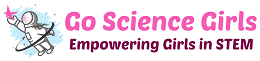

7 Surface Tension Experiments To Try With Kids
- November 2, 2022
- Science Experiments
Here is a list of easy and fun surface tension experiments for kids. These surface tension experiments with water can help kids learn about static water and the forces within it.
Do you love the 4th of July milk fireworks?
What if you can create them using milk?
Have you noticed crazy little balls in your coffee mug while stirring it?
Well, it’s possible to recreate them! There are lots of other factors to know. So, let’s have a look at the seven science experiments that will help to understand physics in a better way while having fun at home.
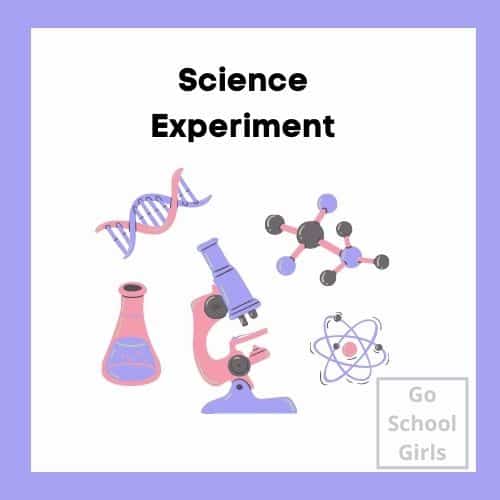
1. Milk Fireworks
This is an easy science activity that needs only a few raw materials and can prove to be a great boredom buster.
Raw materials
- A dish
- Food coloring agents
- Liquid dish soap
Required Steps
- Take the dish and pour some milk into it.
- Now drop a few drops of coloring agents in the middle.
- Now take an earbud soaked in liquid dish soap and dip it into the middle with food coloring agents.
- The colors get scattered in all directions like fireworks!
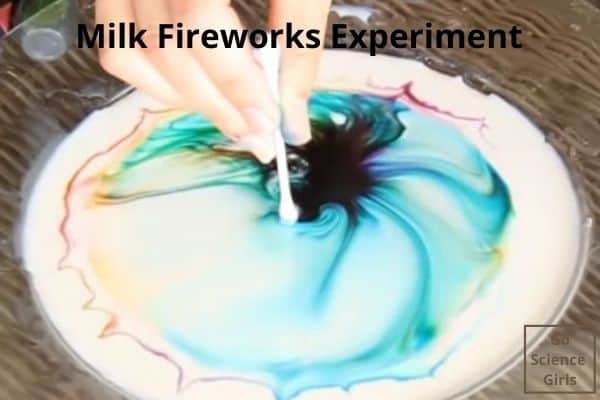
Observation
Experiment Observing that adding little soap to the milk weakens its surface tension by pushing the milk molecules with its hydrophobic ends. Also, the food coloring agents are pushed along with them, and end up having a spectacular sight of fireworks on liquids !
Note: You can conduct this experiment with milk at different temperatures such as warm and very cold to see whether this will make any difference to the behavior of the milk molecules.
2. Water BBS
This experiment demonstrates how crazy little balls notice in the coffee mug while stirring it.
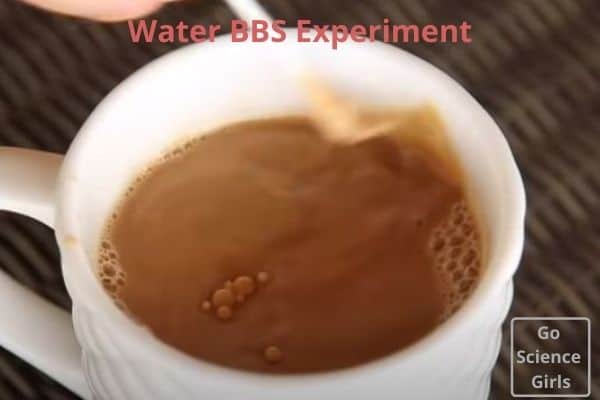
- One cup of coffee
- One coffee stirrer
- Few drops of liquid soap
Required steps
- Take the coffee mug and stir it with the stirrer
- Maybe nothing will happen, and then mix a few drops of liquid soap
In this experiment, notice some little balls in the coffee mug, which are nothing but anti-bubbles. These bubbles are formed when a liquid is dropped turbulently into the same or another liquid.
These are thin films of gas enclosing a sphere of liquid that can appear and then get fully submerged in the liquid.
Unlike ordinary air bubbles, these anti-bubbles do not rise quickly on the top. Patient to see them as they are quite mesmerising.
3. Soap Boat
This science activity video on a soap boat experiment is all about the surface tension of water and the impact of soap on water.
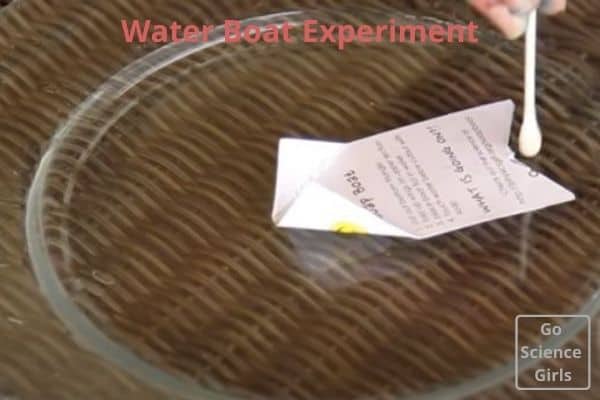
- 1 dish containing water
- 1 little boat with a notch out of a card
- A few cotton buds
- Liquid soap
- Take the dish and place the little paper boat on the surface of the water
- Now, soak the cotton bud in liquid soap and touch its tip into the water to power your paper boat
In this experiment, the boat will start moving swiftly! Now, this happens when you touch the soap on the surface of the water. Soap weakens its surface tension and creates enough force to push the lightweight paper boat. Interesting to notice it!
4. Floating Card
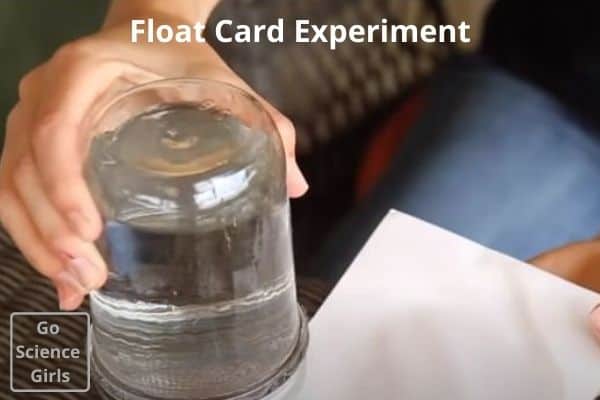
- 1 open jar with a mesh screen on its mouth
- 1 card
- 1 jug of water
- Take the open jar with the mesh screen and pour water into it from the jug
- Now, take the card and place it gently in the mouth of the jar
- Invert the jar, and you will see that it will uphold the card!
- Next, gently remove your hand from the card
- Slid out the card from beneath the jar
- The jar will hold up the water mysteriously!
Observation
Observations help to notice the mysterious water suspension. So, the science behind this floating water trick is nothing but the surface tension across the screen, which holds up the water.
There is also a role of cohesion to play in this science activity. It is the cohesion that causes surface tension. Here, water molecules remain joined together between each tiny opening of the screen mesh and form a thin invisible membrane that is strong enough to hold the water when the jar is inverted.
You can even stick some needles inside the jar! Interestingly, the surface tension will successfully prevent the water from falling in that case too!
You can use this experiment as a magic trick before your friends and can, later on, explain to them the science behind the water suspension.
5. Suddenly sinking paper clips
This science activity video on paper clip floating and sinking is again about the surface tension of water.
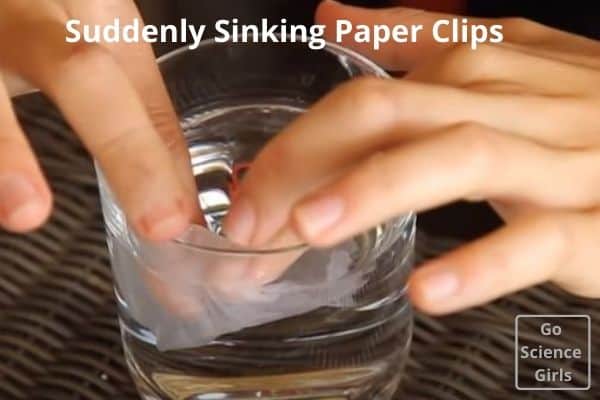
- 1 glass containing water
- 1 paper clip
- 1 piece of tissue paper
- A small quantity of liquid soap
- Take the paper clip and place it on top of the water surface of the glass
- Try to balance it on the water surface
- If it sinks, take it out from the glass
- Now, place the piece of small tissue paper on the water surface and then put the paper clip on it
- Next, gently remove the tissue paper from beneath the paper clip as it will start floating on the water surface
- Now take the Q-tip and soak it in liquid soap and touch its tip into the water
- The paper clip will again sink at the bottom!
Now, wondering why is the paper clip floating on water soap? Well, the reason is again the humble surface tension!
In the second step, then try to make the paper clip float on the water surface, it sinks because the metal with which the clip is made is denser than the water.
However, when placing it on a piece of floating tissue paper, it does not sink because now the surface tension of the water is supporting it.
Again, when you touch the water with soap, this surface tension gets reduced. So, the clip sinks like a brick into the glass.
Also, let’s experiment with this interesting activity with different lightweight objects to see whether the same thing is happening again.
6. Penny Dropper
Have you ever wondered how many drops of water can fit on a penny ?
Well, this super fun science activity will give all the answers.
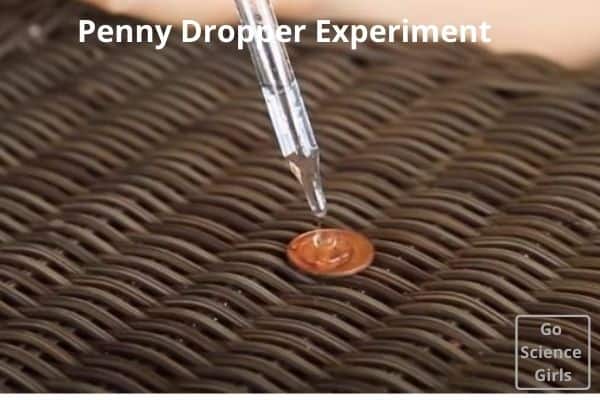
- 1 plastic dropper
- Take the penny and place it on a flat surface
- Now take the dropper, fill it with water, and put a drop of water at the center of your penny
- Keep on adding water drops to the penny and count
- A dome shape made up of water drops will form on the penny
The experiment makes us observe that a penny can hold several water drops before it eventually starts spilling over the coin. Here, it is the surface tension of water that prevents the water molecules from falling apart. So, the water molecules remain together and form a dome shape. Even Experimenting with other liquids such as saltwater, milk, and soapy water to figure out whether they yield the same result or not.
7. Leidenfrost Effect
Have you ever heard about the Leindenfrost effect ?
Well, it is a phenomenon where liquids, instead of getting evaporated, glided on the surface of a pan. This happens when the pan is heated beyond the boiling point of that liquid.
This effect was named after the German doctor Johann Gottlob Leidenfrost (1715-1794), who described this effect.
However, to do this exciting science experiment, you will need adult supervision as this involves heat hazards!
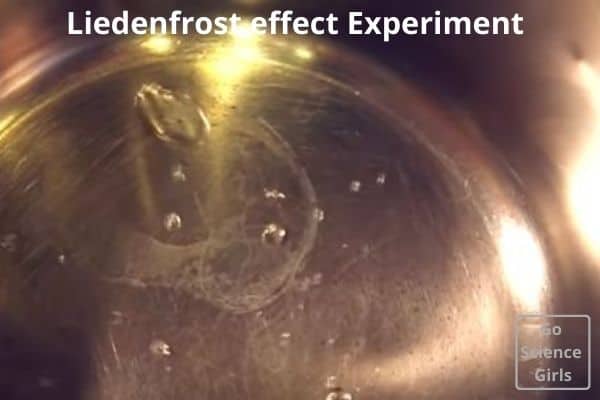
- 1 empty pan
- One dropper
- Take the empty pan and put it on a stove.
- Next, add some water droplets into the pan one by one with the help of the dropper, and the water droplets will quickly evaporate.
- Keep on adding the water droplets but now increase your speed.
- Water droplets will now not evaporate. They will instead make small spheres gliding on the hot surface of the pan.
The observation makes us wonder how does water dance on a hot pan . See, when heating the pan more than the boiling point of water, which is 100-degree Celsius, water drops vaporize quickly that it forms a layer of steam that insulates the rest of the water droplets are added from the hot surface of the pan. As a result, you end up watching the dancing water droplets.
All the above activities can be done at home to develop a better understanding of some key concepts of physics.
Dianna Cowern, also called physics girl presented a video of seven experiments or science tricks that offer surface tension, anti-bubble, cohesion, and lienenforst effect.
Courtesy: Physics Girl
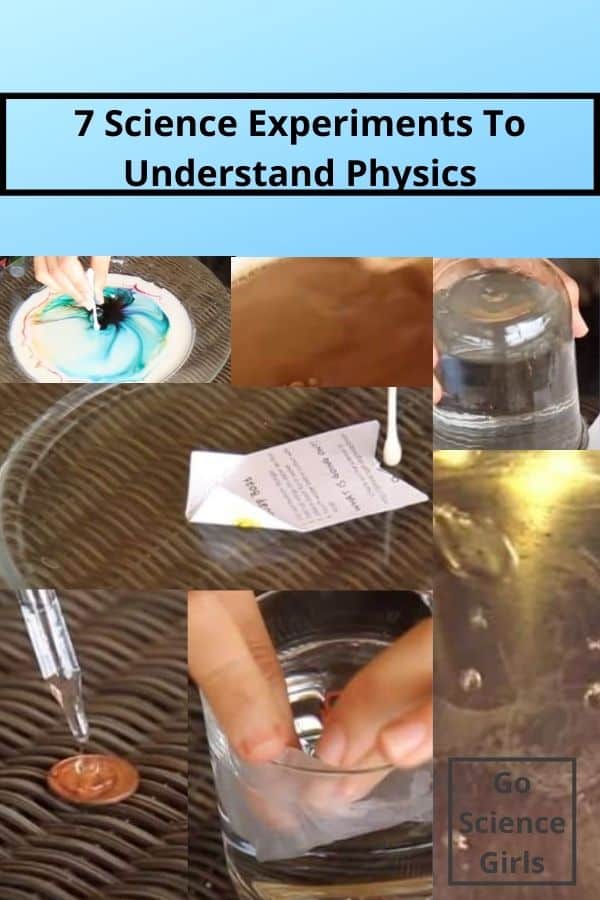
https://www.youtube.com/watch?v=WsksFbFZeeU&feature=emb_logo
https://www.antibubble.org/
https://www.stevespanglerscience.com/lab/experiments/water-screen/
https://msdlt.instructure.com/courses/108/files/2571/download?wrap=1
https://www.rookieparenting.com/how-many-drops-of-water-can-you-put-on-a-penny/
https://www.sociologygroup.com/water-drops-dance-hot-plate/
Leave a Reply Cancel Reply
Your email address will not be published. Required fields are marked *
Name *
Email *
Add Comment *
Save my name, email, and website in this browser for the next time I comment.
Post Comment
Get Your ALL ACCESS Shop Pass here →

Surface Tension Experiments
Learn about the surface tension of water with our simple definition below. Try these fun surface tension experiments at home or in the classroom. As always, you’ll find fantastic and easy-to-do science experiments .

Easy Surface Tension Experiments
Here are some fun ways to demonstrate the surface tension of water. Plus, all you need is a handful of common household supplies. Surface tension is a combination of chemistry and physics . Let’s play with science today!
Magic Pepper and Soap Experiment
Sprinkle some pepper in water and make it dance across the surface. This fun pepper and soap experiment will teach kids about the surface tension of water.
Bubble Snakes
Find out how you can blow up a gigantic bubble snake, all with the help of surface tension.
Drops Of Water On A Penny
A fun science activity with pennies and water. How many drops of water do you think you can get on a penny? The results might surprise you and all because of surface tension!

Floating Paperclip Experiment
How do you make a paperclip float on water? Learn about the surface tension of water with a few simple supplies.
Magic Milk Experiment
Try this color-changing milk and soap experiment. Like water, the dish soap breaks the surface tension of the milk, allowing the food coloring to spread out.

Geometric Bubbles
Explore surface tension while you blow bubbles! Make your own homemade bubble solution too!
Paper Clips In A Glass
How many paper clips fit in a glass of water? It’s all to do with surface tension!
Skittles Experiment
Why don’t the skittles colors mix in water? Explore how surface tension of water makes effects the process. Also set this up with M&Ms.
Soap Powered Boat Experiment
Explore surface tension up close as kids observe firsthand how soap influences the movement of a small boat on the water’s surface.
Bonus Activity: Water Drop Painting
Not an experiment as such but still a fun activity that combines science and art. Paint with water drops using the principle of surface tension of water.

What Is Surface Tension Of Water?
Surface tension exists on the surface of water because water molecules like to stick to each other. This force is so strong that it can help things sit on top of the water instead of sinking into it, like our pepper and soap experiment below.
The high surface tension of water allows a paper clip, with much higher density, to float on water. It also causes drops of rain to stick to your windows and is why bubbles are round. The surface tension of water also helps propel water-striding insects on the surface of ponds.
💡 Also, learn about capillary action !
Scientist Agnes Pockels discovered the science of surface tension of fluids simply by doing the dishes in her kitchen.
Despite her lack of formal training, Pockels was able to measure the surface tension of water by designing an apparatus known as the Pockels trough. This was a key instrument in the new discipline of surface science. In 1891, Pockels published her first paper, “Surface Tension,” on her measurements in Nature.
What is the scientific method?
The scientific method is a process or method of research. A problem is identified, information about the problem is gathered, a hypothesis or question is formulated from the information, and the hypothesis is put to test with an experiment to prove or disprove its validity. Sounds heavy…
What in the world does that mean?!? The scientific method should be used as a guide to help lead the process.
You don’t need to try and solve the world’s biggest science questions! The scientific method is all about studying and learning things right around you.
As kids develop practices that involve creating, gathering data, evaluating, analyzing, and communicating, they can apply these critical thinking skills to any situation.
💡 Learn more about the scientific method and how to use it [here]
Helpful Science Resources
Here are a few resources that will help you introduce science more effectively to your kiddos or students and feel confident when presenting materials. You’ll find helpful free printables throughout.
- Best Science Practices (as it relates to the scientific method)
- Science Vocabulary
- All About Scientists
- Free Science Worksheets
- DIY Science Kits
- Science Tools for Kids
- Scientific Method for Kids
- Citizen Science Guide
- Join us in the Club
More Physical Science Activities
- Gravity Experiments
- Magnet Activities
- Simple Machine Projects
- Potential & Kinetic Energy
- Static Electricity
- Light Experiments
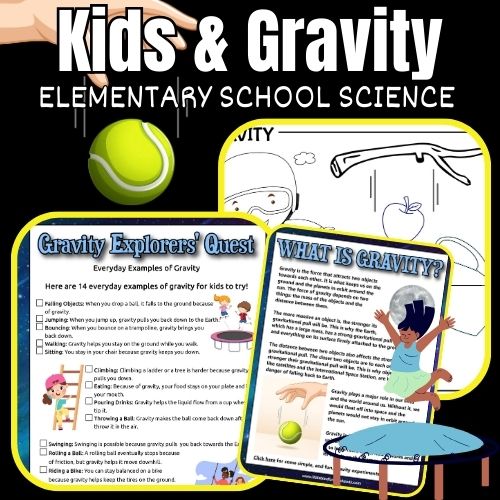
Printable Science Projects For Kids
If you’re looking to grab all of our printable science projects in one convenient place plus exclusive worksheets and bonuses like a STEAM Project pack, our Science Project Pack is what you need! Over 300+ Pages!
- 90+ classic science activities with journal pages, supply lists, set up and process, and science information. NEW! Activity-specific observation pages!
- Best science practices posters and our original science method process folders for extra alternatives!
- Be a Collector activities pack introduces kids to the world of making collections through the eyes of a scientist. What will they collect first?
- Know the Words Science vocabulary pack includes flashcards, crosswords, and word searches that illuminate keywords in the experiments!
- My science journal writing prompts explore what it means to be a scientist!!
- Bonus STEAM Project Pack: Art meets science with doable projects!
- Bonus Quick Grab Packs for Biology, Earth Science, Chemistry, and Physics

Subscribe to receive a free 5-Day STEM Challenge Guide
~ projects to try now ~.


