton J.P. A prospective view of animal and human fasciolosis. Parasite Immunol. 2016; 38 (9):558–568. [ PMC free article ] [ PubMed ] [ Google Scholar ]
- Dowd E., Gerba P., Pepper L. Confirmation of the human-pathogenic microsporidia Enterocytozoon bieneusi, Encephalitozoon intestinalis , and Vittaforma corneae in water. Appl. Environ. Microbiol. 1998; 64 (9):3332–3335. [ PMC free article ] [ PubMed ] [ Google Scholar ]
- Doyle E. University of Wisconsin; Madison: 2012. Helicobacter spp.- Food- or Waterborne Pathogens? FRI Food Safety Review. [ Google Scholar ]
- Giao M., Azevedo N., Wilks S., Vieira M., Keevil C. Persistence of Helicobacter pylori in heterotrophic drinking-water biofilms. Appl. Environ. Microbiol. 2008; 74 (19):5898–5904. [ PMC free article ] [ PubMed ] [ Google Scholar ]
- Gibson K.E. Viral pathogens in water: occurrence, public health impact, and available control strategies. Curr. Opin. Virol. 2014; 4 :50–57. [ PMC free article ] [ PubMed ] [ Google Scholar ]
- Gofti-Laroche L., Gratacap-Cavallier B., Demanse D., Genoulaz O., Seigneurin J., Zmirou D. Are waterborne astrovirus implicated in acute digestive morbidity (E.MI.R.A. study)? J. Clin. Virol. 2003; 27 (1):74–82. [ PubMed ] [ Google Scholar ]
- González G., Pujol H., Liprandi F., Deibis L., Ludert E. Prevalence of enteric viruses in human immunodeficiency virus seropositive patients in Venezuela. J. Med. Virol. 1998; 55 (4):288–292. [ PubMed ] [ Google Scholar ]
- Jiang C. Human adenoviruses in water: occurrence and health implications: a critical review. Environ. Sci. Technol. 2006; 40 (23):7132–7140. [ PubMed ] [ Google Scholar ]
- Jin D., Kong X., Cui B., Jin S., Xie Y., Wang X., Deng Y. Bacterial communities and potential waterborne pathogens within the typical urban surface waters. Sci. Rep. 2018; 8 (1):13368. [ PMC free article ] [ PubMed ] [ Google Scholar ]
- Khuroo M., Khuroo M., Khuroo N. Transmission of hepatitis E virus in developing countries. Viruses. 2016; 8 (9):253. [ PMC free article ] [ PubMed ] [ Google Scholar ]
- Lee E., Cebelinski A., Fuller C., Keene E., Smith K., Vinjé J., Besser M. Sapovirus outbreaks in long-term care facilities, Oregon and Minnesota, USA, 2002-2009. Emerg. Infect. Dis. 2012; 18 (5):873–876. [ PMC free article ] [ PubMed ] [ Google Scholar ]
- Levett N. Leptospirosis. Clin. Microbiol. Rev. 2001; 14 (2):296–326. [ PMC free article ] [ PubMed ] [ Google Scholar ]
- Limmathurotsakul D., Kanoksil M., Wuthiekanun V., Kitphati R., Day P., Peacock J. Activities of daily living associated with acquisition of melioidosis in northeast Thailand: a matched case-control study. PLoS Neglected Trop. Dis. 2013; 7 (2):2072. [ PMC free article ] [ PubMed ] [ Google Scholar ]
- Lindsay S., Dubey P., Blagburn L. Biology of Isospora spp. from humans, nonhuman primates, and domestic animals. Clin. Microbiol. Rev. 1997; 10 (1):19–34. [ PMC free article ] [ PubMed ] [ Google Scholar ]
- Liu L., Oza S., Hogan D., Perin J., Rudan I., Lawn J.E., Cousens S., Mathers C., Black R.E. Global, regional, and national causes of child mortality in 2000-13, with projections to inform post-2015 priorities: an updated systematic analysis. Lancet. 2015; 385 (9966):430–440. [ PubMed ] [ Google Scholar ]
- Liyanage H.M., Magana Arachchi D.N., Abeysekara T., Guneratne L. Toxicology of freshwater cyanobacteria. J. Environ. Sci. Health Part C. 2016; 34 (3):137–168. [ PubMed ] [ Google Scholar ]
- Magana-Arachchi D.N., Wanigatunge R.P., Liyanage H.M. Molecular characterization of cyanobacterial diversity in Lake Gregory, Sri Lanka. Chin. J. Oceanol. Limnol. 2011; 29 (4):898–904. [ Google Scholar ]
- Marshall M.M., Naumovitz D., Ortega Y., Sterling C.R. Waterborne protozoan pathogens. Clin. Microbiol. Rev. 1997; 10 (1):67–85. [ PMC free article ] [ PubMed ] [ Google Scholar ]
- Maunula L., Miettinen T., Von Bonsdorff H. Norovirus outbreaks from drinking water. Emerg. Infect. Dis. 2005; 11 (11):1716–1721. [ PMC free article ] [ PubMed ] [ Google Scholar ]