Surface Tension Experiments for Kids
Activities » Science » Surface Tension Experiments for Kids
SHARE THIS POST:

I earn commissions from my affiliated links. Please see my disclosure policy for more details.
Are you looking for simple, yet fascinating science experiments that your kids can do at home? Why not explore the topic of surface tension with some fun activities and experiments? Surface tension is an interesting phenomenon that captures the imagination of even young children.
With just a few materials, they will be able to conduct hands-on experiments to observe and understand surface tension in action.
In this post, we’ll talk about what surface tension is, provide examples of relevant everyday objects as well, and explain four engaging experiments you and your kids or students can try out today! Let’s get started!
As you can probably guess from all my science-related posts lately, my sons are a bit, shall we say, obsessed with science experiments right now. For example, we love the dancing milk experiment and love doing experiments with try ice !
What are 5 examples of surface tension?
Surface tension is the property of a liquid that allows it to resist external forces that could increase its surface area. Here are five examples of surface tension in action:
- Water Striders: Water striders are insects that can walk on the surface of water due to the surface tension of water. Their legs do not break through the surface because of the cohesive forces between water molecules.
- Capillary Action: Surface tension is responsible for capillary action, where liquids can rise or fall in narrow tubes or porous materials against the force of gravity. This phenomenon is seen in plants’ ability to draw water from the soil into their roots.
- Droplets Forming on Leaves: When you see water droplets forming on the surface of leaves or other hydrophobic (water-repellent) surfaces, it’s due to surface tension. The cohesive forces of water cause it to bead up rather than spread out.
- Soap Bubbles: Soap bubbles are held together by surface tension. The soap molecules in the bubble’s film reduce surface tension, allowing the bubble to form and hold its shape.
- Droplets on a Needle: When you touch the surface of water with a fine needle, it can cause water to form a droplet at the tip of the needle. This is due to the surface tension of water trying to minimize its surface area.
Surface tension plays a significant role in various natural phenomena and everyday experiences, as demonstrated by these examples.
Surface Tension Science Experiment for Kids
Science is hands-on and fun for kids. The great part is that kids learn something while doing experiments that they enjoy! With a little bit of knowledge, you can easily bring this learning into your home and classroom.
Surface tension experiments can be both fun and educational for kids. These experiments help children understand the concept of surface tension, which is the property of the surface of a liquid that allows it to resist an external force due to the cohesive nature of its molecules.
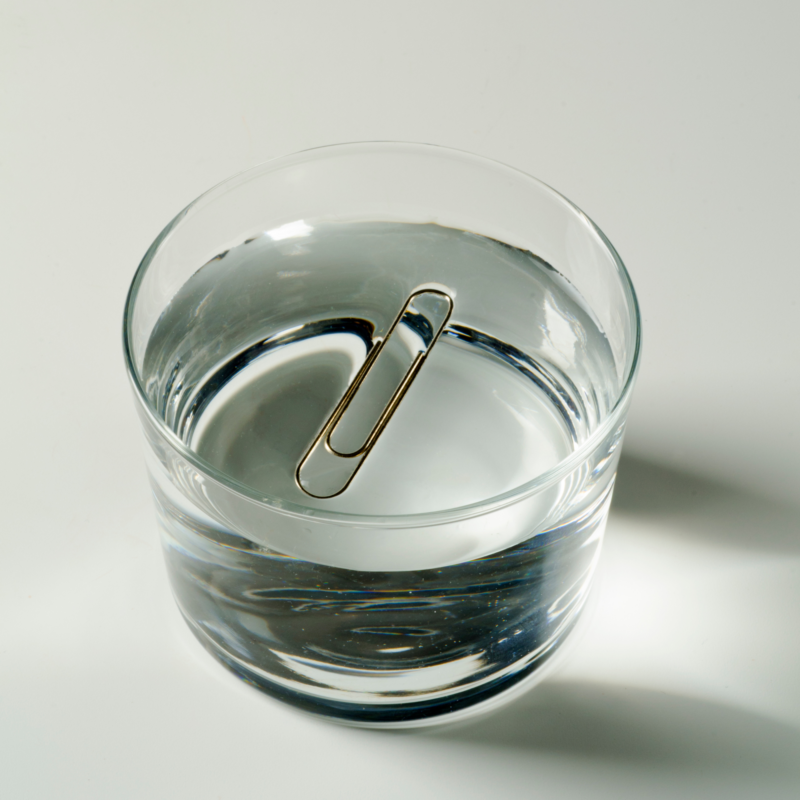
Surface Tension Science Activities
#1 – floating paper clip.
You’ll need a bowl of water, a paper clip, and a tissue or small piece of aluminum foil. Kids can place the paperclip gently on the surface of the water. They’ll observe how the water’s surface tension allows the paperclip to float.
#2 – Magic Milk
For this experiment, you’ll need a shallow dish, milk, food coloring, and liquid dish soap. Pour a small amount of milk into the dish, add a few drops of different food coloring, and then add a drop of dish soap in the center. Kids will be amazed as they watch the colors race to the edges of the dish due to changes in surface tension.
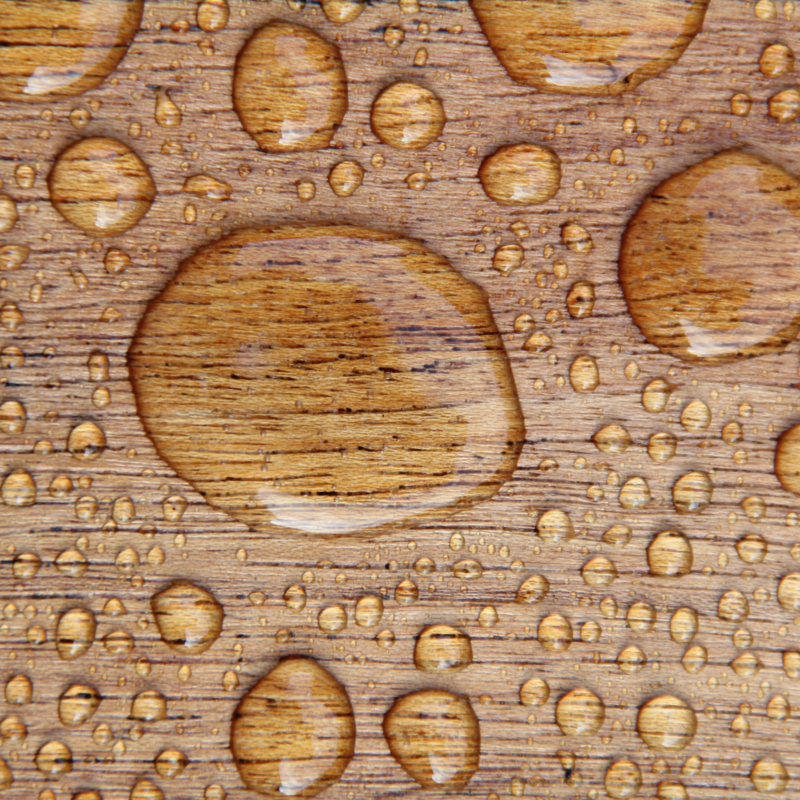
#3 – Floating Needle
Gather a bowl of water and a sewing needle. Slowly and carefully place the needle on the surface of the water. Kids will see that the needle can float on the surface due to surface tension.
#4 – Soap Boat
This experiment requires a small piece of aluminum foil, a bowl of water, and a bar of soap. Kids can cut the aluminum foil into the shape of a small boat and place it in the water. Then, they can carefully touch the soap to the water near the boat, and they’ll observe how the boat moves due to changes in surface tension.
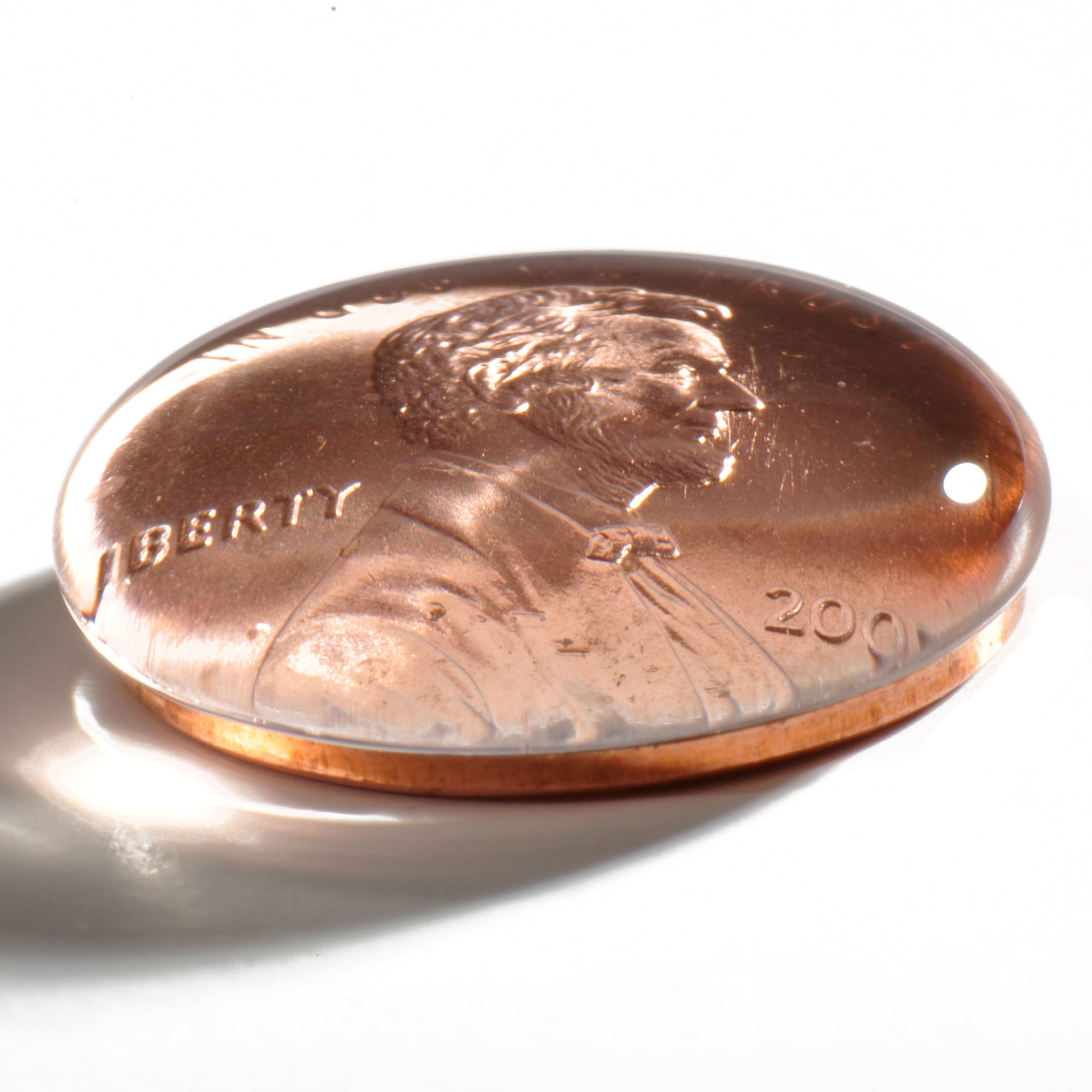
#5 – Water Droplets on a Coin
Kids can place a clean, dry coin on a flat surface and carefully add water droplets one at a time using a dropper. They’ll notice how the water forms droplets and doesn’t immediately spread out due to surface tension.
#6 – Soap Bubbles
Making soap bubbles is a classic surface tension experiment. You’ll need a solution of water and dish soap and a bubble wand or straw. Kids can dip the wand or straw into the solution and gently blow it to create bubbles. They’ll learn how surface tension creates the thin film of the bubble.
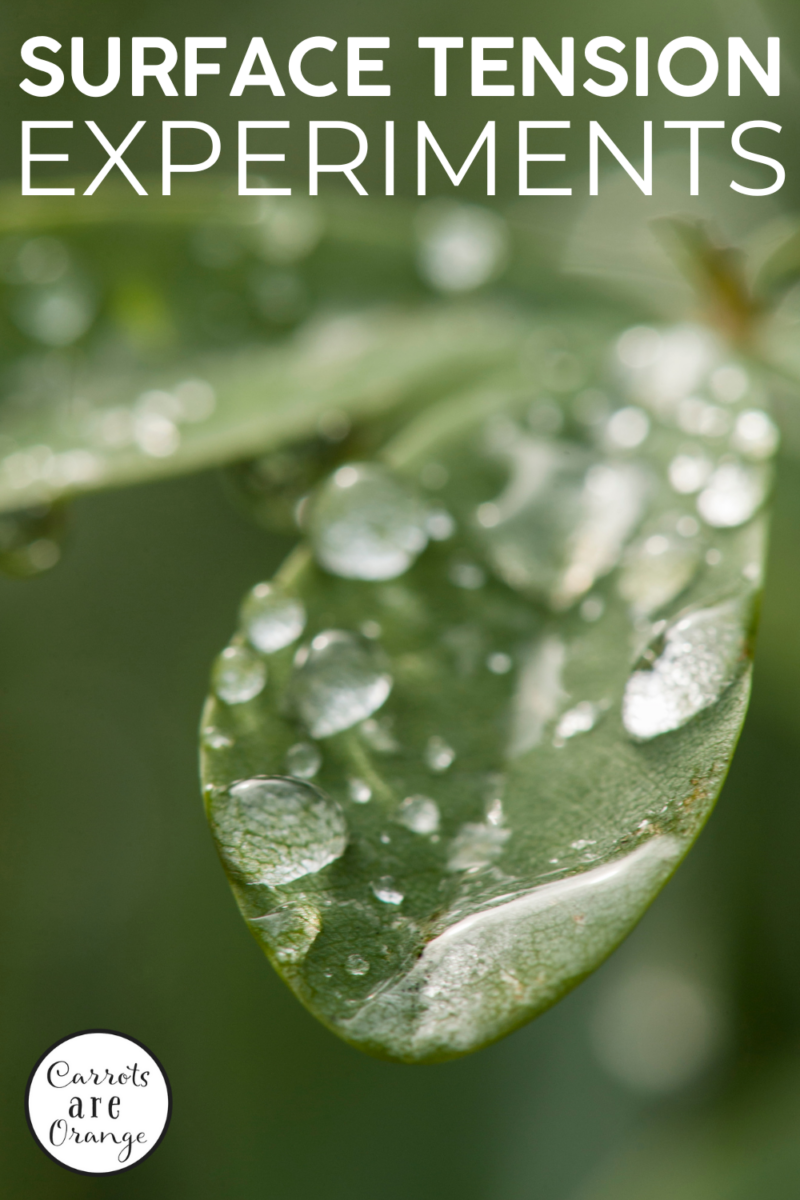
#7 – Floating Pepper
Fill a bowl with water and sprinkle a small amount of pepper on the surface. Kids can then dip their finger, which has a tiny bit of liquid dish soap on it, into the water near the pepper. They’ll observe the pepper moving away from the soap as the surface tension is disrupted.
#8 – Water Bridge
Place two identical cups close together on a flat surface and fill them with water. Use a straw to create a bridge between the two cups by carefully touching the surface of the water in each cup. Kids will see a water bridge forming due to surface tension.
Remember to always supervise kids during these experiments, especially when handling small objects and liquids, and ensure they understand the safety precautions involved. These experiments can help children learn about the properties of water and surface tension in an engaging and hands-on way.
Easy Surface Tension Experiment with Dish Soap
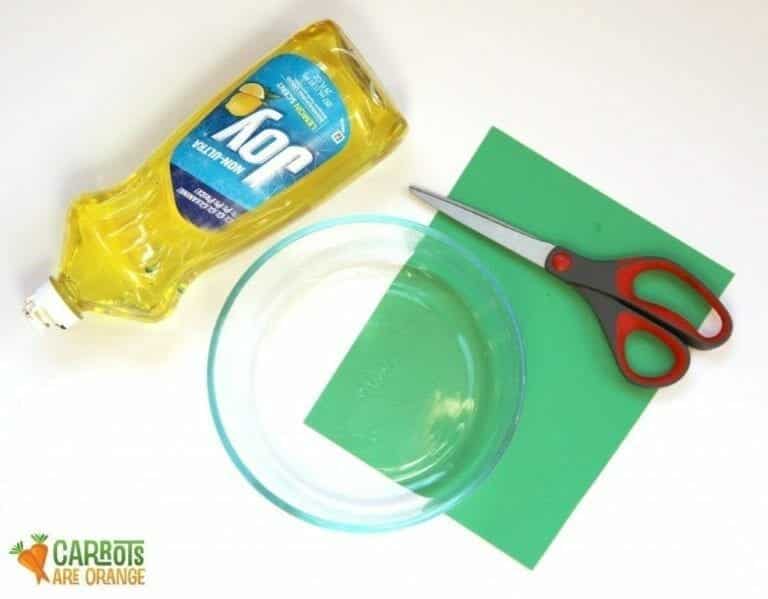
* I used a circular bowl first, then we used a longer Pyrex bowl, which worked better because there was more space for the boat to speed off

- Cut a triangle from the card stock for your “boat”
- Fill your bowl with water*
- Gently place the triangle on top of the water (introduce the concept of surface tension)
- Place a small amount of dish soap on a finger
- Dip the fingertip into the water behind the “boat”
- Observe the boat speed off
- Encourage and ask questions

What is the Science Behind Surface Tension?
Surface tension is a fascinating phenomenon in fluid dynamics that arises due to the cohesive forces between the molecules of a liquid. It’s responsible for several interesting behaviors and effects, such as the ability of small insects to walk on water, the formation of droplets, and the shape of liquid surfaces.
Here’s a brief overview of the science behind surface tension:
- Molecular Cohesion: Surface tension is primarily a result of the cohesive forces between liquid molecules. These forces are primarily caused by intermolecular interactions, such as van der Waals forces and hydrogen bonding, depending on the nature of the liquid. These forces cause molecules at the surface of the liquid to be attracted to their neighboring molecules beneath the surface.
- Unequal Forces: Molecules inside the liquid experience cohesive forces from all directions because they are surrounded by other liquid molecules. However, molecules at the surface do not have molecules above them to balance the cohesive forces. This imbalance in forces results in a net inward force, which acts to minimize the surface area of the liquid.
- Energy Minimization: Nature tends to minimize the energy of a system. In the case of a liquid, minimizing the surface area minimizes the energy associated with the surface. As a result, liquids naturally form shapes that minimize their surface area, which is usually a sphere for small droplets or a flat surface for larger bodies of liquid.
- Mathematical Expression: Surface tension is quantified by a physical property called surface tension coefficient (symbolized as σ), which is measured in units of force per unit length (e.g., N/m or dyn/cm). It represents the amount of force required to increase the length of a liquid interface by a unit amount. The higher the surface tension, the more difficult it is to increase the surface area of the liquid.
- Effects of Surface Tension: Surface tension is responsible for various everyday phenomena, such as the formation of water droplets, capillary action (the rise of liquids in narrow tubes), the ability of small objects to float on the surface of water, and the shape of liquid menisci in narrow containers.
- Contact Angle: When a liquid wets a solid surface, the angle at which the liquid meets the solid is called the contact angle. The contact angle is determined by the balance between adhesive forces (liquid-solid interaction) and cohesive forces (liquid-liquid interaction) and is influenced by surface tension.
- Surfactants: Substances known as surfactants (surface-active agents) can reduce surface tension by interfering with the cohesive forces between liquid molecules. Surfactants are commonly used in detergents and soaps to help break up and remove dirt and grease from surfaces.
Surface tension is a result of the attractive forces between liquid molecules, which causes the surface of a liquid to behave like a stretched elastic membrane. It plays a crucial role in many natural and industrial processes and is a fundamental concept in fluid mechanics.
Water Surface Tension Experiment
Experiment to teach kids about water surface tension.
Posted by Admin / in Matter Experiments
Water has a property we see everyday, but may not know it is there until it is pointed out. It is called water surface tension. If you have ever watched bugs appear to skate on top the surface of the water, they are using water surface tension to move across the water without sinking. Try this surface tension experiment to help to understand why.
Materials Needed
- Small paperclip, Size # 1 (about 1.25" long (3.2 cm))
- Small bowl or cup
- Liquid soap
- Mixing spoon, if needed
WATER TENSION EXPERIMENT STEPS
Step 1: Fill up a cup or bowl of water. Plain tap water works great.
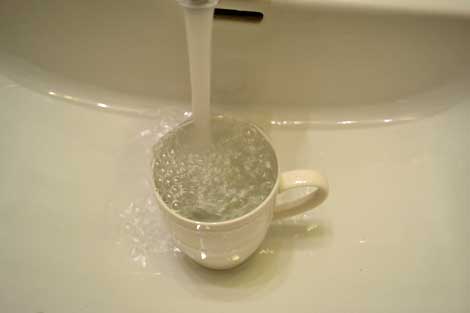
Step 2: Take some metal paperclips and drop them into the bottom of the water to demonstrate how metal paperclips have a density greater than water so they sink.
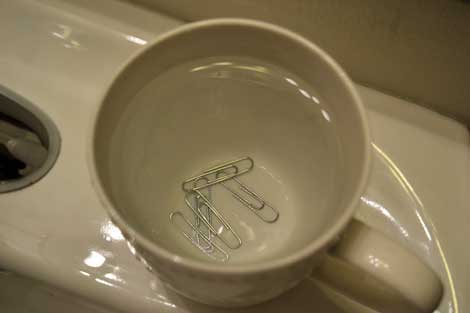
Show how metal paperclips sink in water
Step 3: Carefully place a metal paperclip on the surface of the water. Use a #1 paperclip (they work the best).
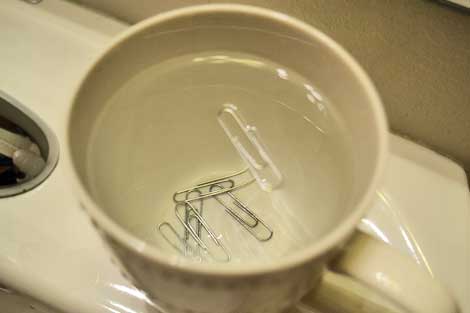
Carefully place the paperclip on the water surface
Step 4: Show the kids how the paperclip now magically floats on the surface of the water.
Step 5: Shake the cup/bowl of water to show that the paperclip is the same as the others and let them see it sinks.
Step 6: Make another paperclip float on the water surface.
Step 7: Now add some liquid soap to the water where the paperclip is floating. What happened?
Step 8 (optional): Try to repeat the experiment using liquids other than water. Do you get the same results?
SCIENCE LEARNED
Water has cohesion that pulls the water molecules together. We can see examples of water cohesion in nature. Water droplets form to make rain. If you pour water over a car windshield it groups together to form drops and streams of water. Water joins together to form streams, rivers and oceans. Water in soil helps that soil stick together so if you dig a hole, it stands up for a while. If soil did not have cohesion then everytime the wind blew hard, it would result in dust storm. Without the cohesive forces of water our world would be much different.
In the experiment we start with showing the kids that paperclips are heavier than water and they sink right to the bottom. When they are carefully placed at the water surface, however, the paperclips float. There is an added force at the surface of the water that is not present under the surface of the water. It is called surface tension. The surface tension is in fact the cohesion of the water pulling it together. Since all of the water is below the surface and their is no water above it there is internal pressure created in the water at the surface. This acts like a skin that helps the paperclip float on the water surface.
What happened when the soap was added? Why did the paperclip suddenly sink? Simple. The soap reduce the cohesion force in the water, resulting removing the "skin" from the water surface.
- About the author
- Back to Experiment
Please select the social network you want to share this page with:
We like you too :)
Thanks for taking time to give us feedback!
- Matter experiments
- science experiments for kids
- water experiments for kids
- liquid experiment for kids
- teaching kids about liquid
- liquid science
- water surface tension
- buoyancy experiments for kids
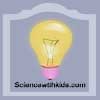
posted by Admin
- previous experiment
- next experiment
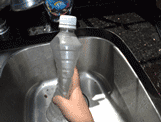
Air Pressure Experiment
in Matter Experiments
Simple Experiment with a good visual demonstration of changing air pressure.
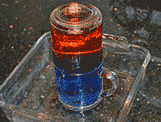
Hot and Cold Water Density
Use this simple experiment to demonstrate hot and cold water density..
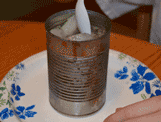
Water Cycle Experiment
Experiment to show all the phases of the water cycle.
- Skip to main content
- Skip to primary sidebar
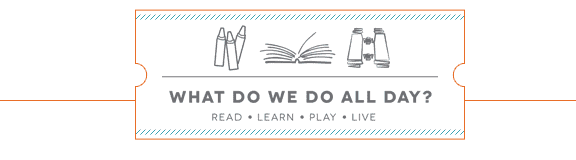
Easy Science: Surface Tension Water Drop Races
Science experiments don't need to be complicated! A water drop race is a simple science project is a great was to pass the time when you need a quick distraction for your kids. It can be part of an in-depth classroom exploration into the concept of surface tension and molecule cohesion.
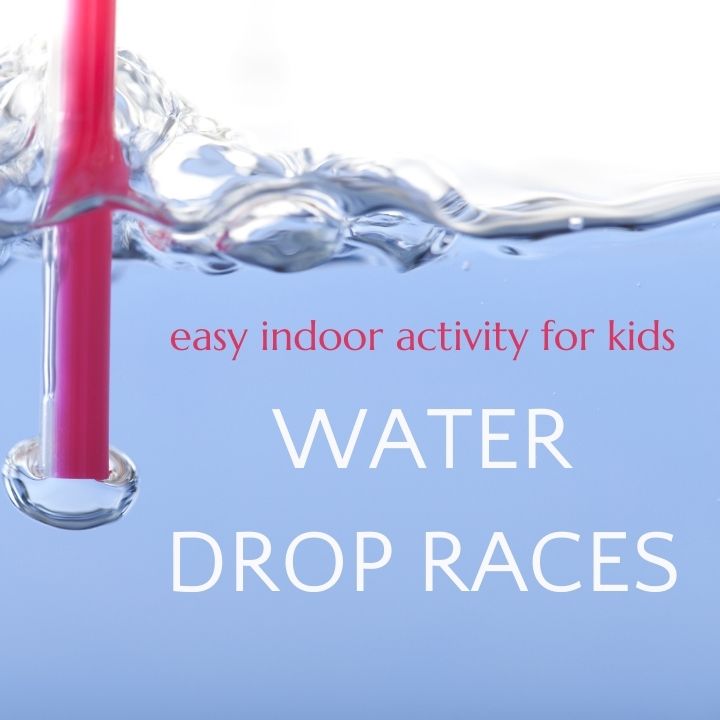
What is Surface Tension?
Surface tension is
the property of the surface of a liquid that allows it to resist an external force, due to the cohesive nature of its molecules. The cohesive forces between liquid molecules are responsible for the phenomenon known as surface tension. The molecules at the surface of a glass of water do not have other water molecules on all sides of them and consequently they cohere more strongly to those directly associated with them. USGA
A classic kids surface tension experiment is the one where you drip water drop by drop onto a penny. You can also observe surface tension by floating a paper clip on the surface of water.
Bugs also use surface tension to hang out on ponds and streams. Leaves during a rain storm also demonstrate water's cohesive nature.

For this water drop race experiment, surface tension is responsible for the spherical shape of the water droplets. The waxy paper keeps the water from being absorbed away by the surface they sit on.
However, even if your child is too young to grasp the concepts of surface tension and the bonding properties of water molecules, it's still a super fun indoor activity that will make kids say, "Cool!!!"
Water Drop Surface Tension Experiment
How to conduct water drop races
You will need: one straw per scientist glass of water water dropper wax paper or parchment paper

Observations
Kids will start to notice ways in which they can control their water drops. Young scientists can explore surface tension strength by blowing air through the straw extra hard, which will cause larger water drops break up into smaller water beads.
Kids will also learn how to blow water beads together to merge them into larger droplets. No doubt, they will have fun sucking water drops up the straw and blowing them back out! My kid loved blowing his off the table to "plop" on the floor! ( It's just water, after all. )
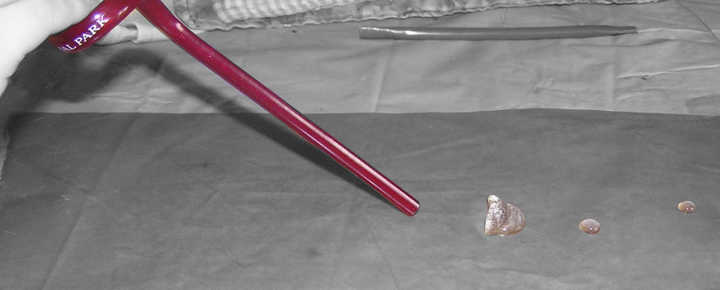
Conclusions
Whether or not you use this surface tension experiment to go into depth about how water molecules, tension and cohesion work, water drop races provide lots of entertainment!
I absolutely loved how this project has the added bonus of keeping the kids busy for an extended period of time without a lot parental involvement. And because it's just a small amount of water that is used, you won't have to worry about everything getting wet or the kids making a big mess to clean up!
More water experiments
- Water refraction is just like magic!
- Amaze your kids with a leak proof hole in a bag!
- Make a coin jump off a bottle
- All our favorite indoor water activities
I First Published this idea 3/16/09.

Reader Interactions
Leptir says
June 04, 2010 at 9:14 am
Looks like fun 🙂 I'll probably try it in my classroom with kids. Thanks for sharing 🙂
MaryAnne says
June 04, 2010 at 12:15 pm
What an excellent activity! Thanks for the idea =)
June 05, 2010 at 1:44 am
Christy says
June 05, 2010 at 5:33 pm
My kids will love this! Thanks.
Raising a Happy Child says
June 09, 2010 at 11:14 am
I have to try it out - wind races have been popular here, but we haven't done them with water.
September 12, 2016 at 5:29 pm
Hi! I'm about to launch a year-long science course for young kids at some of the local schools. I love this idea! It will be a PERFECT fun activity to end the "Surface tension" lesson with! We'll be doing some fun experiments to bring the concept home to the kids, and this is just what I needed! Thanks so much Erica!
September 14, 2016 at 12:16 pm
Glad the idea was useful!
Julie C Billow says
April 17, 2017 at 12:45 am
I just tried this out myself and had fun! Try adding some color to the experiment by drawing on the waxed paper with markers and then watch as the water drops blow through the colors and absorb them. Fascinating!
April 19, 2017 at 11:07 am
I love that idea!
March 09, 2018 at 3:22 pm
Used this today with my preschool kids. Many had fun watching the water break apart and go back together. I added food coloring to the water and used freezer paper ??
Rebecca says
March 28, 2019 at 3:00 pm
We used color waters and blew the the color water droplets into each other to see what colors they turned into. The kids loved it.
March 29, 2019 at 8:08 am
What a fantastic idea!
Leave a Reply Cancel reply
Your email address will not be published. Required fields are marked *
This site uses Akismet to reduce spam. Learn how your comment data is processed .
- Skip to primary navigation
- Skip to main content
- Skip to primary sidebar
- MEMBER LOGIN
Hands On As We Grow®
Hands on kids activities for hands on moms. Focusing on kids activities perfect for toddlers and preschoolers.
Magic Finger Pepper Experiment for Kids
Learning Science Kindergartners Preschoolers Toddlers Experiment Kitchen Water Activities 4 Comments
Alisha shares this super simple surface tension science experiment for kids will leave your kiddos amazed!
In my home, we call this the Magic Finger trick. It’s a great way to get your toddlers, preschoolers, and school-aged kiddos interested in the wonders and joys of science.
Best part – there’s no setup!
Here are 30 other great experiments to do with your preschooler!
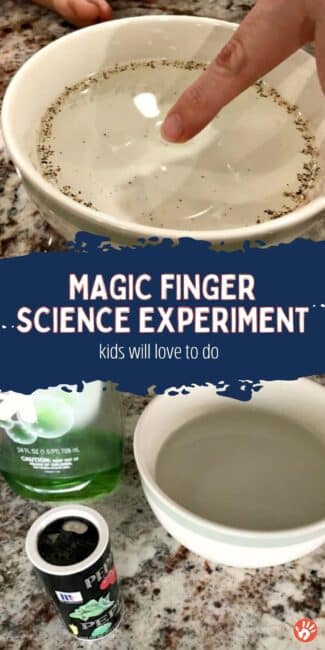
You only need three supplies for this surface tension experiment; all of the items are found in your kitchen.
- A bowl of water
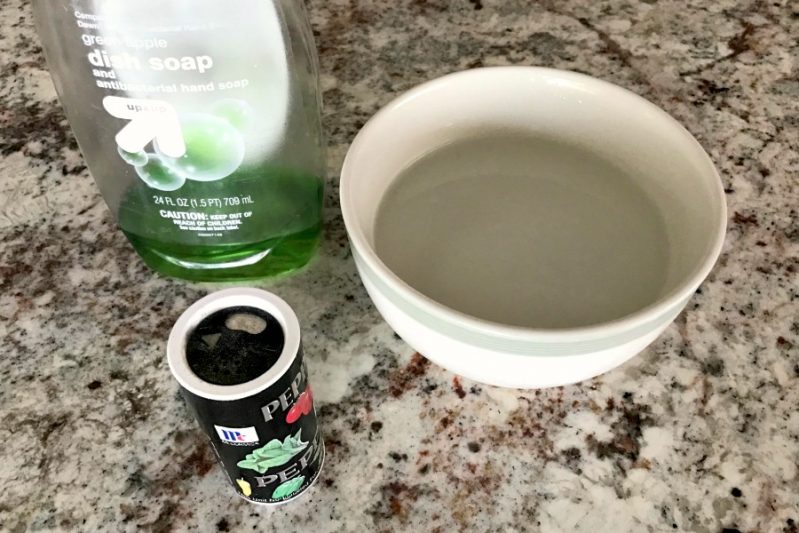
Begin by grabbing a bowl from your cabinet and fill it up with water at least half way.
Next, sprinkle some pepper into the bowl of water. Use enough pepper to cover the top of the water.
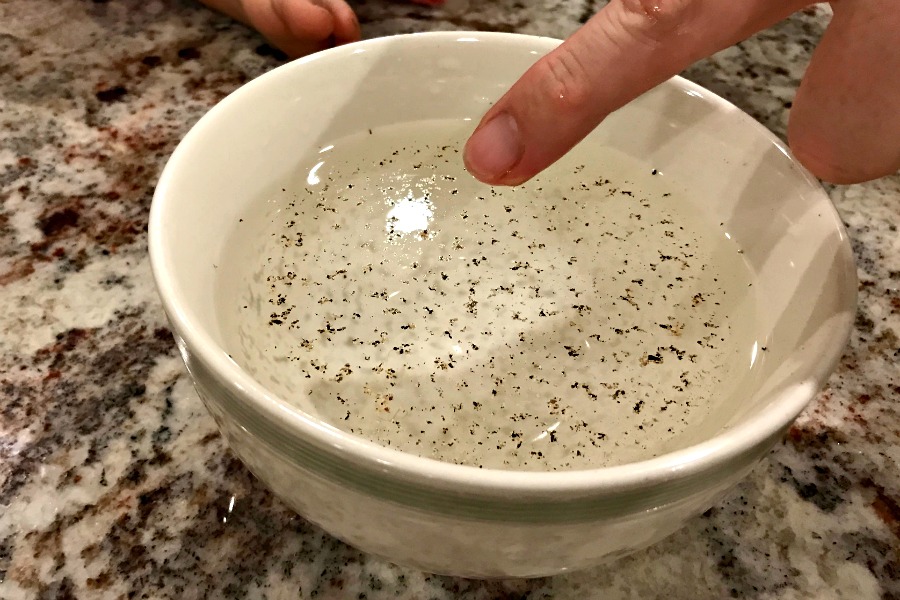
Then, prepare your kiddos for the amazement that is about to occur.
Simply put some dish soap on the tip of your finger and touch the middle of the water.
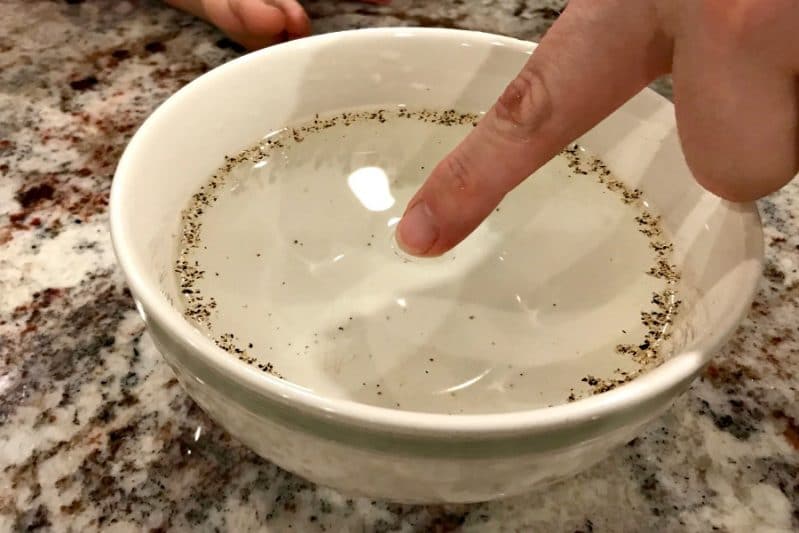
Your little ones might think it is magic…
…but in fact there is real science behind the experiment.
When the soap touches the water, the surface tension changes, and the pepper no longer floats on top. But the water molecules still want to keep the surface tension, so they pull back away from the soap, and carry the pepper along with them.
After doing the experiment and talking about surface tension, let your kiddos take a turn using their magic finger.
Here are some great water activities for toddlers!
An extension of this surface tension experiment for kids would be to try other objects on the water.
- Would rice react the same way as the pepper?
- Would pom poms (affiliate link) be too big to react to the surface tension change?
Did you find any other items that reacted the same way as the pepper?
About alisha warth.
I have raised my children doing activities with them. As a homeschool mom, I am always looking for ways to make our learning fun. I'm honored to be able to contribute my ideas to the awesome site that is Hands On As We Grow.
More Hands on Kids Activities to Try
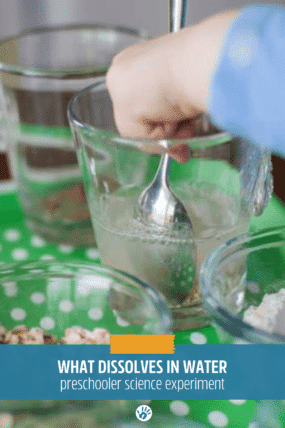
Reader Interactions
sharon Klass says
January 10, 2019 at 12:07 pm
Hi, I haven’t tried this with kids yet but I had so much fun doing it myself I know my kiddoes will too. I thank you so much for the seven day challenge, I’m so excited about all the activities and the suggestions. Thank you Sharon K.
August 19, 2018 at 7:32 pm
Thank you foe sharing your great ideas. Its very helpful.
Leave a Comment Cancel reply
Your email address will not be published. Required fields are marked *
This site uses Akismet to reduce spam. Learn how your comment data is processed .
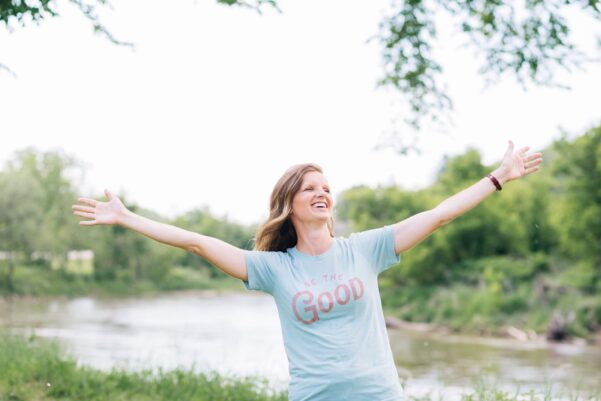