- Murray J., Vos T., Lozano R., Naghavi M., Flaxman D., Michaud C., Ezzati M., Shibuya K., Salomon A., Abdalla S., Aboyans V. Disability-adjusted life years (DALYs) for 291 diseases and injuries in 21 regions, 1990–2010: a systematic analysis for the global burden of disease study 2010. Lancet. 2012; 380 (9859):2197–2223. [ PubMed ] [ Google Scholar ]
- Pager T., Steele D. Astrovirus-associated diarrhea in South African adults. Clin. Infect. Dis. 2002; 35 (11):1452–1453. [ PubMed ] [ Google Scholar ]
- Pal M., Hadush A. Leptospirosis: an infectious emerging waterborne zoonosis of global significance. Air Water Borne Dis. 2017; 6 :1–4. [ Google Scholar ]
- Patel C., Shanker R., Gupta V., Upadhyay R. Q-PCR based culture-independent enumeration and detection of Enterobacter: an emerging environmental human pathogen in riverine systems and potable water. Front. Microbiol. 2016; 7 :172. [ PMC free article ] [ PubMed ] [ Google Scholar ]
- Rusinol M., Girones R. Summary of excreted and waterborne viruses. In: Rose J.B., Jiménez-Cisneros B., editors. Global Water Pathogen Project (GWPP) Michigan State University; E. Lansing, MI: 2017. UNESCO. [ Google Scholar ]
- Schaub S., Oshiro R. Public health concerns about Caliciviruses as waterborne contaminants. J. Infect. Dis. 2000; 181 (s2):S374–S380. [ PubMed ] [ Google Scholar ]
- Taylor J.M., Sutherland A.D., Aidoo K.E., Logan N.A. Heat-stable toxin production by strains of Bacillus cereus, Bacillus firmus, Bacillus megaterium, Bacillus simplex and Bacillus licheniformis . FEMS Microbiol. Lett. 2005; 242 (2):313–317. [ PubMed ] [ Google Scholar ]
- United Nations General Assembly (UNGA) 2010. The Human Right to Water and Sanitation: Resolution/Adopted by the General Assembly. [ Google Scholar ]
- UNSD . United Nations General Assembly Resolution (UNSD); 2015. Transforming Our World: The 2030 Agenda for Sustainable Development. A/RES/70/1. [ Google Scholar ]
- Walker F., Rudan I., Liu L., Nair H., Theodoratou E., Bhutta A., O'Brien L., Campbell H., Black E. Global burden of childhood pneumonia and diarrhoea. Lancet. 2013; 381 (9875):1405–1416. [ PMC free article ] [ PubMed ] [ Google Scholar ]
- Wang H., Liddell A., Coates M., Mooney D., Levitz E., Schumacher E., Murray C.J. Global, regional, and national levels of neonatal, infant, and under-5 mortality during 1990–2013: a systematic analysis for the global burden of disease study 2013. Lancet (Lond Engl) 2014; 384 (9947):957–979. [ PMC free article ] [ PubMed ] [ Google Scholar ]
- Wasey A., Salen P. StatPearls [Internet] StatPearls Publishing; Treasure Island, FL: 2019. Escherichia coli ( E. coli 0157 H7) https://www.ncbi.nlm.nih.gov/books/NBK507845/ (Updated 2019 Feb 3) Available from: [ PubMed ] [ Google Scholar ]
- Weintraub A. Immunology of bacterial polysaccharide antigens. Carbohydr. Res. 2003; 338 (23):2539–2547. [ PubMed ] [ Google Scholar ]
- WHO, UNICEF . World Health Organization (WHO) and the United Nations Children’s Fund (UNICEF); Geneva: 2017. Progress on Drinking Water, Sanitation and Hygiene: Update and Sustainable Development Goal Baselines. License: CC BY-NC-SA 3.0 IGO. [ Google Scholar ]
- WHO, UNICEF, World Bank . third ed. 2009. State of the World's Vaccines and Immunization. Geneva. [ Google Scholar ]
- Wiersinga J., Virk S., Torres G., Currie J., Peacock J., Dance A., Limmathurotsakul D. Melioidosis. Nat. Rev. Dis. Prim. 2018; 4 :17107. [ PMC free article ] [ PubMed ] [ Google Scholar ]
- World Health Organization . 1997. Division of Emerging and Communicable Diseases Surveillance and Control Annual Report- 1996. [ Google Scholar ]
- World Health Organization . 2003. Emerging Issues in Water and Infectious Disease. [ Google Scholar ]
- World Health Organization . fourth ed. WHO Press; Geneva, Switzerland: 2011. Guidelines for Drinking-Water Quality. [ Google Scholar ]
- World Health Organization WHO position paper on hepatitis A vaccines-June 2012. Wkly. Epidemiol. Rec. 2012; 87 (28–29):261–276. [ Google Scholar ]
- World Health Organization . 2019. Dracunculiasis (Guinea-Worm Disease) Fact Sheet. [ Google Scholar ]
- Yu L., Plouffe F., Pastoris C., Stout E., Schousboe M., Widmer A., Summersgill J., File T., Heath M., Paterson L., Chereshsky A. Distribution of Legionella species and serogroups isolated by culture in patients with sporadic community-acquired legionellosis: an international collaborative survey. J. Infect. Dis. 2002; 186 (1):127–128. [ PubMed ] [ Google Scholar ]

IMAGES
VIDEO
COMMENTS
The. most common waterborne diseases in Nigeria. include Cholera, Dracunculiasis, Hepatitis, and. Typhoid [ 27]. Cases of water borne diseases. linked to contaminations of drinking water with ...
Several research reports, government and non-government resources demonstrate this quietly. ... For this paper, only waterborne hepatitis viruses A and E will be discussed. In populations with unsafe water and inadequate sanitation, viral hepatitis A and E are food and waterborne diseases that can cause acute epidemics. ... N-hexane and water ...
1. Introduction. Waterborne disease is a global burden which is estimated to cause more than 2.2 million deaths pear year and higher cases of illness every day, including diarrhea, gastrointestinal diseases and systematic illnesses [1,2].About 1.4 millions of these deaths are children [].It is suggested that waterborne diseases have an economic cost associated of 1 billion dollars annually ...
Purpose: Climate change threatens progress achieved in global reductions of infectious disease rates over recent decades. This review summarizes literature on potential impacts of climate change on waterborne diseases, organized around a framework of questions that can be addressed depending on available data. Recent findings: A growing body of ...
1. Introduction. Water-borne diseases (WBDs) are infectious diseases, such as cholera, shigella, typhoid, hepatitis A and E, and poliomyelitis, that are transmitted to humans through contaminated water [].These infections are caused by a number of bacterial, viral, and parasitic organisms where there is inadequate sanitation, hygiene, and safe water for drinking, cooking and cleaning [].
Climate-sensitive waterborne diseases have a distinct seasonal pattern, whereby warm summer months are associated with an increased incidence of bacterial diarrheal infections — specifically ...
Department of Geography, Egerton University, Nakuru, Kenya. Abstract. Waterborne diseases remain a major global public health issue and a great. environmental concern and the outbreak is common in ...
The paper examines the global trends and main health impacts of these events based on databases and case studies, identifies gaps in the Sustainable Development Goals (SDGs) indicator framework for monitoring health impacts of disasters and suggests recommendations to address these gaps. ... Water-borne diseases: gastrointestinal (GI) disease ...
Author Summary Water is essential for maintaining life on Earth but can also serve as a media for many pathogenic organisms, causing a high disease burden globally. However, how the global distribution of water-associated infectious pathogens/diseases looks like and how such distribution is related to possible social and environmental factors remain largely unknown. In this study, we compiled ...
Table 1 Water-borne diseases cases reported during 2014-2019 in India [32, 33] ... The business of sanitation provides a lot of prospects for the corporate sector to conduct research on waterborne diseases, their management, and methods of reducing their occurrence with innovative products and services. ... The paper does not contain any ...
Climate change has significantly influenced the spread of waterborne diseases (WBDs), which affect environmental quality and human life. The impact of climate change is greatest in developing countries, especially in the Association of Southeast Asian Nations (ASEAN) countries. Vibrio cholerae, a waterborne pathogen, is most susceptible to and most prevalent during severe climatic changes. The ...