What Parents Have to Say…
Shop ebooks of activities.
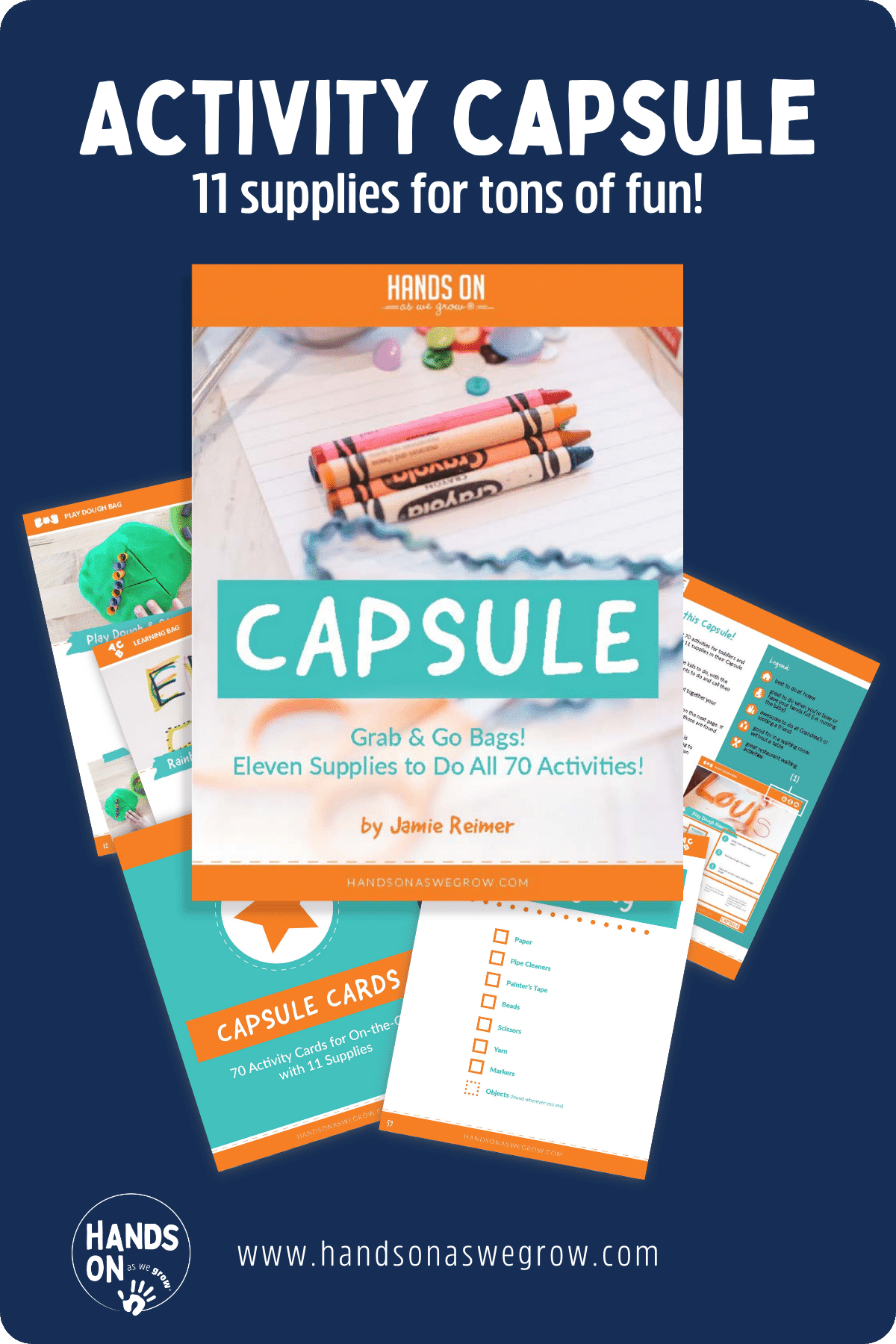
Get activity plans delivered to your inbox, every week!
Activities that hands-on parents absolutely love.
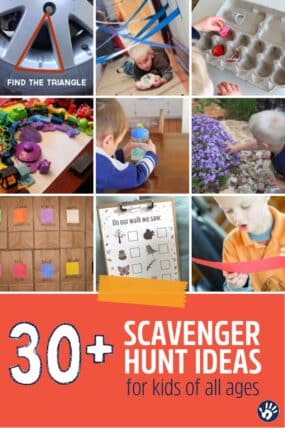
30+ Scavenger Hunt Ideas for Kids To Do at Any Age
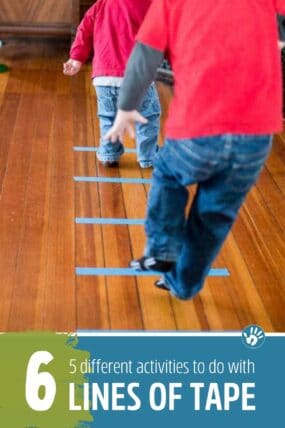
6 Different Activities for 6 Lines of Tape
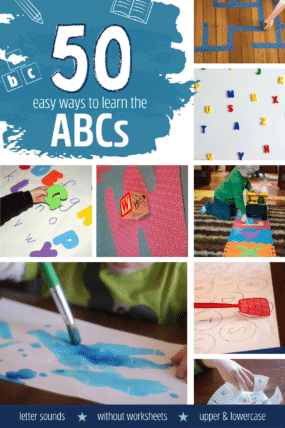
50 Simple & Fun Alphabet Activities for Preschoolers
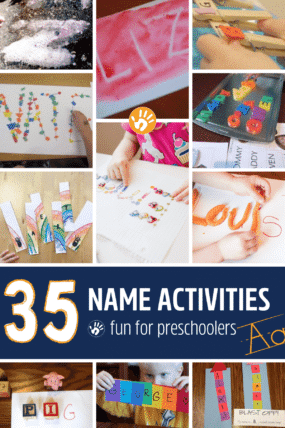
35 Name Activities For Preschoolers
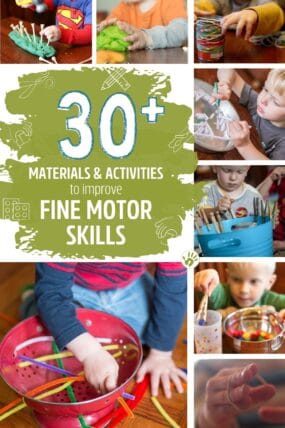
Improve Kids Fine Motor Skills with 30 Materials & Activities
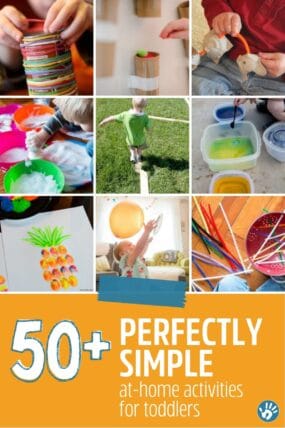
50+ Simple Activities for Toddlers
Get started having fun with your kids.
PLAN THE FUN WITH THE FREE KIDS ACTIVITIES PLANNER! AND RECEIVE ACTIVITIES EVERY WEEK!
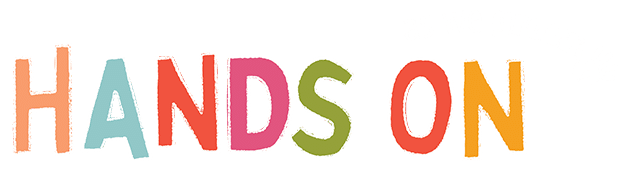
- Preschoolers
- Kindergartners
- Grade School
- Literacy & ABCs
- Math & 123s
- Art Projects
- Gross Motor
- Shop Activity Plans
- Member Login
- Skip to primary navigation
- Skip to main content
- Skip to primary sidebar
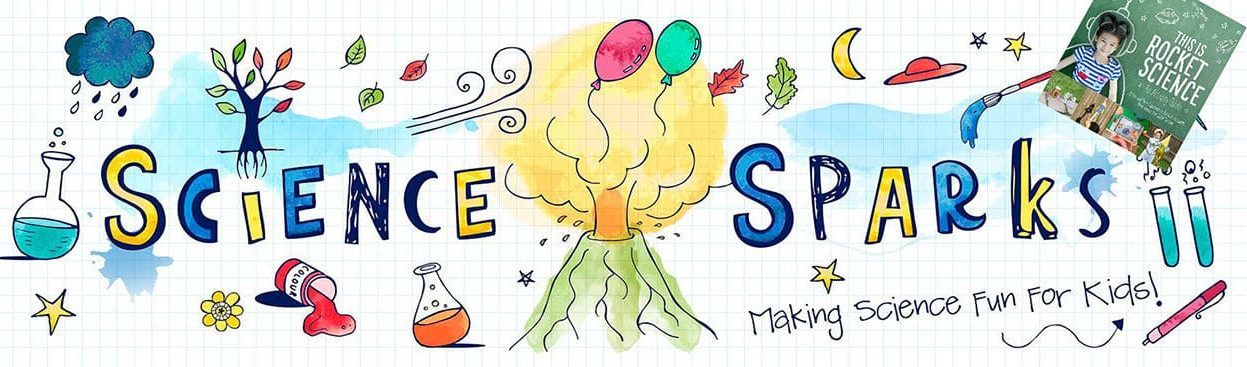
- FREE Experiments
- Kitchen Science
- Climate Change
- Egg Experiments
- Fairy Tale Science
- Edible Science
- Human Health
- Inspirational Women
- Forces and Motion
- Science Fair Projects
- STEM Challenges
- Science Sparks Books
- Contact Science Sparks
- Science Resources for Home and School
Super Simple Surface Tension
August 7, 2013 By Emma Vanstone 3 Comments
Do you remember our lolly stick races ? They are a great way to explore surface tension, but this activity demonstrating surface tension with toothpick s is even easier!
The toothpicks start off in a triangle shape and then fly apart when a little washing-up liquid is added.
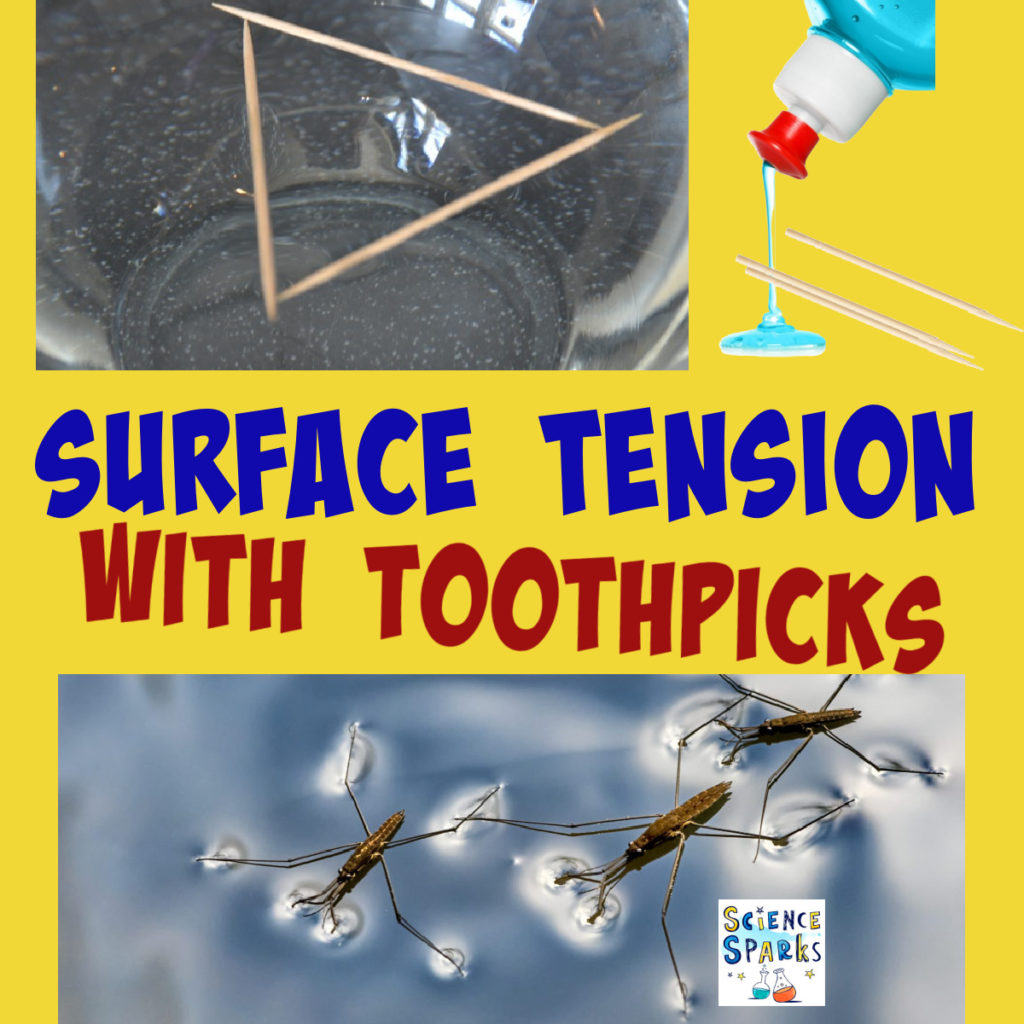
Surface tension activity with toothpicks
You’ll need
- Cocktail sticks or toothpicks
- A clean bowl
- Washing up liquid /dish soap
- A large bowl
Instructions
- Fill the bowl with water and let it settle for a few minutes.
- Carefully place the cocktail sticks on the top. Try and get them to form a triangle.
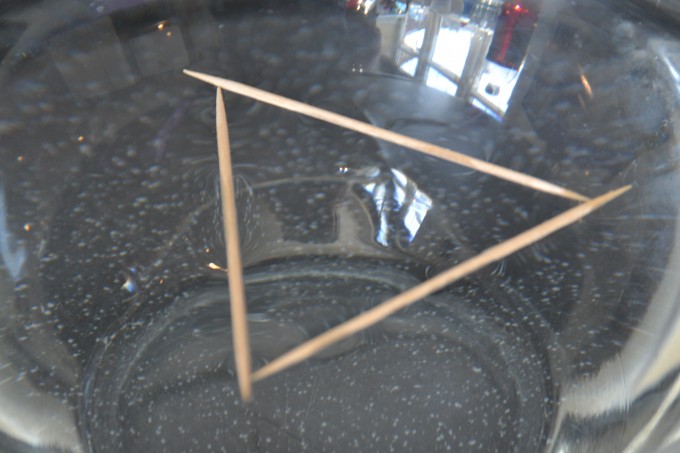
- Add a drop of washing-up liquid, and watch what happens.
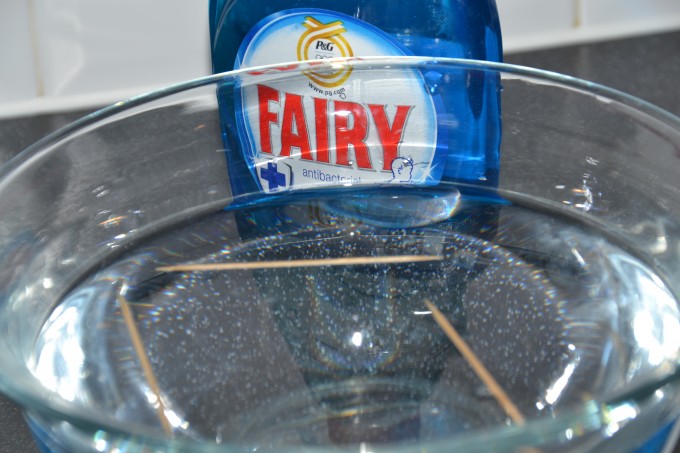
Why does it work? Surface tension explained!
This activity is a very simple demonstration of surface tension. When you have a container full of water, the water molecules below the surface are pulled together equally in all directions, but those on top are pulled together more tightly, as they don’t have water molecules above them. This draws them together to form a kind of ‘skin’ that we call surface tension . When the washing-up liquid is added, it disrupts the arrangement of the water molecules, decreasing the surface tension inside the triangle of toothpicks.
Water molecules move from areas of low surface tension to high surface tension, and so the sticks move.
Don’t worry if that explanation seems too much for your child. I don’t always explain the full scientific principles to my children, but watching and taking part in activities like these really does encourage children to start to question why things happen, and the concepts will start to make more sense to them as time goes on.
More surface tension experiments
Try racing lolly sticks across a bath or large container of water.
Raising Lifelong Learners has a great activity for making a paperclip float using surface tension.
Watch food colouring shoot across a tray of milk .
If you enjoyed this investigation, why not try one of my other easy science experiments !
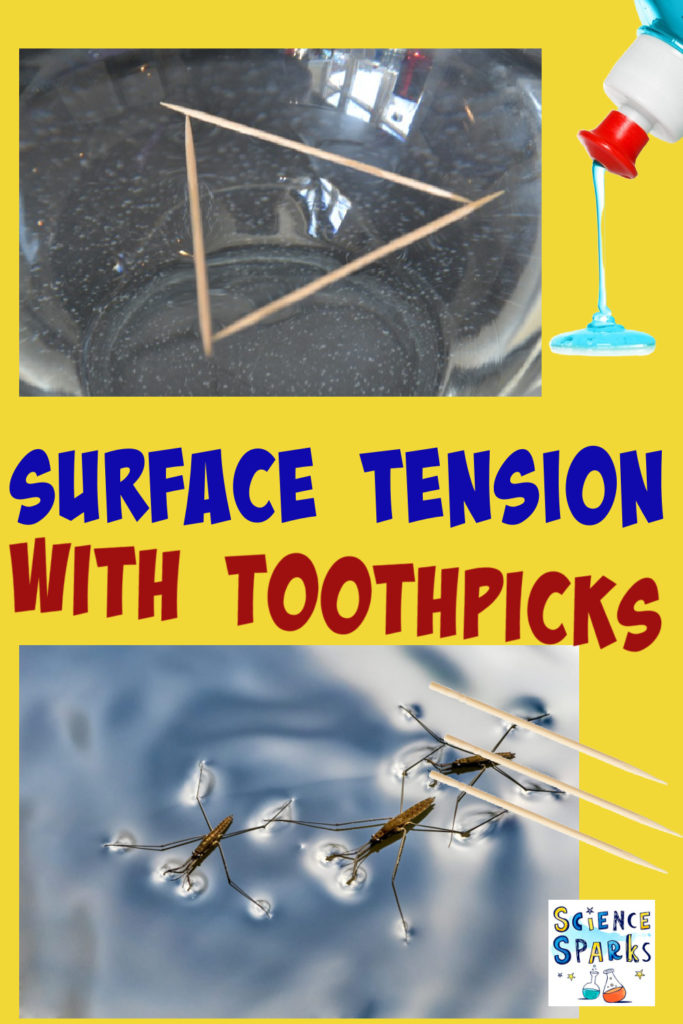
Last Updated on September 19, 2023 by Emma Vanstone
Safety Notice
Science Sparks ( Wild Sparks Enterprises Ltd ) are not liable for the actions of activity of any person who uses the information in this resource or in any of the suggested further resources. Science Sparks assume no liability with regard to injuries or damage to property that may occur as a result of using the information and carrying out the practical activities contained in this resource or in any of the suggested further resources.
These activities are designed to be carried out by children working with a parent, guardian or other appropriate adult. The adult involved is fully responsible for ensuring that the activities are carried out safely.
Reader Interactions
Leave a reply cancel reply.
Your email address will not be published. Required fields are marked *
Testing Surface Tension
Surface tension is why feathers, canoes, and even snakes can float on top of the water. But what about the surface tension of other liquids? Or what happens when you add soap to water? Let’s find out!
HYPOTHESIS: When the surface tension of liquids is changed, it changes a liquid’s ability to allow objects to float.
Supplies needed:
Here is what you do:, how many pebbles can the bowl hold until it sinks, ask yourself:, conclusion:, find out more: .
How is it possible for people to float on top of water AND sink or drown?
- Earth Science
- Physics & Engineering
- Science Kits
- Microscopes
- Science Curriculum and Kits
- About Home Science Tools
Science Projects > Physics & Engineering Projects > Water Experiments
Water Experiments
Surface tension experiments.
Surface tension is one of the most important properties of water .
It is the reason that water collects in drops, but it’s also why plant stems can “ drink water ,” and cells can receive water through the smallest blood vessels.
You can test multiple surface tension experiments using just a few household items.
What You Do:
1. Start with a cup of water and some paperclips. Do you think a paperclip will float in the water? Drop one in the cup to find out. Since the paperclip is denser than the water, it will sink to the bottom of the cup.
Now find out if you can use surface tension to float the paperclip. Instead of dropping the paperclip into the cup, gently lay it flat on the surface of the water.
(This is tricky — it may help to place a piece of paper towel slightly bigger than the paperclip in the water. Then lay the paperclip on top of it. In a minute or so, the paper towel will sink, leaving the paperclip floating on top of the water.)
2. Even though the paperclip is still denser than the water, the strong attraction between the water molecules on the surface forms a type of ‘skin’ that supports the clip.
3. Now put a drop of dish soap in the water. This will bind with the water molecules, interfering with the surface tension .
The paper clip will sink. You can try floating other things on top of the water also – pepper floats well until you add dish soap. Can you find any other light items that will float?
Surface tension creates the ‘skin’ on top of the water, but it is also what causes water to stick together in drops.
Observe how these drops stick together by experimenting with water and a penny. All you need is a cup of water, a penny, and a medicine dropper .
First make a prediction: how many drops of water do you think you can fit on the top surface of the penny? Add one drop. After seeing how much room it takes, do you want to rethink your first prediction?
Now continue carefully adding drops until the water spills off the penny. Try this three times, recording the number of drops each time, and then find the average number of drops that can fit.
Surface tension is the reason you can fit so much water on the penny. The water molecules attract each other, pulling together so the water doesn’t spill.
Try this experiment with different-sized coins. Predict how many drops you can fit on a quarter compared with the penny.
For one final surface tension experiment, start with a full glass of water. Predict how many pennies you can add to the water without the glass overflowing. Gently add pennies one by one. Because of surface tension, the water will rise above the rim of the glass before it spills! Compare your original prediction with the number of pennies you were able to add.
Freezing Point
Have you ever wondered why rivers and lakes freeze in the winter, but oceans do not? In this experiment we will see that it is the presence of salt in the ocean that makes it less likely to freeze.
What You Need:
- 1-gallon freezer bag
- 1-quart freezer bag
- crushed ice
- thermometer
1. Fill the gallon freezer bag half full with crushed ice. Add one cup of salt and seal the bag. Put on some gloves and knead the ice and salt until the ice has completely melted.
2. Use the thermometer to record the temperature of the saltwater mixture. Even though the ice has melted, the temperature should be less than 32°F (0°C).
3. Now put about an ounce of water in the quart freezer bag. Seal the quart bag and then put it in the saltwater mixture in the larger bag. Seal the larger bag also and leave it until the water inside the quart bag freezes.
How did the water freeze when surrounded only by saltwater?
The salt broke apart the bonds between the water molecules in the ice, causing it to melt, but the temperature remained below the freezing point for pure water.
Salt (and other substances dissolved in water) will always lower the freezing point .
This is why water in the ocean rarely freezes.
- Find out more about salt water by making a Solar Purifier
More Water Projects:
- Liquid Density
- Hot Water: Convection Science
- Water Wheel
Physics & Engineering
Welcome! Read other Physics & Engineering related articles or explore our Resource Center, which consists of hundreds of free science articles!
Shop for Physics Supplies!
Home Science Tools offers a wide variety of Physics products and kits. Find physics & engineering tools, equipment, STEM kits & more for kids and adults.
Related Articles

Homopolar Motor – Make a Spinning Wire Sculpture
In this experiment, we will make a homopolar motor! To make a simple motor (homopolar motor) that doubles as a work of art you will need three things – a battery, magnet, and wire. Use one of our neodymium magnets to power the spinning wire motor. What You Will Need:...
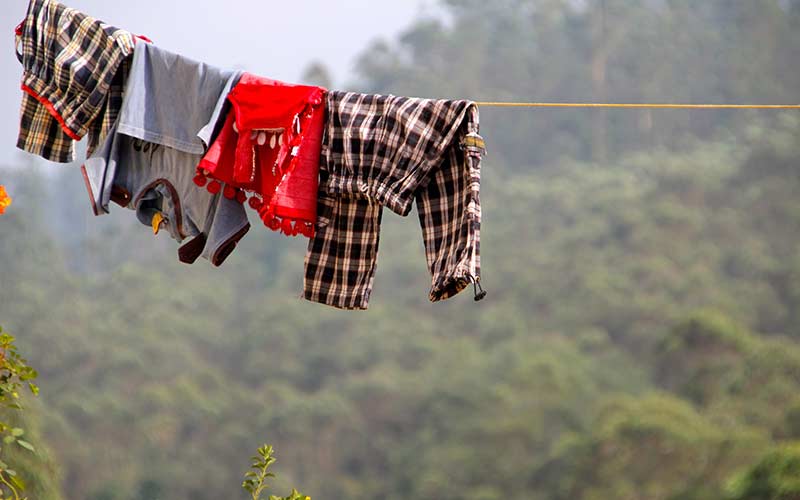
Solar Energy Matching Game
Print out this page on a sheet of heavy paper or cardstock. Kids can color the pictures and cut out the squares to make a matching game. Half of the squares show a way to use solar energy as an alternative to the picture shown on the other squares. Place all the...

Simple Spring Break Science Projects
Spring break is here! What will you do with your time off? Perhaps you're looking forward to a family vacation, or a few days of down time at home. Either way, find a quick and easy project that's sure to put a sparkle in the eye of any science lover, or win over a...
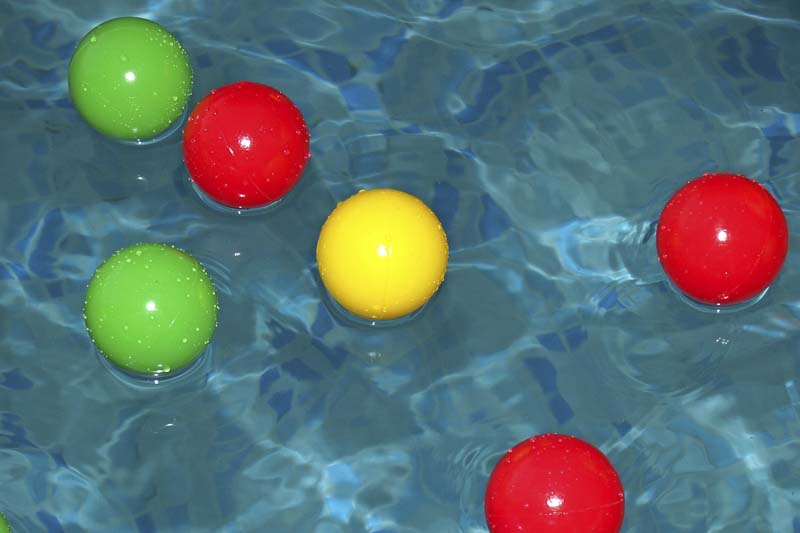
Sink or Float Worksheet
Use the Sink or Float Worksheet with the "Sink or Float?" science project to encourage kids to make predictions, perform tests, and record their results. This project is perfect for indoor discovery - especially in colder months! Put a towel under a large container of...
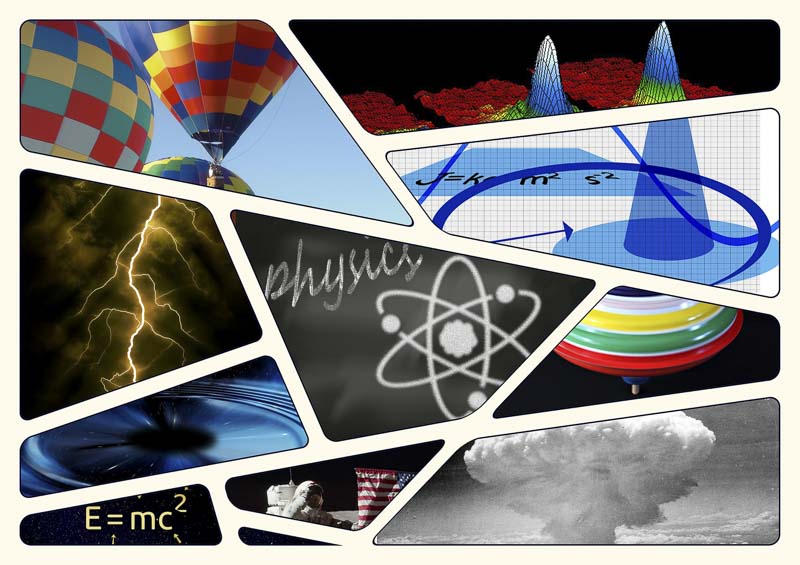
Physics Science Fair Projects
Find physics science fair project ideas about magnetism, electricity, energy and solar power, and more.
JOIN OUR COMMUNITY
Get project ideas and special offers delivered to your inbox.
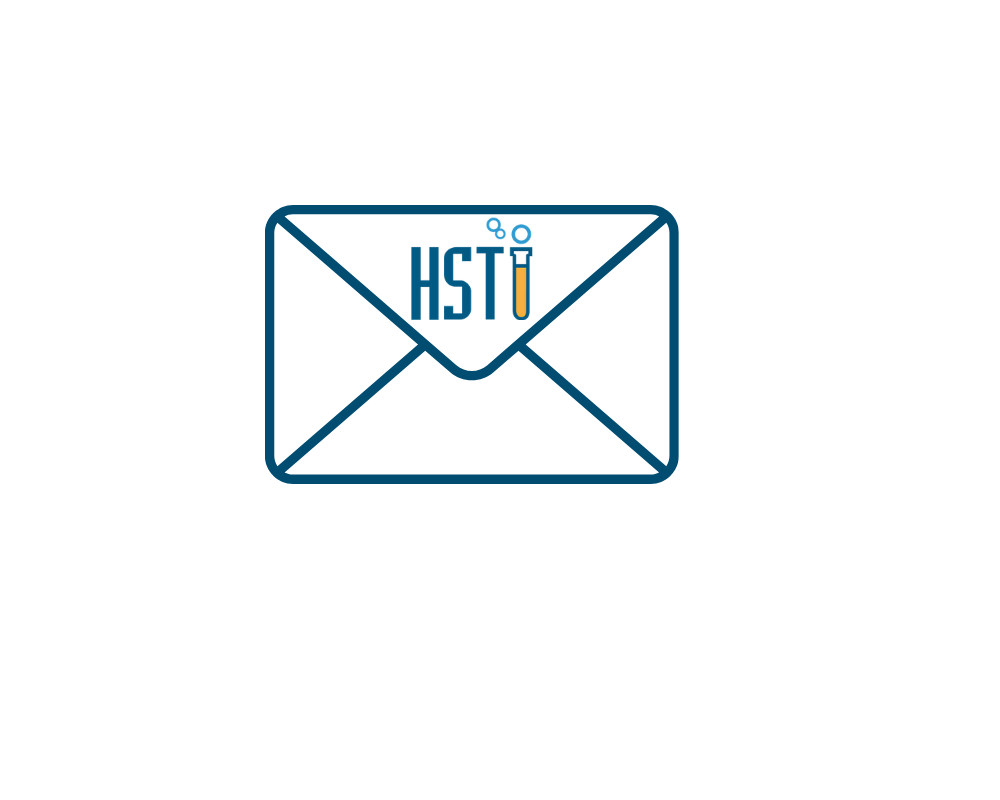
20 Experiments With Tension Water