Waterborne diseases continue to take a heavy toll on the global community, with developing nations, and particularly young children carrying most of the burden of morbidity and mortality. Starting with the historical context, this article explores some of the reasons why this burden continues today, despite our advances in public health over the past century or so. While molecular biology has ...
The unavailability of pipe-borne water and the dependence of rural dwellers on surface waters which are often contaminated with faecal materials are the major causes of the rising prevalence of waterborne diseases. Despite numerous efforts by government at various levels and other agencies interested in water and its safety, waterborne diseases are still a major public health and environmental ...
The concept of water-borne diseases is used and defined differently in the theories and practices of different actors involved in the field. The US Centers for Disease Control and Prevention (CDCs, see chapter "Centers for Disease Control and Prevention (CDCs)") refer to water-borne disease as an "illness that occurs after consumption or use of water intended for drinking (potable), or ...
Contributors to this chapter discuss a broad range of responses to the threat of waterborne disease, including drinking water disinfection, increasing access to water, improving sanitation, and investment in and implementation of public health interventions. Among these, the most seemingly straightforward approach—water treatment—is actually far from simple, as Philip Singer, of the ...
Background: Water-borne parasitic infections are caused by pathogenic parasites found in water. These parasites are often not well-monitored or reported, therefore, there is an underestimation of their prevalence. Aims: We systemically reviewed the prevalence and epidemiology of water-borne diseases in the Middle East and North
The parameter \({\beta }_{0}\) estimates the log odds of water borne diseases for the reference group, while \(\beta\) estimates the maximum likelihood, the differential log odds of water borne diseases associated with a set of predictors X, as compared to the reference group, and \(\epsilon\) represents the residual in the model. The variance inflation factor (VIF) ( Additional file -Table-A1 ...
This research is aimed to assess occurrence of water-borne diseases based on personal hygiene and quality of drinking water source. Of the households, 4,237 in 15 selected villages were surveyed ...
We divided total waterborne disease estimates previously reported by structured expert judgment study estimates to produce 2014 burden estimates for ... More research is needed in this area because federal regulations for distribution systems differ from building codes and state and local laws that regulate premise plumbing. In conclusion ...
However, the underlying drivers of exposure and risks are not comprehensively understood, and waterborne diseases continue to pose significant burdens. This study examines the interaction between water quality and human health, focusing on the risk factors for waterborne diseases in communities near the Tano River Basin, Ghana.
Waterborne diseases. have been found in multiple marine mammals and shorebirds, including seals that spend significant time in shared spaces with beachgoers [85-87]. Further research into waterborne disease transmission and exposure risks in marine animals and humans in Florida is warranted.
Protozoan parasites: the invisible menace. The rise of food—and waterborne diseases caused by protozoan parasites, including Cryptosporidium, Cyclospora, Giardia, and Toxoplasma gondii, increasingly threatens food safety.. Cyclospora is a causative agent of gastrointestinal outbreaks, primarily associated with fresh produce such as soft fruits and leafy vegetables.
In Brazil, climate and other human-made environmental changes threaten decades-long efforts to fight schistosomiasis, a widespread and debilitating parasitic disease. Now, researchers have ...
Waterborne diseases that originated due to pathogen microorganisms are emerging as a serious global health concern. ... and parasitic pathogens) in water resources has become a requirement of water quality assessment. Significant research has been conducted to develop rapid, efficient, scalable, and affordable sensing techniques to detect ...
Feature papers represent the most advanced research with significant potential for high impact in the field. A Feature Paper should be a substantial original Article that involves several techniques or approaches, provides an outlook for future research directions and describes possible research applications. ... Optimization of Preparation ...
Microbial waterborne diseases also affect developed countries. In the USA, it has been estimated that each year 560,000 people suffer from severe waterborne diseases, and 7.1 million suffer from a mild to moderate infections, resulting in estimated 12,000 deaths a year [ 6 ].
Feature papers represent the most advanced research with significant potential for high impact in the field. A Feature Paper should be a substantial original Article that involves several techniques or approaches, provides an outlook for future research directions and describes possible research applications. ... Waterborne paint films with ...
Bacterial pathogens are classical etiological agents of waterborne diseases globally. These organisms can occur ubiquitously in many aquatic habitats and humid soils. They are an important part of the biocenosis in various substrates or water systems, especially in their preferred habitats, the biofilms.