Introduction: 20 Experiments With Tension Water
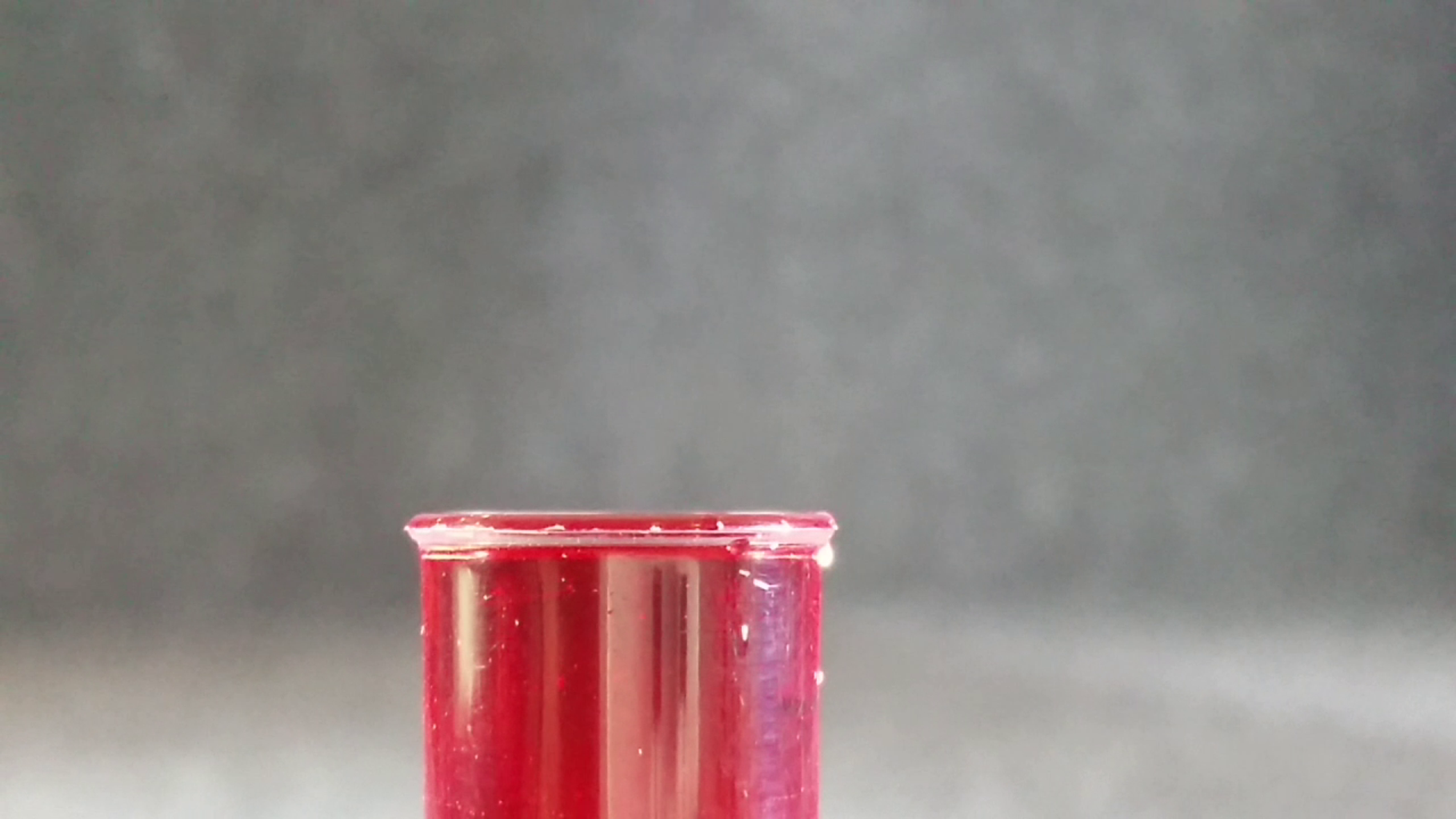
I decided to speak to you in this "instructable" of the surface tension also called water tension.
Click to subscribe to my YouTube channel ► bit.ly/rudyexplor (thanks)
What is the interest to know : the superficial tension?
- To know the cleanliness of the material.
- In order to know if it is bondable and / or Printable
- To know if has been treated
I advise you to go see the video.
For this first experiment, I use a test tube that I fill to the brim.
Do you think that one can put higher than the height of its walls ?
When water is poured above the boundary of a container,
the liquid assumes a spherical shape,
so that its walls occupy the least possible of surface. It not overflow.
All my videos are translat with the subtitles. If you want to make changes to the subtitles of the videos. (I do not necessarily speak very good English)
Go to the video that you want to contribute closed captions to. In the player, select the gear icon. From the drop-down menu, select Subtitles / CC> Add subtitles or CC. You can then modify them
Step 1: The Water Tension on a Coin
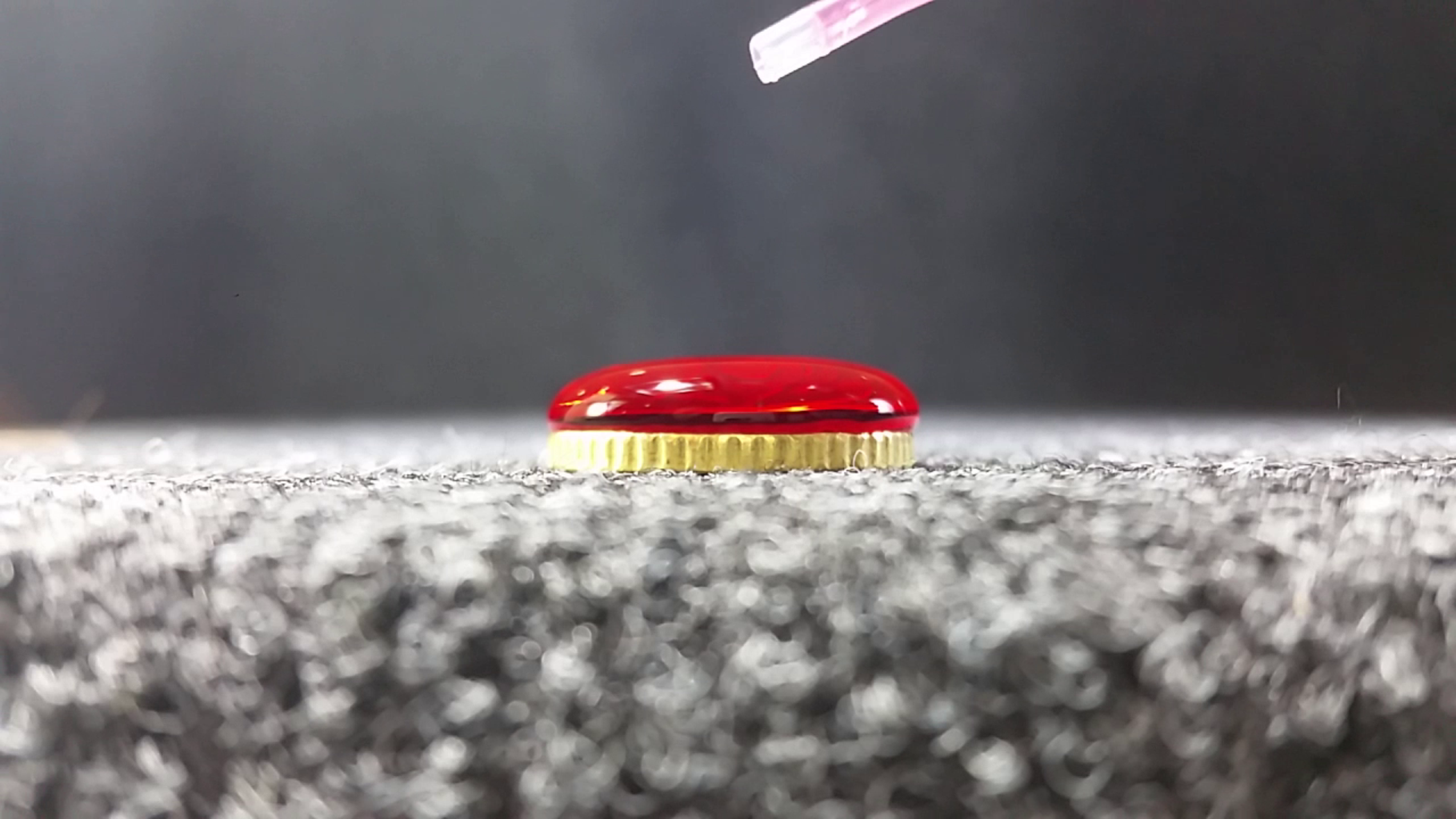
For this second experiment I use a coin.
The surface tension keeps water molecules tied together, forming a kind of membrane or skin.
This half sphere is called meniscus.
Its height is even higher than the height of the coin.
And when the tension is too strong the membrane is broken and iteh water overflows.
Step 2: The Water Tension With Pepper and With Milk
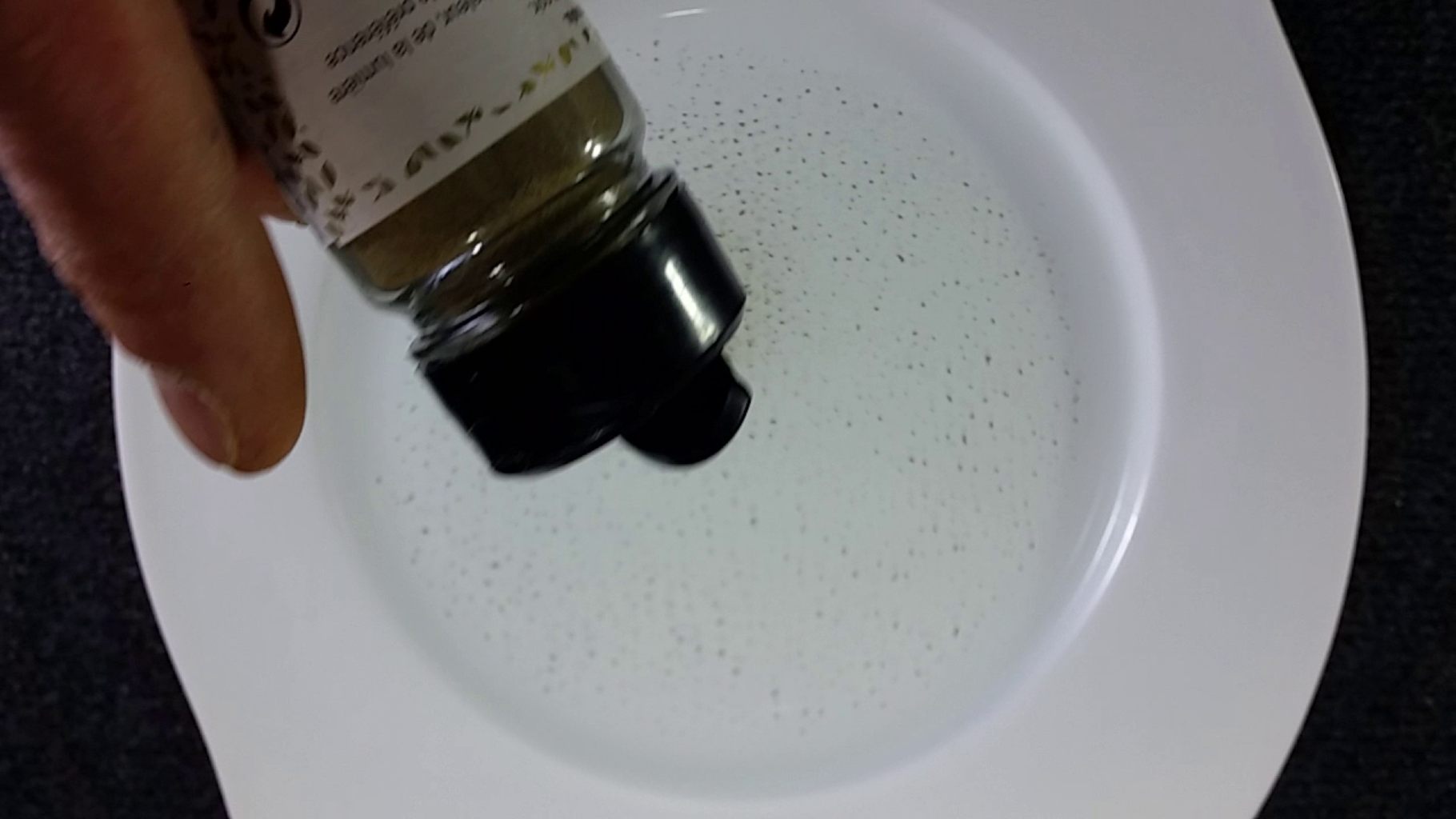
In this third experiment, a plate with water is sprinkled with pepper, the pepper floats thanks to the surface tension and if a drop of dishwashing liquid or soap is added, this breaks up part of the surface tension. And the pepper and push back on the edges of the plate.
In this fourth experiment, on plate with milk , drops dyes are add in the center. It is the same principle as pepper but much more artistic. When you add a drop of dishwashing liquid this is what happens.
Step 3: Water Drop on Ceiling
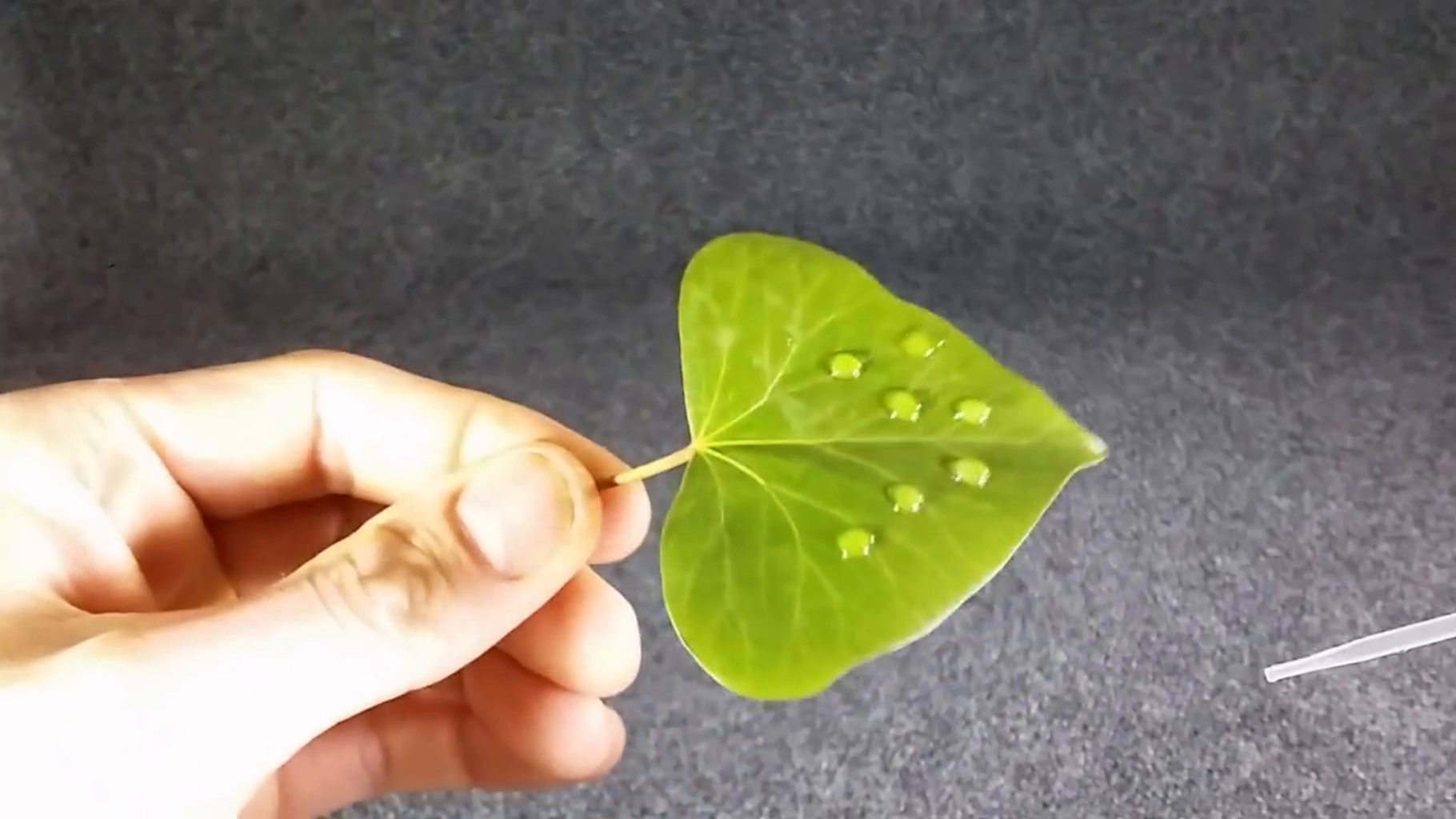
In this fifth experiment. When a drop of water is added to a sheet, it takes on a spherical shape.
Do you think a drop of water can fit on the ceiling? The answer is yes. This tension is so strong that it maintains the drop of water hanging on the turned-up sheet. It is therefore possible to have a drop of water on the ceiling.
Step 4: Tension of Water in a Glass
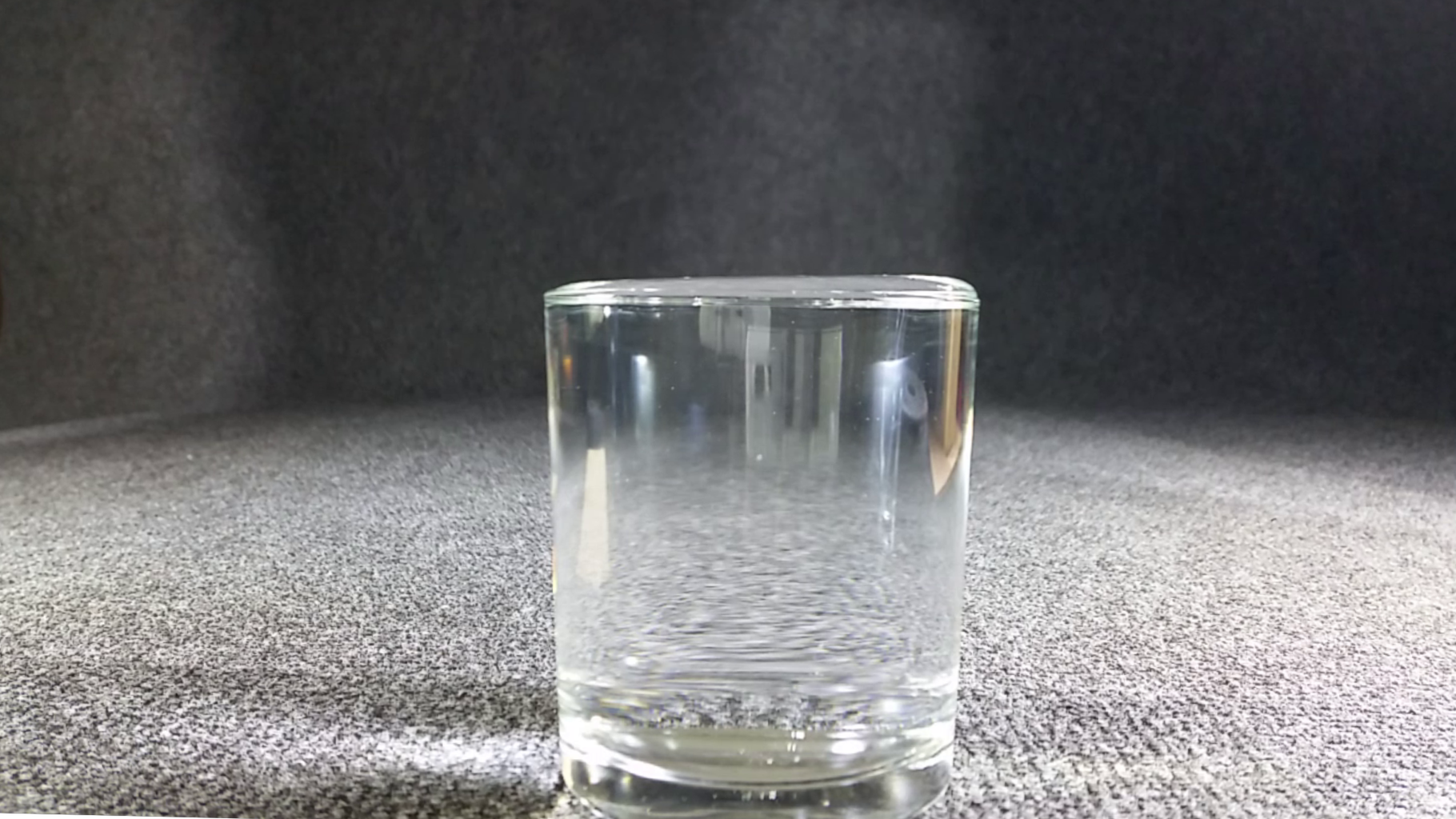
From the sixth to the thirteenth experience
Here I use a glass filled with water. Do you think we can float metal? The answer is yes. The trombone floats. This surface tension can be overcome by exerting a simple pressure on the object and it flows or as with pepper add a drop of dishwashing liquid that is surfactant and tension is broken. A cutter blade can be floated, Float a safety pin, Or needle, brief you will have understood a whole lot of object. With a material density greater than 1. Impressive not? This shows you the strength of this superficial tension.
Step 5: Make a Compass With Water Tension
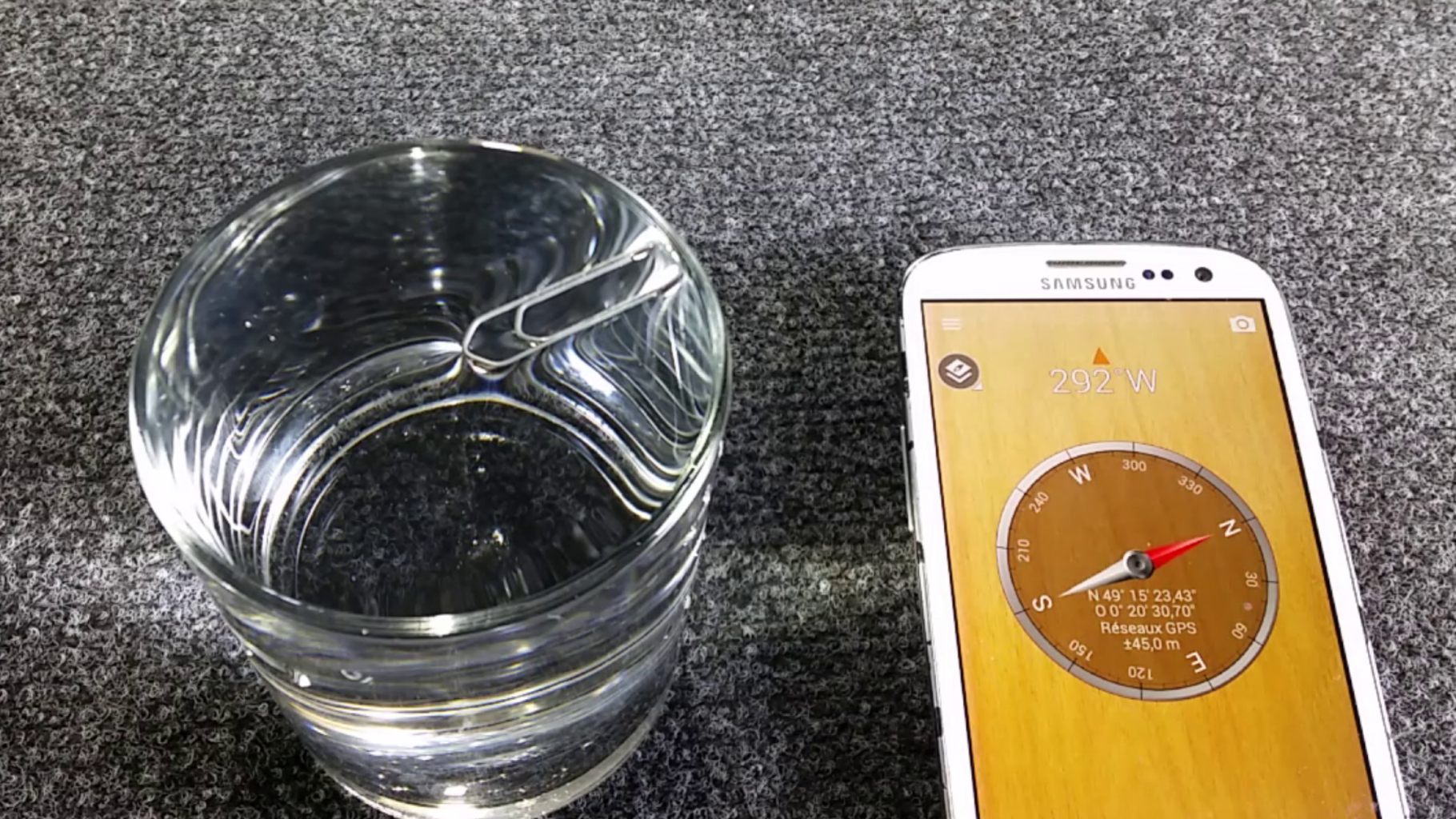
Experience fourteen to sixteen
If you magnet the trombone,
It will indicate in addition to floating the magnetic north.
It's the same as for all metals even the cutter blade.
You learned at school how to make a compass with a cork and a needle Why use the cork,
why not tell us about the surface tension.
Really impressive no ?
If you liked, Please, subscribe to my YouTube channel ► https://goo.gl/PkIcj8 (thanks)
Step 6: Water Tension With a Bottle
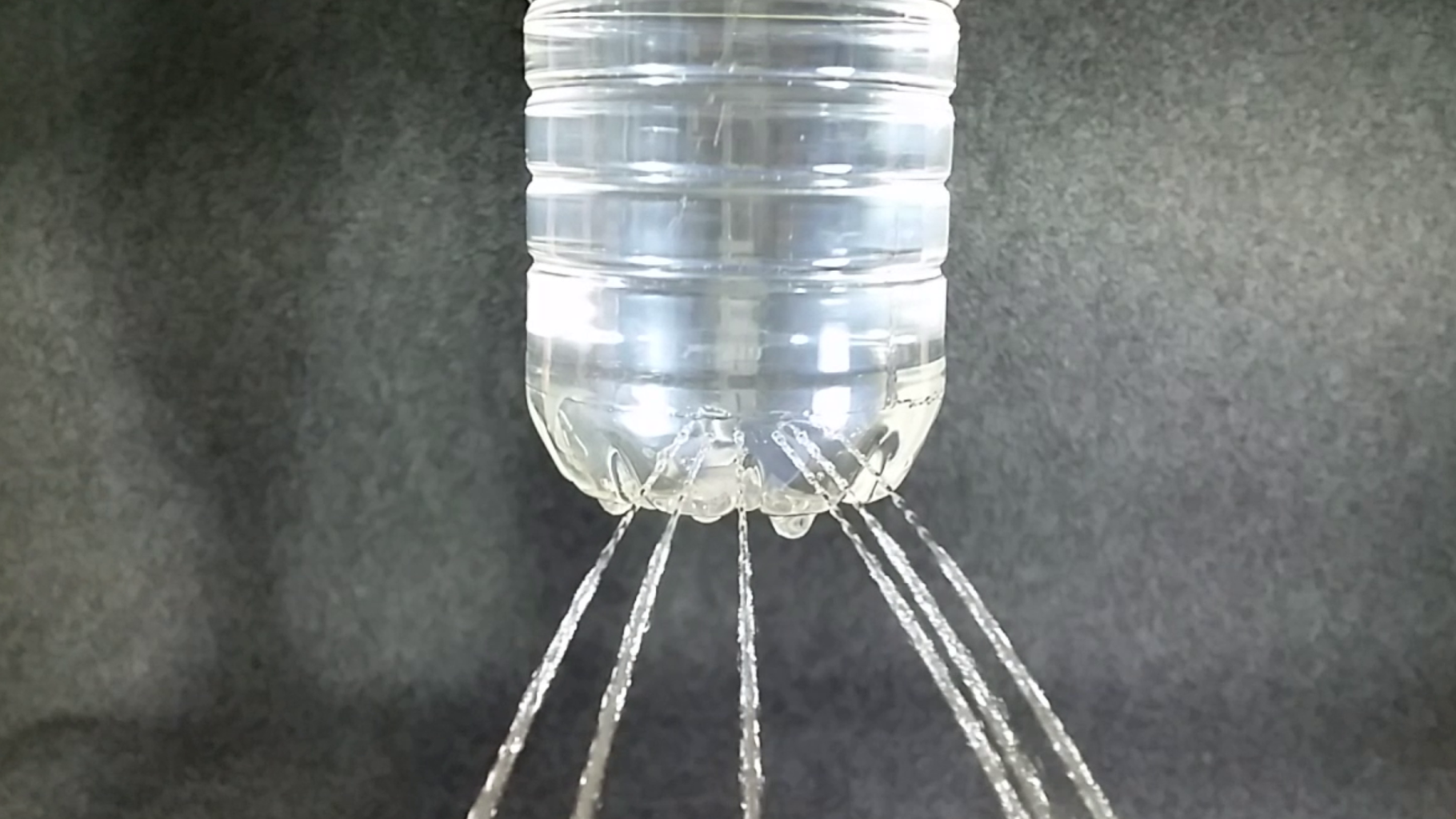
Experience seventeen
If a water bottle is drilled with several very close holes, each hole evacuates the water by a separate jet. If one passes a finger horizontally in front of these holes the superficial tension force gathers the jet in 1 alone.
By ironing the finger vertically the jets are again distinct.
The liquid gathers the jet in 1 alone to minimize its surface
Step 7: Caps Perforated, the Bottle Does Not Sink
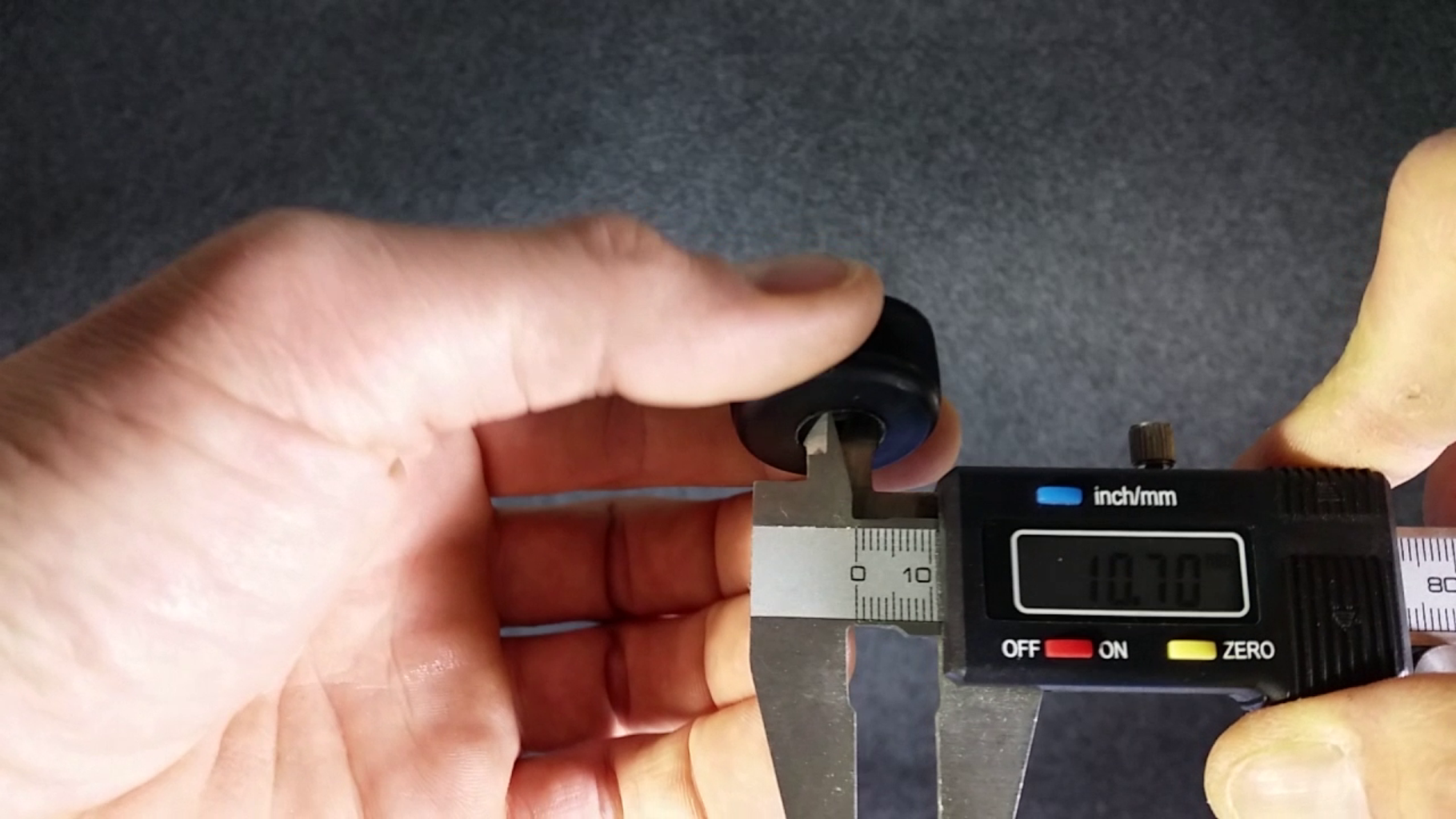
Experience eighteen
I pierced the stopper of a bottle about 12.5 cm. I fill the bottle and close the cap do you think the water will sink?
Thanks to the tension of the water and the pressure of the air, one notices that the water does not flow.
It is the same principle that when you drill a bottle of water with the stopper closed the water does not flow.
Step 8: Strength of the Water Tension With a Rectangle
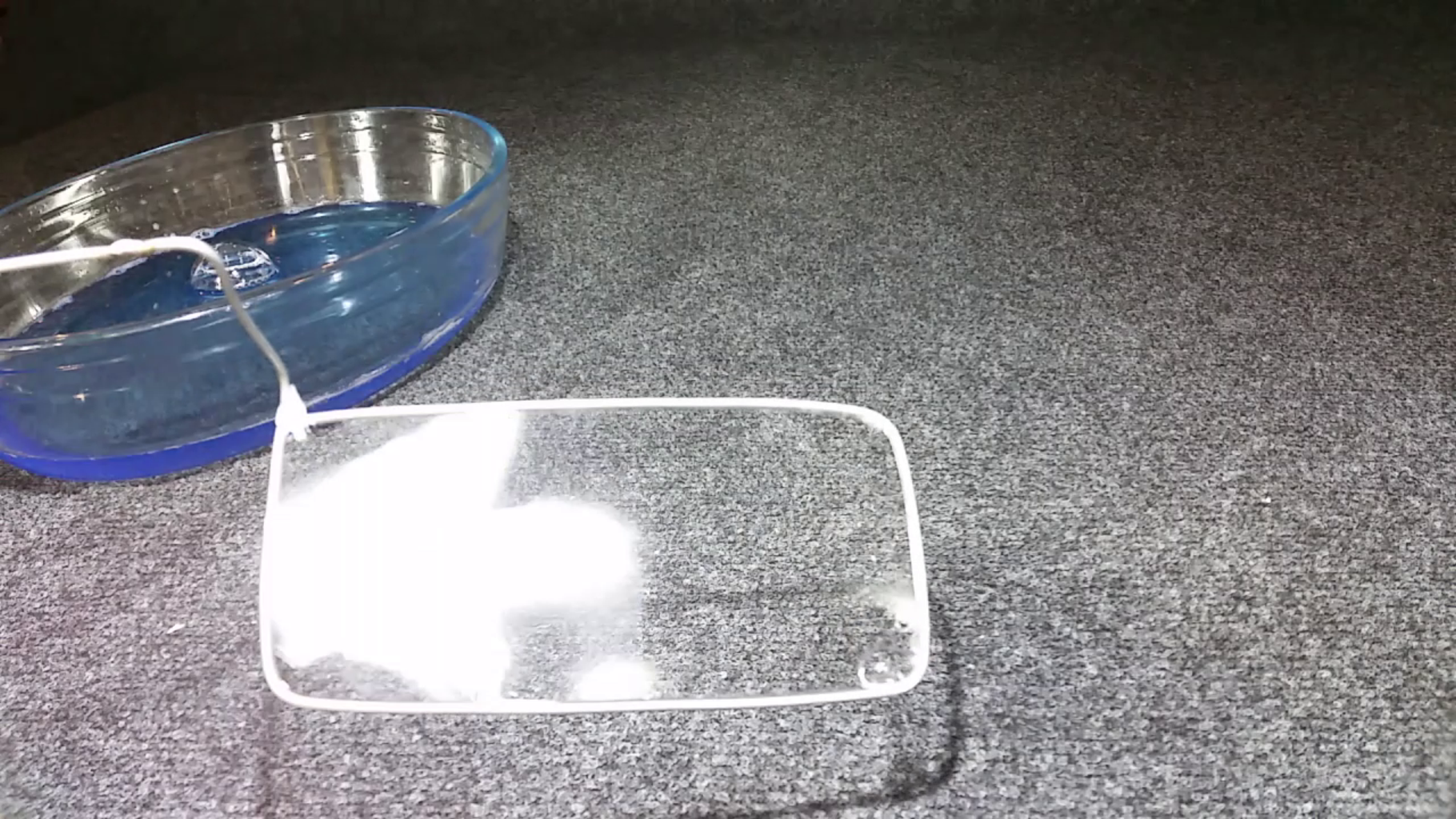
Experience nineteen
If you take a rectangle made with wire and plunge it into soapy water On the rectangle I pose a wire. While there are of two sides of the fil a soapy film the iron wire remains in balance if we broken a part, the attraction force of the remaining part rolls the cylinder to decrease the surface but With the inertia of the rotation the thread falls and the second part disappears
Step 9: Force De La Tension De L'eau Avec Un Cercle
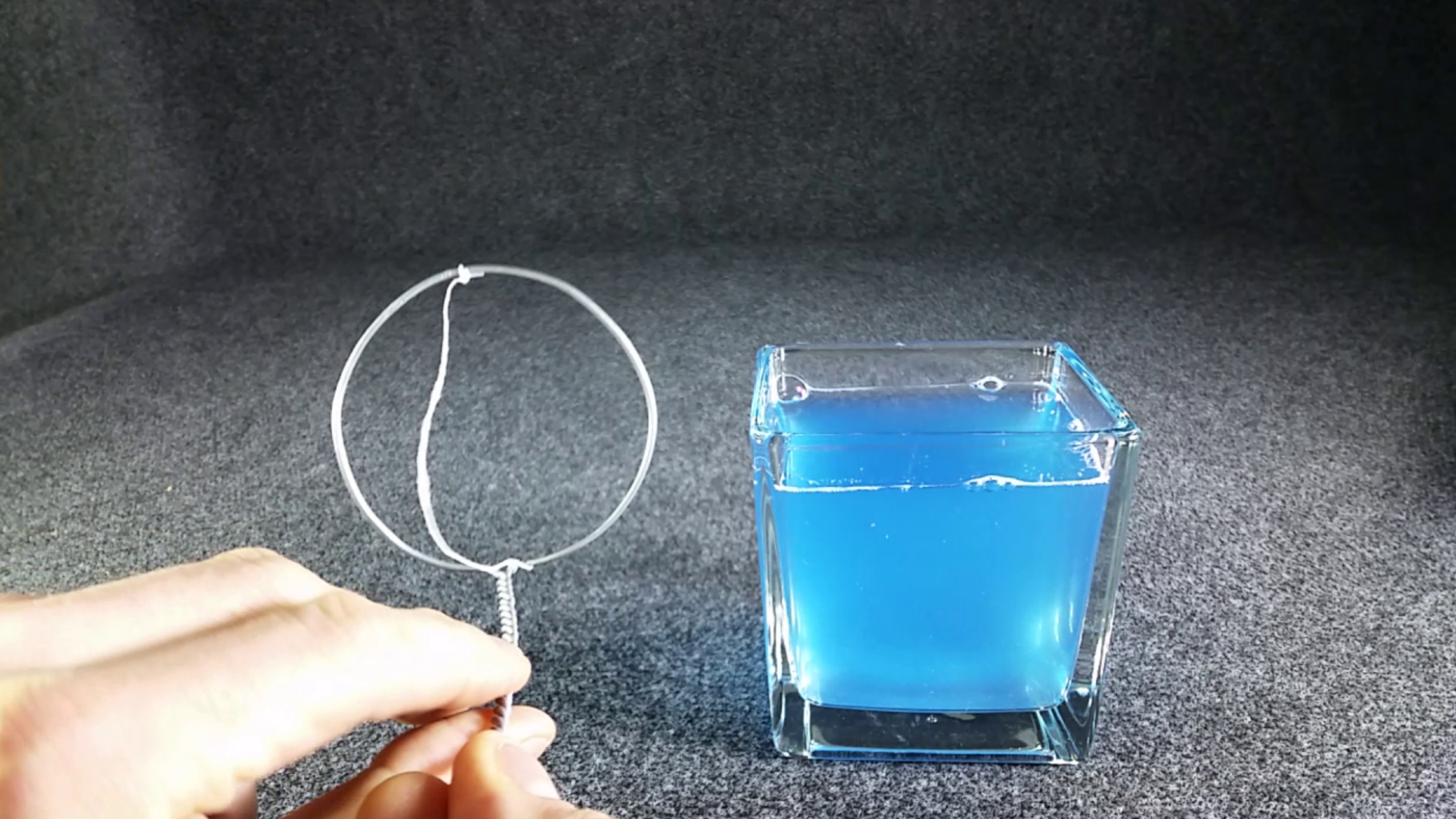
Experience twenty
Taking a ring made of iron wire, a cotton thread is attached to the untensioned medium. This ring is dipped in soapy water. From each side of the wire a film of soap appears.
If one of the soapy surfaces is punctured.
the wire stretches because the remaining part of the soap film pulls the thread towards it to minimize its surface area. If one breaks the second part the wire is no longer subjected to surface tension and it hangs again freely
Step 10: Strength of Water Tension With a Tetrahedron
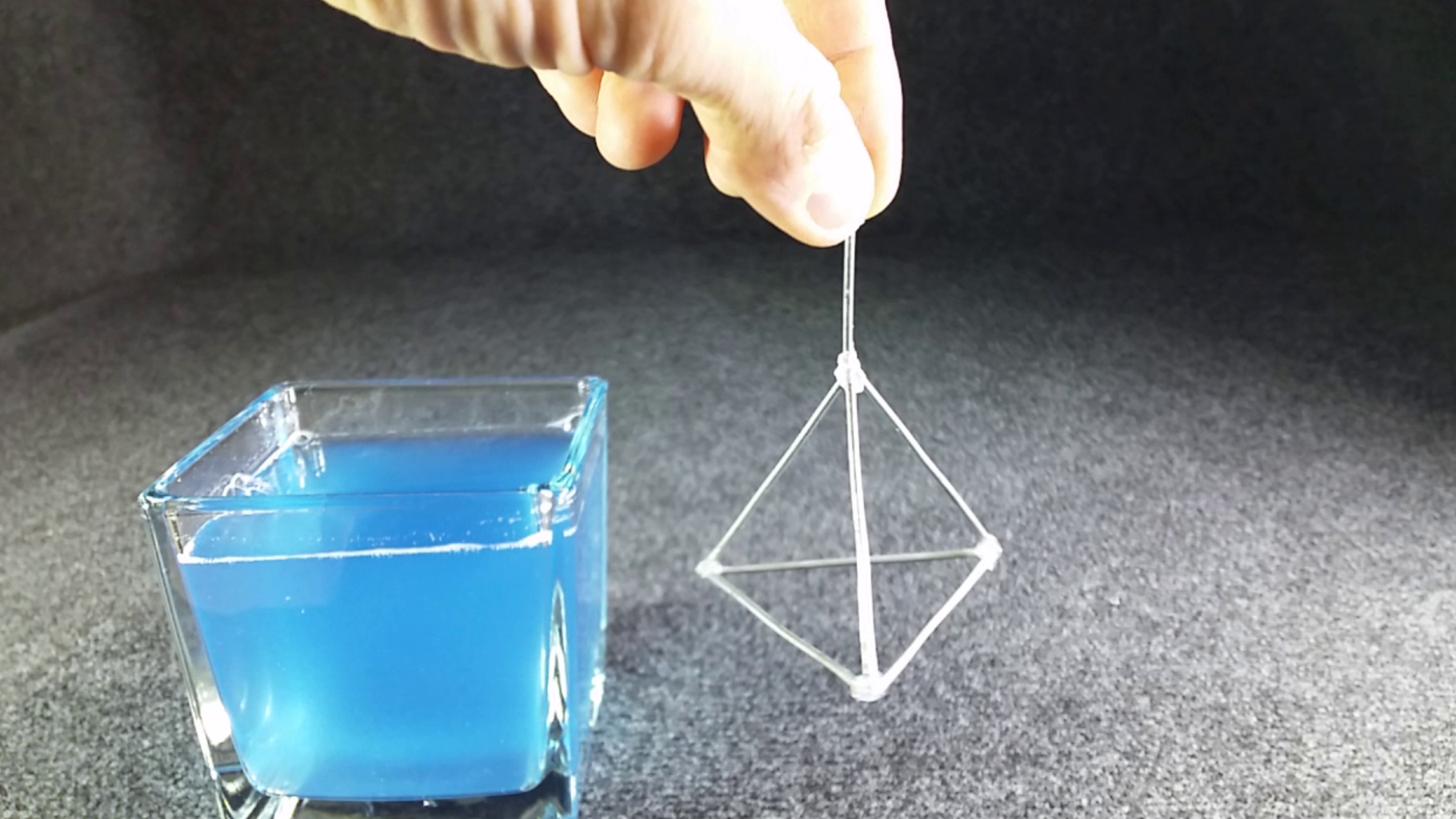
Bonus experience
Always with the iron wire I can create a regular tetrahedron, which you think it will pass ?
There are not 4 sides but 6 sides. Impressive no ! The common point of its faces and a central point. Mathematically it can be shown that the total surface of its 6 sides is less than the total surface of 4 sides of the tetrahedron. The liquid to find the best geometric solution to reduce the surface.
Step 11: Strength of Water Tension With a Cube
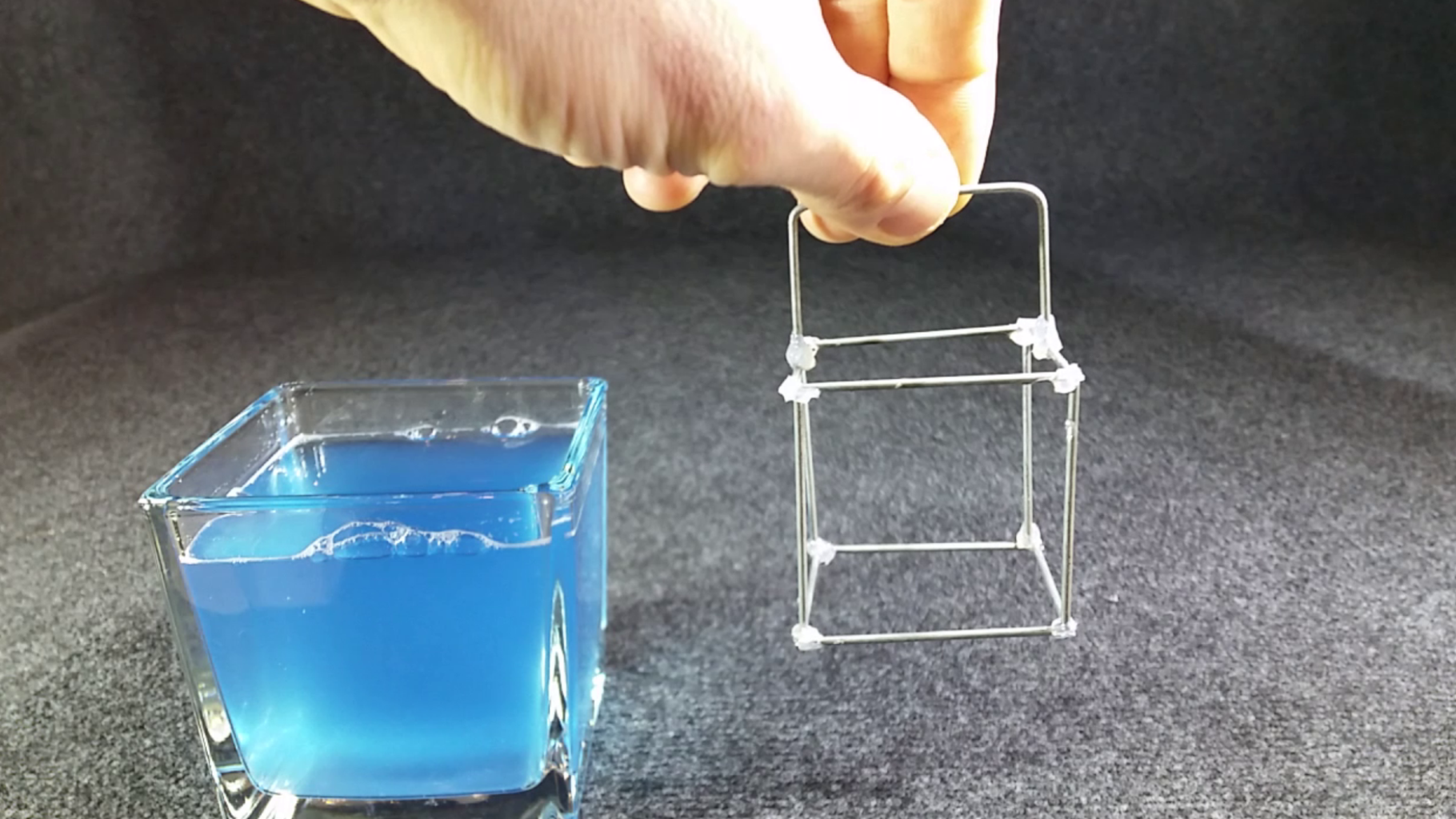
And with a cube what do you think it will happen? One would think that each of the 6 sides would have a soapy film. But again it is not the case there are not 6 sides but 9 sides.
Impressive no !
The common point of its 9 faces and a central face which is a square. Again mathematically it can be shown that the total surface of its 9 sides is smaller than the total surface of the 6 sides of the cube.
The liquid thus finds again the best geometric solution to reduce its surface.
I hope you enjoyed this instructable and this experiment. Leave me a message,it will please me.
If you want to see more,
you can visit my YouTube channel : bit.ly/rudyexplor
Surface Tension - Definition and Experiments
Understand Surface Tension in Physics
- Physics Laws, Concepts, and Principles
- Quantum Physics
- Important Physicists
- Thermodynamics
- Cosmology & Astrophysics
- Weather & Climate
Causes of Surface Tension
Examples of surface tension, anatomy of a soap bubble, pressure inside a soap bubble, pressure in a liquid drop, contact angle, capillarity, quarters in a full glass of water, floating needle, put out candle with a soap bubble, motorized paper fish.
:max_bytes(150000):strip_icc():format(webp)/AZJFaceShot-56a72b155f9b58b7d0e783fa.jpg)
- M.S., Mathematics Education, Indiana University
- B.A., Physics, Wabash College
Surface tension is a phenomenon in which the surface of a liquid, where the liquid is in contact with a gas, acts as a thin elastic sheet. This term is typically used only when the liquid surface is in contact with gas (such as the air). If the surface is between two liquids (such as water and oil), it is called "interface tension."
Various intermolecular forces, such as Van der Waals forces, draw the liquid particles together. Along the surface, the particles are pulled toward the rest of the liquid, as shown in the picture to the right.
Surface tension (denoted with the Greek variable gamma ) is defined as the ratio of the surface force F to the length d along which the force acts:
gamma = F / d
Units of Surface Tension
Surface tension is measured in SI units of N/m (newton per meter), although the more common unit is the cgs unit dyn/cm (dyne per centimeter).
In order to consider the thermodynamics of the situation, it is sometimes useful to consider it in terms of work per unit area. The SI unit, in that case, is the J/m 2 (joules per meter squared). The cgs unit is erg/cm 2 .
These forces bind the surface particles together. Though this binding is weak - it's pretty easy to break the surface of a liquid after all - it does manifest in many ways.
Drops of water. When using a water dropper, the water does not flow in a continuous stream, but rather in a series of drops. The shape of the drops is caused by the surface tension of the water. The only reason the drop of water isn't completely spherical is that the force of gravity pulling down on it. In the absence of gravity, the drop would minimize the surface area in order to minimize tension, which would result in a perfectly spherical shape.
Insects walking on water. Several insects are able to walk on water, such as the water strider. Their legs are formed to distribute their weight, causing the surface of the liquid to become depressed, minimizing the potential energy to create a balance of forces so that the strider can move across the surface of the water without breaking through the surface. This is similar in concept to wearing snowshoes to walk across deep snowdrifts without your feet sinking.
Needle (or paper clip) floating on water. Even though the density of these objects is greater than water, the surface tension along the depression is enough to counteract the force of gravity pulling down on the metal object. Click on the picture to the right, then click "Next," to view a force diagram of this situation or try out the Floating Needle trick for yourself.
When you blow a soap bubble, you are creating a pressurized bubble of air which is contained within a thin, elastic surface of liquid. Most liquids cannot maintain a stable surface tension to create a bubble, which is why soap is generally used in the process ... it stabilizes the surface tension through something called the Marangoni effect.
When the bubble is blown, the surface film tends to contract. This causes the pressure inside the bubble to increase. The size of the bubble stabilizes at a size where the gas inside the bubble won't contract any further, at least without popping the bubble.
In fact, there are two liquid-gas interfaces on a soap bubble - the one on the inside of the bubble and the one on the outside of the bubble. In between the two surfaces is a thin film of liquid.
The spherical shape of a soap bubble is caused by the minimization of the surface area - for a given volume, a sphere is always the form which has the least surface area.
To consider the pressure inside the soap bubble, we consider the radius R of the bubble and also the surface tension, gamma , of the liquid (soap in this case - about 25 dyn/cm).
We begin by assuming no external pressure (which is, of course, not true, but we'll take care of that in a bit). You then consider a cross-section through the center of the bubble.
Along this cross section, ignoring the very slight difference in inner and outer radius, we know the circumference will be 2 pi R . Each inner and outer surface will have a pressure of gamma along the entire length, so the total. The total force from the surface tension (from both the inner and outer film) is, therefore, 2 gamma (2 pi R ).
Inside the bubble, however, we have a pressure p which is acting over the entire cross-section pi R 2 , resulting in a total force of p ( pi R 2 ).
Since the bubble is stable, the sum of these forces must be zero so we get:
2 gamma (2 pi R ) = p ( pi R 2 ) or p = 4 gamma / R
Obviously, this was a simplified analysis where the pressure outside the bubble was 0, but this is easily expanded to obtain the difference between the interior pressure p and the exterior pressure p e :
p - p e = 4 gamma / R
Analyzing a drop of liquid, as opposed to a soap bubble , is simpler. Instead of two surfaces, there is only the exterior surface to consider, so a factor of 2 drops out of the earlier equation (remember where we doubled the surface tension to account for two surfaces?) to yield:
p - p e = 2 gamma / R
Surface tension occurs during a gas-liquid interface, but if that interface comes in contact with a solid surface - such as the walls of a container - the interface usually curves up or down near that surface. Such a concave or convex surface shape is known as a meniscus
The contact angle, theta , is determined as shown in the picture to the right.
The contact angle can be used to determine a relationship between the liquid-solid surface tension and the liquid-gas surface tension, as follows:
gamma ls = - gamma lg cos theta
- gamma ls is the liquid-solid surface tension
- gamma lg is the liquid-gas surface tension
- theta is the contact angle
One thing to consider in this equation is that in cases where the meniscus is convex (i.e. the contact angle is greater than 90 degrees), the cosine component of this equation will be negative which means that the liquid-solid surface tension will be positive.
If, on the other hand, the meniscus is concave (i.e. dips down, so the contact angle is less than 90 degrees), then the cos theta term is positive, in which case the relationship would result in a negative liquid-solid surface tension!
What this means, essentially, is that the liquid is adhering to the walls of the container and is working to maximize the area in contact with solid surface, so as to minimize the overall potential energy.
Another effect related to water in vertical tubes is the property of capillarity, in which the surface of liquid becomes elevated or depressed within the tube in relation to the surrounding liquid. This, too, is related to the contact angle observed.
If you have a liquid in a container, and place a narrow tube (or capillary ) of radius r into the container, the vertical displacement y that will take place within the capillary is given by the following equation:
y = (2 gamma lg cos theta ) / ( dgr )
- y is the vertical displacement (up if positive, down if negative)
- d is the density of the liquid
- g is the acceleration of gravity
- r is the radius of the capillary
NOTE: Once again, if theta is greater than 90 degrees (a convex meniscus), resulting in a negative liquid-solid surface tension, the liquid level will go down compared to the surrounding level, as opposed to rising in relation to it.
Capillarity manifests in many ways in the everyday world. Paper towels absorb through capillarity. When burning a candle, the melted wax rises up the wick due to capillarity. In biology, though blood is pumped throughout the body, it is this process which distributes blood in the smallest blood vessels which are called, appropriately, capillaries .
Needed materials:
- 10 to 12 Quarters
- glass full of water
Slowly, and with a steady hand, bring the quarters one at a time to the center of the glass. Place the narrow edge of the quarter in the water and let go. (This minimizes disruption to the surface, and avoids forming unnecessary waves that can cause overflow.)
As you continue with more quarters, you will be astonished how convex the water becomes on top of the glass without overflowing!
Possible Variant: Perform this experiment with identical glasses, but use different types of coins in each glass. Use the results of how many can go in to determine a ratio of the volumes of different coins.
- fork (variant 1)
- piece of tissue paper (variant 2)
- sewing needle
Place the needle on the fork, gently lowering it into the glass of water. Carefully pull the fork out, and it is possible to leave the needle floating on the surface of the water.
This trick requires a real steady hand and some practice, because you must remove the fork in such a way that portions of the needle do not get wet ... or the needle will sink. You can rub the needle between your fingers beforehand to "oil" it increase your success chances.
Variant 2 Trick
Place the sewing needle on a small piece of tissue paper (large enough to hold the needle). The needle is placed on the tissue paper. The tissue paper will become soaked with water and sink to the bottom of the glass, leaving the needle floating on the surface.
- lit candle ( NOTE: Do not play with matches without parental approval and supervision!)
- detergent or soap-bubble solution
Place your thumb over the small end of the funnel. Carefully bring it toward the candle. Remove your thumb, and the surface tension of the soap bubble will cause it to contract, forcing air out through the funnel. The air forced out by the bubble should be enough to put out the candle.
For a somewhat related experiment, see the Rocket Balloon.
- piece of paper
- vegetable oil or liquid dishwasher detergent
- a large bowl or loaf cake pan full of water
Once you have your Paper Fish pattern cut out, place it on the water container so it floats on the surface. Put a drop of the oil or detergent in the hole in the middle of the fish.
The detergent or oil will cause the surface tension in that hole to drop. This will cause the fish to propel forward, leaving a trail of the oil as it moves across the water, not stopping until the oil has lowered the surface tension of the entire bowl.
The table below demonstrates values of surface tension obtained for different liquids at various temperatures.
Experimental Surface Tension Values
Benzene | 20 | 28.9 |
Carbon tetrachloride | 20 | 26.8 |
Ethanol | 20 | 22.3 |
Glycerin | 20 | 63.1 |
Mercury | 20 | 465.0 |
Olive oil | 20 | 32.0 |
Soap solution | 20 | 25.0 |
Water | 0 | 75.6 |
Water | 20 | 72.8 |
Water | 60 | 66.2 |
Water | 100 | 58.9 |
Oxygen | -193 | 15.7 |
Neon | -247 | 5.15 |
Helium | -269 | 0.12 |
Edited by Anne Marie Helmenstine, Ph.D.
- Understanding Time Dilation Effects in Physics
- 6 Kinds of Simple Machines
- What Is Mass?
- Life of Léon Foucault, Physicist Who Measured the Speed of Light
- Multiverse Definition and Theory
- What Is the Shear Modulus?
- Huygens' Principle of Diffraction
- The Difference Between Terminal Velocity and Free Fall
- What Is Time? A Simple Explanation
- What Is Young's Modulus?
- Understanding What Fluid Dynamics is
- What Is Blackbody Radiation?
- Black Holes and Hawking Radiation
- Quantum Physics Overview
- What Is the Twin Paradox? Real Time Travel
- Heinrich Hertz, Scientist Who Proved Existence of Electromagnetic Waves
Thank you for visiting nature.com. You are using a browser version with limited support for CSS. To obtain the best experience, we recommend you use a more up to date browser (or turn off compatibility mode in Internet Explorer). In the meantime, to ensure continued support, we are displaying the site without styles and JavaScript.
- View all journals
- Explore content
- About the journal
- Publish with us
- Sign up for alerts
- Open access
- Published: 09 September 2024
Hydrogen adsorption on fcc metal surfaces towards the rational design of electrode materials
- Cláudio M. Lousada 1 &
- Atharva M. Kotasthane 1
Scientific Reports volume 14 , Article number: 20972 ( 2024 ) Cite this article
Metrics details
- Electrocatalysis
- Electronic structure
- Heterogeneous catalysis
The successful large-scale implementation of hydrogen as an energy vector requires high performance electrodes and catalysts made of abundant materials. Rational materials design strategies are the most efficient means of reaching this goal. Here we present a study on the adsorption of H-atoms onto fcc transition metal surfaces and propose descriptors for the rational design of electrodes and catalysts by means of correlations between fundamental properties of the materials and among other properties, their experimentally measured performance as hydrogen evolution electrodes (HEE). A large set of quantum mechanical modelling data at the DFT level was produced, covering the adsorption of H-atoms onto the most stable surfaces (100), (110) and (111) of: Ag, Au, Co, Cu, Ir, Ni, Pd, Pt and Rh. For each material and surface, a coverage dependent set of minimum energy structures was produced and chemical potentials for adsorption of H-atoms were obtained. Averaging procedures are here proposed to approach modelling to the experiments. Several correlations between the computed data and experimentally measured quantities are done to validate our methodology: surface plane dependent adsorption energies, chemical potentials and experimentally determined surface energies and work functions. We search for descriptors of catalytic activity by testing correlations between the DFT data obtained from our averaging procedures and experimental data on HEE performance. Our methodology allows us to obtain linear correlations between the adsorption energy of H-atoms and the exchange current density ( i 0 ) in a HEE, avoiding the volcano-like plots. We show that the chemical potential has limitations as a descriptor of i 0 because it reaches an early plateau in terms of i 0 . Simple quantities obtained from database data such as the first stage electronegativity (χ) as devised by Mulliken has a strong linear correlation i 0 . With a quantity we denominate modified second-stage electronegativity (χ 2m ) we can reproduce the typical volcano plot in a correlation with i 0 . A theoretical and conceptual framework is presented. It shows that both χ and χ 2m , that depend on the first ionization potential, second ionization potential and electron affinity of the elements can be used as descriptors in rational design of electrodes or of catalysts for hydrogen systems.
Introduction
Dissociative hydrogen adsorption or H-atom adsorption at metal surfaces is a phenomenon that determines the performance of innumerous systems of high technological and scientific importance. The most obvious connection between this atomic scale process with practical applications is within hydrogen production and usage. This encompasses an array of electrocatalytic systems such as electrodes in diverse electrolysis reactors 1 or in fuel cells 2 , but also photocatalytic systems where there has been a considerable effort to develop H 2 (g) production from the sunlight assisted dissociation of H 2 O at the surface of semiconductors doped with metallic inclusions that function as co-catalysts 3 , 4 . H-atom adsorption is also a key parameter in corrosion and hydrogen absorption and embrittlement by metals 5 , 6 . Additionally, diverse important catalytic processes involve H-atom transfer reactions between molecular species at the surface of high-performance catalysts 7 .
Rational materials design is a strategy for the development of new functional, high-performance materials that is gaining momentum because of the link it can provide between properties and function 8 . This topic has attracted considerable attention because of the developments in modern experimental and computational techniques that allow more detailed studies of materials at the atomic scale 9 . To correlate atomic scale properties with processes to generate descriptors of performance for usage in rational design is of utmost importance for the successful development of better materials 10 . Improving the materials used in high performance applications is an important task that requires access to high-quality data that can be rationalized and correlated to produce descriptors for the rational design of those materials 11 . Especially relevant to this task, is to first develop correlations between properties and function for simpler materials—single component with simpler geometries—before embarking on the study of complex multicomponent-multi-structure systems 12 , 13 .
Despite the relevance of H-atom adsorption at metal surfaces, there is no account that systematically investigates the effects of the substrate in terms of both surface planes and increasing coverage of H-atoms on the adsorption energies (Δ E ads ) and on the driving force for adsorption. Understanding these effects and the origin for the driving force for adsorption and for the formation of chemical bonds is essential to understand and predict performance of materials in real systems. The success of the d band model for this task is unquestionable 14 , 15 . However, this model requires a rather complex analysis to be put in practice 16 . Because of its inherent complexity, its physical significance may not be directly understood by researchers more oriented towards engineering that focus on system design and upscaling. For these tasks, the existence of simpler descriptors of performance that can be used in rational materials design and in the first stages of materials selection, that can link simple properties with performance, and that can be understood in terms of simple quantities that are intrinsic to the materials is important 12 , 17 , 18 .
The available bulk literature data on H-atom adsorption onto different surfaces of common transition metals has been obtained with different methods and by different authors often under different conditions as detailed in the Supplementary Information section to this paper. This has an impact especially in the computational data whose quality relies on the cancelation of errors of differential quantities such as Δ E ads . Computational data obtained with different computational methodologies has different error magnitudes and deviates from the real values in different directions—some methods systematically underestimate while others overestimate—which results in large data dispersions that do not allow accurate analysis, rationalization, and derivation of performance descriptors. Supplementary Information Figure S1 shows how Δ E ads data for adsorption of H-atoms on Cu as a function of surface coverage defined as fraction of monolayer (ML), varies depending on the data source.
In general, for transition metals with fcc structure, the low Miller index surfaces (100), (110), and (111) are the most stable and most frequently occurring in polycrystals 19 . For practical applications these are thus the most relevant surfaces in terms of the performance of the systems where these materials are used for their surface properties. For hydrogen systems that depend on surface reactions, the adsorption energy of the hydrogen atom (Δ E ads H) is among the most significant parameters to consider. This quantity depends on both the geometrical and electronic properties of the adsorbent surfaces. In general, the traditional decoupling of geometric and electronic effects in adsorption can be seen as a necessary oversimplification, because the electronic structure of the surfaces depends on their geometries and vice-versa. Properties that depend on the surface plane such as work function ( W ) among others, are both determined by the surface structure and by bulk properties such as the ionization potential ( IP ), electron affinity ( EA ) and consequently by the electronegativity ( χ ). We have previously shown that for transition metal and lanthanide oxides in solution, very good correlations between experimentally measured catalytic activity and computational data could be obtained against the IP , EA and χ of the cations. These correlations could predict catalytic properties such as energy barriers and strength of adsorption of products and intermediates in processes where free radicals were generated at the surfaces 12 . Based on this principle, we have further derived a descriptor of O-atom adsorption onto clean and doped Al surfaces with different dopants. The descriptor is a modified Mulliken electronegativity that involves the second ionization potential IP 2 of the dopant atom and their first EA 20 . The very good predictive power of this function which is based on textbook data, allowed us to predict trends in adsorption energy of O-atoms using quantities solely intrinsic to the dopant atoms at the surface. The systematic analysis of the electronic structure of the surface-dopant-O-atom complex revealed how the bands and newly formed electronic states sequentially follow a trend that depends on IP and EA and explains why these quantities could be used for devising predictive descriptors of adsorption 20 . The success of this descriptor of adsorption is because it includes quantities dependent on filled or valence states, the IP 2 , and on empty states, the EA . This makes the descriptor able to account for donation and back donation upon formation of chemical bonds at the surface. Because for adsorption of the same adsorbate onto different surfaces, the reference electron energy of the adsorbate is the same, the descriptor will grasp the relative differences between filled and empty states for different surfaces or different materials.
In this work we tested the usage of descriptors for predicting trends in adsorption of H-atoms on a series of transition metals of technological relevance that have fcc structure. We show that the first-stage electronegativity and the modified second-stage electronegativity of the metals are good descriptors of trends in adsorption at (111), (110) and (100) surfaces of fcc metals, and that these descriptors can be used in tasks of rational design or materials selection. The first-stage electronegativity of the metals correlates linearly with experimentally measured exchange current density, avoiding the volcano-like plot. These results also highlight the importance of averaging over different adsorbate coverages and over different surface planes for the modelling data to be closer to the experimental conditions in engineered catalytic reactor systems.
Literature data
Methodologies for literature data collection on hydrogen adsorption are detailed in the Supplementary Information to this paper.
Computational details
Density functional theory (DFT) calculations were performed using the Vienna ab initio simulation package (VASP 5.4.1) 21 with the Perdew–Burke–Ernzerhof (refs 22 , 23 .) (PBE) exchange–correlation functional with ultrasoft pseudopotentials of the projector augmented wave 24 , 25 (PAW) type. The PBE functional has shown good accuracy for describing the adsorption of H-atoms onto metal surfaces 26 , 27 , 28 , and also for surface and bulk properties of metals 29 , 30 . We tested the inclusion of descriptors of van der Waals interactions which are important in molecular adsorption, using the D3 correction 31 as PBE-D3. However, the adsorption energies of H-atoms at the surfaces of these transition metals computed at the PBE level are orders of magnitude larger than the D3 correction to those energies which results in negligible relative differences— < 0.002 eV on average—in the adsorption energies obtained with and without the D3 correction. Because of this and to facilitate direct comparison with the literature, the data here presented has been computed at the PBE level. The energies herein reported are electronic energies at 0 K which allow accurate direct comparisons between preferred binding sites with similar chemical environments 32 , 33 , 34 . The (100), (110) and (111) fcc surfaces were modelled with p (2 × 2) surfaces using the supercell models shown in Fig. 1 .

Supercell models with p (2 × 2) symmetry used to study adsorption of H-atoms onto the fcc transition metals: Ag, Au, Co, Cu, Ir, Ni, Pd, Pt, Rh.
The supercells with periodic boundary conditions have a thickness of five atomic layers for (100) and (110) along the axis perpendicular to the surface planes and four atomic layers for (111), all with vacuum layers of 15 Å. These models have been employed in our previous works for the study of adsorption of H-atoms and are sufficient to reduce spurious interactions between the periodically repeating images 27 , 28 , 35 . Adsorption of H-atoms was investigated at one of the sides of the slabs. During optimization of the geometries, for (100) and (110), the three bottom layers were kept fixed while the remaining top layers as well as the H-atoms were allowed to relax. For (111) the two bottom layers were kept fixed while the remaining top layers and the H-atoms were allowed to relax. For geometry optimizations a plane wave cutoff of 500 eV and a Γ centered k-point mesh of (6 × 6 × 1) in the Monkhorst–Pack sampling scheme were used with a Gaussian smearing of 0.1 eV width. The self-consistent field (SCF) electronic energies were considered as converged when the change was smaller than 1 × 10 −5 eV between cycles and the force acting on each of the atoms smaller than 0.002 eV Å −1 . The study of the adsorption of H-atoms started with a single H-atom per supercell (0.25 ML). At this stage we searched for adsorption site preference. Subsequently the coverage was increased sequentially until one monolayer (1 ML). The definition of ML here used is that recommended by IUPAC 36 .
The adsorption energies of the H-atoms were determined as
where E ( n H- M _slab) is the energy of n H-atoms bound to a specific surface of a metal M ; E H is the energy of an H-atom in vacuum and E M _slab is the energy of the supercell containing the bare surface of M . A more negative value for Δ E ads H implies stronger adsorption. The values of Δ E ads H are normalized per H-atom considering the following correspondence between ML and the number of adsorbed H-atoms per supercell: 0.25 ML = 1H; 0.5 ML = 2H: 0.75 ML = 3H and 1 ML = 4H. Two different average quantities have been devised to express the Δ E ads H. The average over the coverage for each surface plane for each metal is defined as
where Δ Ē ads H (100) , Δ Ē ads H (110) , Δ Ē ads H (111) are the average adsorption energies for the H-atom for each of the surfaces for a given metal; and Δ E ads H (0.25) , Δ E ads H (0.5) , Δ E ads H (0.75) , and Δ E ads H (1) are the corresponding adsorption energies of the H-atom for each surface coverage—0.25 to 1 ML—expressed per H-atom. Because the differences in the surface energies for the three low Miller index surfaces of fcc metals are in general not large 37 , in a polycrystal all these surfaces can be present, even if in different extents, but will thus be responsible for the overall catalytic activity of the material. Hence, the average adsorption energy of the H-atoms for each metal here studied (Δ Ē ads H) was obtained as
The electronic energy dependent chemical potential µ i is defined as a function of ML fraction up to 1 ML as
where Δ E ads is the adsorption energy of i H atoms. This expression does not include the zero-point vibrational energies because in previous works we found that this quantity does not contribute to changes in the trends of adsorption nor absorption for similar geometries 28 , 38 . The expression does not include also corrections for pressure nor temperature. The values of µ were thus determined from plots of Δ E ads H i vs ML for each surface leading to the quantities µ i (100) , µ i (110) and µ i (111) which are the coverage dependent chemical potentials for H-atom adsorption at the corresponding surfaces of each metal. Because each 0.25 increase in ML corresponds to an H-atom, the µ i expressed per ML can be easily expressed per number of H-atoms by computing µ i /4. The average chemical potential µ avg for adsorption of H at the surface of each metal here considered is determined as
Results and discussion
Analysis of literature data.
It is thus expectable that, if for a given material the electronic properties of each surface, for example (100), (110) and (111), change in a consistent way and can be linked to certain trends in adsorption, the intrinsic properties of the materials such as IP , EA , and χ can also be related to the type of surface by a function. Correlations between surface plane dependent work function W , for the (100), (110) and (111) surfaces and bulk IP and EA that are independent of the surface are shown in the Supplementary Information Figure S I2 for a series of transition metals with fcc structure. The correlations between W and IP , EA and χ are not linear as expected because the electronic states of the surfaces that influence W depend on complex quantum mechanical effects that do not correlate linearly with IP , EA and χ . However, the fact that the data of Figure S I2 varies for the different surface planes in a tendentially linear fashion suggests that it is possible to correlate certain aspects of the performance of the surfaces with bulk properties of the materials if we obtain an adequate parameter that “adapts” the bulk properties χ , EA and IP , to the specific surface plane, taking into account a specific surface process, that itself it is surface plane dependent. Such simplification that does not depend on descriptors that need complex computational data would allow a fast track for evaluation of performance and for the design of materials and systems that are aimed at specific surface performance.
Coverage dependent adsorption energies
Adsorbate coverage is an important parameter that determines the adsorption energy 39 , 40 . We have previously demonstrated that even for defective surfaces, adsorbate coverage has an important role both in the formation and in the magnitude of dipoles and in the strength of adsorption 17 , 41 , 42 . Long-range adsorbate–adsorbate effects have been determined to amount to 15 Å in Cu for coverages as low as 1/16 ML and 1/32 ML. For S-atoms, these interactions can affect the magnitude of the adsorption energy by 0.4 eV and the magnitude and sign of local dipole momenta—from + 1.217 D for adsorption at neighboring sites, to − 2.390 D for adsorption at a distance of 18 Å 17 . For applications in high performance catalytic reactors however, higher coverages closer to 1 ML have to be considered. We started by studying the coverage dependence of Δ E ads H from 0.25 to 1 ML. The resulting data is shown in Supplementary Information Figure S I3 and the obtained chemical potentials µ i are summarized in Table 1 .
The dependence of Δ E ads with ML is linear for all metals and surface planes except for Cu(111) and Co(111) most likely due to spin effects as these surfaces are known to undergo adsorbate induced transitions that can lead to non-zero spin momenta and to localized dipoles upon adsorption 17 , 43 , 44 , 45 . The extent of surface reconstruction for the same surface planes at the same coverage of H-atoms is different for different metals as shown in Figure SI4 for Ag and Co. This highlights the importance of studying both different coverages and also allowing the models to relax during geometry optimization to find the real minima. The chemical potentials expressed as a function of surface energy reveal how the process of adsorption resembles that of reconstructing the bulk material: chemical bonds between a surface and an adsorbate which are more similar to the bonds in the bulk metal lead to stronger correlations with the surface energy. The correlations between µ i and the surface energies of the fcc metals are shown in Fig. 2 .

µ i (eV/ML) as a function of the surface energies (eV) for the series of fcc metals here studied. The surface energy of Co(110) is non-existent in the literature and has not been included.
There is a strong dependency of µ i with the surface energy for the sets of surfaces (110) and (111), while for the set (100) the correlation is weak. The 110 surface is the more open of the three which leads to more degrees of freedom for bonding with H-atoms in terms of their disposition ie. top, bridge hollow etc., as highlighted in Figure SI4. The effect of the changes in binding site preference reflects in the correlation between surface coverage and µ i . However, the 110 surface is not the surface for which the binding site preference changes the most with coverage. For the 100 set of surfaces, the changes in adsorption site preference as the coverage of H-atoms changes are the most extreme from the complete set of metals here studied. These effects can be attributed to adsorbate–adsorbate effects that change both magnitude and direction of local dipole momenta at different coverages 17 . These results show the importance of allowing adsorbate geometry relaxation in computations of adsorption dependent such quantities. The consequences of the more extensive changes in binding site preference as a function of coverage (100), are different correlations between the adsorption energy and the descriptors of adsorption that we are devising in this work as it will be shown in the next section.
Descriptors of adsorption energy
Simple descriptors of Δ E ads that predict relative differences in this quantity in catalytic reactors could boost the design of catalysts 46 , 47 , 48 . Predictors of relative Δ E ads H can thus help design better electrodes for electrolysis and fuel cells among other systems where Δ E ads H is of relevance 46 , 49 . In this context, we tested how well our averaging methodology can grasp the non-linearities observed in experiments by correlating experimentally measured Ws of the metals and our average adsorption energies of the H-atom Δ Ē ads H (eV) obtained according to Eq. ( 3 ). The resulting data is shown in Fig. 3 . The data shows that our averaging strategy grasps experimental conditions better than other modelling approaches that focus on single coverages as the linear correlations show.
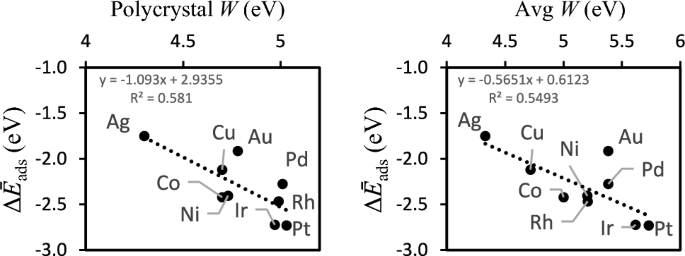
Left panel: experimentally measured work functions W for polycrystals of the metals 50 and their correlation with the average adsorption energies of the H-atom Δ Ē ads H (eV) obtained according to Eq. ( 3 ), over coverage and surface planes. Average (avg) W obtained by averaging the W of the (100), (110) and (111) surfaces retrieved from literature 51 .
Additionally, the correlation between Δ Ē ads H and the two sets of experimental W shows that averaging adsorption energies over coverage and over the surfaces with lower surface energies is a good approach to correlate intrinsic physical properties with adsorption performance. This is because in the polycrystals the three surfaces (100), (110) and (111) can occur because the differences in their energies are small 37 . Averaging over data obtained for each surface is thus a better approximation for polycrystals. The linear correlations between Δ E ads H at each coverage for each type of surface and the W of each surface are weak—0.11 < R 2 < 0.48—for most of the surfaces and coverages as the collection of data in Supplementary Information Figure S I5 shows. The exceptions are the higher coverages 0.75 and 1 ML at the (111) surfaces and the 1 ML coverage at the (110) surfaces. This shows that W cannot account for the shifts in adsorbate binding site preference that occur when the coverage increases, as we have already discussed above. The set of (100) surfaces are those where these effects are more significant because of the larger number of possible adsorbate binding sites. This demonstrates that geometric and electronic effects go hand in hand and methods for prediction of adsorption must account for these. Averaging over both coverage and surface planes, and finding the energy minima for each geometry at each coverage accounts substantially for both effects as Fig. 3 demonstrates.
Descriptors of catalytic activity
The exchange current density, i 0 , in electrochemical reactions is analogous to the rate constant in chemical reactions. For a homologous series of reactions, Brønsted, Evans and Polanyi (BEP) have shown that there is a linear correlation between the transition state energies—that in turn affect the corresponding reaction rate constants—and the adsorption energies in what is now known as the BEP relation 52 , 53 . Several authors have explored this correlation for the successful prediction of catalytic performance of metal surfaces 54 , including ourselves, in our case for surfaces of oxides in solution 12 . Here we explore the correlation between i 0 at the hydrogen evolution electrode (HEE) and the Δ E ads H with an approach fundamentally similar to what was tested previously, but with important differences 55 . The difficulties that the authors of reference 55 encountered are due to the usage of data obtained with different methods for the determination of Δ E ads H which in turn have different errors. Additionally, the study considered only ¼ ML and 1 ML. In the present study we consider the overall average of the Δ E ads H, defined as Δ Ē ads H that in turn is the average of the Δ E ads H obtained for each surface plane also averaging over the different coverages as described in Eq. ( 3 ). This makes the quantity Δ Ē ads H grasp both surface coverage effects on the Δ E ads H and the effects of the surface index in this quantity.
In Fig. 4 , the more reactive metals are at the left of the plot while the less reactive and unreactive are at the right as previously observed for a volcano plot of the activities of these metals 55 . However, the close to linear dependency of i 0 with Δ Ē ads H can be attributed to the fact that as observed for the Ws data of Fig. 3 , the averaging of the adsorption energies in terms of surface planes and in terms of surface coverages grasps the experimental conditions to a better extent that if only selected surface planes or coverages are used. This is because of the highly dynamical nature of the reactions of evolution of hydrogen whose rate of formation depends on the Δ E ads H but the rate of desorption from the surface depends on the surface coverage as well on the relation between Δ E ads H and surface coverage, that we express here as the surface coverage dependent chemical potential, µ i . We have previously succeed in using a similar averaging approach for the prediction of the formation of H 2 (g) at the surface of Cu(110), where averaging over different adsorbate conformation energies and coverages was necessary for accounting for the contributions of adsorbate dispositions that can occur in the real systems because these have similar energies 35 , 41 , 42 , 56 .

Dependency of the exchange current density at the hydrogen evolution electrode log ( i 0 /A cm −2 ) with the adsorption energy of the H-atom Δ Ē ads H (eV) obtained according to Eq. ( 3 ). The linear fit has a slope m = − 2.6, an intercept at origin b = − 10.59 and a goodness of fit of 0.60.
The quadratic correlation between µ avg and i 0 in Fig. 5 shows that a volcano-like trend of quadratic nature may apply to these two quantities. Fitting the data with linear functions leads to very poor correlations for elements whose µ avg are more negative than − 9 eV/ML and a quadratic function leads to better goodness of fit. The data clearly shows two groups of materials in terms of correlation between µ avg and i 0 . It shows also that the driving force for adsorption reaches a plateau in terms of its effect in the electrode performance.

Average chemical potential for H-atom adsorption ( µ avg ) and correlation with the exchange current density at the hydrogen evolution electrode ( i 0 ). µ avg were determined from Eq. ( 5 ). Experimental values of i 0 retrieved from 55 and references therein. Less active catalysts (circles), more reactive catalysts (diamonds).
We previously used primary quantities for the prediction of oxygen adsorption and dissociation on pure and doped Al surfaces 20 and in the description of catalytic activity of oxides in solution 12 , correlating computational with experimental data. The first of those quantities that we will test here is the first-stage electronegativity (χ) as defined by Mulliken. The second quantity is what we called “modified second-stage electronegativity” where instead of the quantities used for the second-stage electronegativity in the definition by Mulliken, we employed the second ionization potential IP 2 of the metal and the one electron affinity EA. The details are given elsewhere 12 , 20 . Both expressions are
where χ 2m is the modified second-stage electronegativity, IP is the first ionization potential of the metal, IP 2 is the second ionization potential of the metal—surface independent quantities–and EA is the electron affinity of the metal—a surface independent quantity as well. IP, IP 2 and EA are quantities—for the elements—retrieved from common databases. The details of the choice of IP 2 instead of IP (first ionization energies) for devising χ 2m are given in our original work. In general, IP 2 together with EA accounts for the different extents of donation-backdonation that occurs when the H-atom binds to the surface 57 , 58 , 59 , 60 , 61 , and the relaxation of the remaining orbitals in the d-band of the metal as described in the Newns-Anderson model. For a given adsorbate, depending on the metal, the balance between donation-backdonation of electron density will change. Our descriptor (χ 2m ) grasps the correct phenomenology of these processes for dopants on Al surfaces and their effect on oxygen adsorption 20 . For the set of fcc metals here study, the plots of the exchange current density ( i 0 ) in a hydrogen evolution electrode versus χ and χ 2m are shown in Fig. 6 .
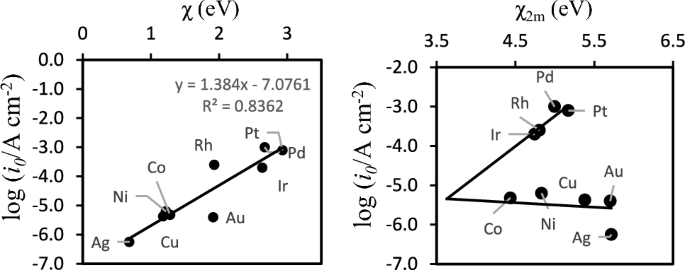
First-stage electronegativity χ (eV) and modified second-stage electronegativity χ 2m (eV) determined according to Eqs. ( 6 ) and ( 7 ) and their correlations with the exchange current density i 0 . χ and χ 2m are obtained from the well-known quantities IP and EA. Experimental values of i 0 retrieved from 55 and references therein.
The data of Fig. 6 shows an excellent correlation between the first-stage electronegativity of the metals (χ) and i 0 . This shows that χ can in fact be used as a linear descriptor in the search for catalysts for HEE. Further support to this observation is also given by χ 2m in Fig. 6 . The “sideways volcano plot” resultant from correlating χ 2m with i 0 provides considerable insight into the phenomenological description of bonding: donation–backdonation, between the H-atom and the surfaces as it will be discussed in the next section.
Theoretical and conceptual framework of the descriptors of catalytic activity
In the Newns–Andersson model the energetic positioning of the d-band center with respect to the orbitals of the adsorbate explains certain trends in catalytic activity. The direct correlation between the model and a surface process is with regard to adsorption energies, that in turn relate with frequency of events or with rates by the BEP relation leading to an indirect correlation between the d-band derived quantities and the frequency or rates of events. Bonding between an adsorbate to a series of surfaces can thus be rationalized in terms of how high or low the d-band center and/or edge for the different materials lay with respect to one another. However, this model does not account directly for the role of electrons of the metal that lay in other orbitals such as p and s and nor accounts for effects of Pauli exclusion for example. The relationship between the energies of the d-band and the p and s bands of the metal is considered only indirectly because certain shifts in the d-band can lead to shifts in the p and s energies, but this is not a universal rule, it is in fact far from it 16 , 62 , 63 . The details of this model and how the chemical bonding between different surfaces and adsorbates come into play have been extensively described in reference texts 62 , 64 , 65 .
In our model here presented we use quantities that despite their simplicity contain the necessary information for the description of donation and backdonation from and to adsorbates. Many of the data here computed is correlated with polycrystalline and “poly-Miller-index” surfaces. The averaging over coverage and surface plane that we have employed is necessary to obtain such correlations which in turn show that there are primary electronic properties of the materials which are surface plane independent and still determinant for the description of the activity of the metal as a HEE. However, such primary quantities require an algebraic workout to obtain a descriptor that contains the correct assessment of electron donation and backdonation to and from the adsorbate, and that weighs these two processes of donation and backdonation. The typical quantities devised for example in conceptual DFT 66 , are related with where the electron orbitals are located energetically. As we previously employed for predicting reactivity of transition metal and actinide oxides 12 , this allows a description of hardness, softness and similar properties that depend on the work necessary to attach and detach electrons. However, the driving forces for the loss or gain of electrons are equally well described by the IP, IP 2 and EA provided the correct algebraic expression is used.
The plots correlating the primary and secondary quantities used to determine the first-stage and second-stage electronegativities, χ and χ 2m respectively, and the average adsorption energies of H-atoms, Δ Ē ads H are given Fig. 7 .
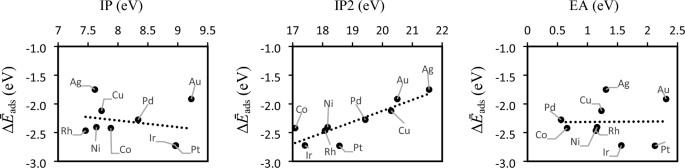
First ionization potential IP, second ionization potential IP 2 and electron affinity EA and their correlation with the average adsorption energies Δ Ē ads H of the H-atom to the corresponding metals obtained according to Eq. 3 . The values of IP, IP 2 and EA used here are retrieved from common databases for the elements and are surface independent properties.
The first ionization potential IP accounts for the energy of the highest occupied electronic states of the metal, with no distinction between orbitals, d or p. IP accounts for the energy—or work necessary if we wish to express so—for the electron transfer from the metal to occur. The electron affinity EA accounts for the ability of the metal to accommodate electrons. This quantity can be positive or negative but for metals it is mostly positive, meaning that energy is released when the metal atom accepts an incoming electron. The second ionization potential, IP 2 accounts for the energy necessary to remove a second electron from the metal. This quantity includes thus the relaxation of the metal orbitals after the first electron has been removed which in turn account for electronic relaxation effects in the donation–backdonation to and from the adsorbate. IP and IP 2 are largely related but include different physical descriptors of the electronic structure and this is demonstrated by the different degrees of correlation and signs of the slopes of their plots versus Δ Ē ads H in Fig. 7 . The first-stage electronegativity χ that contains IP and EA will thus account for the balance between the energy gained and lost in the donation-backdonation to and from the H-atom to the surfaces. The second-stage electronegativity χ 2m will account for relaxation effects of the orbitals of the metal in the bonding with the adsorbate. This is because it accounts for the ease of removal of a second electron from the metal, which in turn is a quantity that contains information about the ease of relaxation of the highest energy electronic bands of the metal. Interestingly, the quantities IP and EA, despite leading to poor correlations with the adsorption energy of the H-atoms as shown in Fig. 7 , are included both in the linear correlation between χ and i 0 and the volcano like correlation between χ 2m and i 0 shown in Fig. 6 that result in strong correlations. This shows that χ and χ 2m contain the essential information of electronic structure features of the metals that determine the dynamical factors that result in different i 0 in the HEE. χ and χ 2m have thus the potential to be used as descriptors in the search for HEE materials and other electrode or catalytic materials where adsorption of H-atoms is a key process. The simplicity of these functions means that they can be easily implemented in machine learning algorithms for catalyst screening and design.
Conclusions
The search for a large set of literature data on H-atom adsorption on fcc metals shows that data computed by different authors with different methods have uncertainties that do not allow the creation of statistically significant sets of data for usage in analysis of properties with good accuracy. Thus we present here a large set of DFT data on H-atom adsorption on the surfaces of fcc metals that is both coverage and surface plane dependent. Despite our set of data being obtained for discrete surface planes and coverages, our averaging procedure leads to data that can be correlated with experimental properties obtained for polycrystals. This shows that our averaging procedure can approximate the DFT data to the diversity of coverages and surfaces that experiments deal with. The correlations between surface energies, work functions and adsorption related properties if obtained for single surface planes and coverages without averaging are poor. This is because changing coverage implies changes in adsorbate biding site preferences for certain planes also accompanied by different extents of surface reconstruction. Our averaging methodology grasps these effects and allows the correlation between H-atom adsorption and experimental data obtained for polycrystals as shown by the linear correlations that we obtained between DFT data and exchange current density ( i 0 ) in a hydrogen evolution electrode.
We also propose descriptors of electrode activity using the first-stage (χ) and second-stage electronegativity (χ 2m ). Because these quantities depend on electron energies of the metals, they include the essential information that determines donation and backdonation to and from adsorbates and even effects of electronic relaxation. The result is that can χ be linearly correlated with i 0 and χ 2m can be correlated with i 0 via a function that resembles the typical volcano plot. Because of these strong correlations, especially between i 0 and χ with is strongly linear, both χ and χ 2m can be used as robust descriptors of catalytic activity in search and in the design of new materials for usage as hydrogen evolution electrodes. The direct dependence of χ and χ 2m on electron energy levels simplifies considerably the number of degrees of freedom to consider in the design of catalysts.
Data availability
The data generated is available from the corresponding author upon reasonable request.
Wang, S., Lu, A. & Zhong, C. J. Hydrogen production from water electrolysis: Role of catalysts. Nano Converg. 8 (1), 4. https://doi.org/10.1186/s40580-021-00254-x (2021).
Article CAS PubMed PubMed Central Google Scholar
Zhong, C. J. et al. Nanostructured catalysts in fuel cells. Nanotechnology 21 (6), 062001. https://doi.org/10.1088/0957-4484/21/6/062001 (2010).
Article ADS CAS PubMed Google Scholar
Cao, H., Tang, X., Tang, H., Yuan, Y. & Wu, J. Photoinduced intermolecular hydrogen atom transfer reactions in organic synthesis. Chem. Catal. 1 (3), 523–598. https://doi.org/10.1016/j.checat.2021.04.008 (2021).
Article CAS Google Scholar
Engge, Y., Maulana, F. & Nurhuda, M. Dissociation of water into hydrogen and oxygen through a combination of electrolysis and photocatalyst. IOP Conf. Ser. Earth Environ. Sci. 743 (1), 012054. https://doi.org/10.1088/1755-1315/743/1/012054 (2021).
Article Google Scholar
Okonkwo, P. C. et al. A focused review of the hydrogen storage tank embrittlement mechanism process. Int. J. Hydrog. Energy https://doi.org/10.1016/j.ijhydene.2022.12.252 (2023).
Dong, L. et al. Application of atomic simulation for studying hydrogen embrittlement phenomena and mechanism in iron-based alloys. Int. J. Hydrog. Energy. 47 (46), 20288–20309. https://doi.org/10.1016/j.ijhydene.2022.04.119 (2022).
Article ADS CAS Google Scholar
Jia, J. et al. Solar water splitting by photovoltaic-electrolysis with a solar-to-hydrogen efficiency over 30. Nat. Commun. 7 (1), 13237. https://doi.org/10.1038/ncomms13237 (2016).
Article ADS CAS PubMed PubMed Central Google Scholar
Gao, D., Arán-Ais, R. M., Jeon, H. S. & Roldan Cuenya, B. Rational catalyst and electrolyte design for CO 2 electroreduction towards multicarbon products. Nature Catal. 2 (3), 198–210. https://doi.org/10.1038/s41929-019-0235-5 (2019).
Belviso, F. et al. Viewpoint: Atomic-scale design protocols toward energy, electronic, catalysis, sensing applications. Inorg. Chem. 58 (22), 14939–14980. https://doi.org/10.1021/acs.inorgchem.9b01785 (2019).
Article CAS PubMed Google Scholar
Cheng, N., Zhang, L., Doyle-Davis, K. & Sun, X. Single-atom catalysts: From design to application. Electrochem. Energy Rev. 2 (4), 539–573. https://doi.org/10.1007/s41918-019-00050-6 (2019).
Wang, W., Liu, J., Yu, M., Zhao, C. & Wang, L. Acceleration of material R&D process through rational design. Comput. Mater. Sci.. 160 , 397–402. https://doi.org/10.1016/j.commatsci.2019.01.009 (2019).
Lousada, C. M., Brinck, T. & Jonsson, M. Application of reactivity descriptors to the catalytic decomposition of hydrogen peroxide at oxide surfaces. Comput. Theor. Chem. 1070 , 108–116. https://doi.org/10.1016/j.comptc.2015.08.001 (2015).
Suen, N. T. et al. Electrocatalysis for the oxygen evolution reaction: Recent development and future perspectives. Chem. Soc. Rev. 46 (2), 337–365. https://doi.org/10.1039/c6cs00328a (2017).
Hammer, B. & Norskov, J. K. Why gold is the noblest of all the metals. Nature 376 (6537), 238–240. https://doi.org/10.1038/376238a0 (1995).
Pettersson, L. G. M. & Nilsson, A. A molecular perspective on the d-band model: Synergy between experiment and theory. Topics Catal. 57 (1), 2–13. https://doi.org/10.1007/s11244-013-0157-4 (2014).
Xin, H. & Linic, S. Communications: Exceptions to the d-band model of chemisorption on metal surfaces: The dominant role of repulsion between adsorbate states and metal d-states. J. Chem. Phys. 132 , 22. https://doi.org/10.1063/1.3437609 (2010).
Lousada, C. M., Johansson, A. J. & Korzhavyi, A. Adsorption of hydrogen sulfide, hydrosulfide and sulfide at Cu(110)—polarizability and cooperativity effects. First stages of formation of a sulfide layer. ChemPhysChem 19 (17), 2159–2168. https://doi.org/10.1002/cphc.201800246 (2018).
Montemore, M. M. & Medlin, J. W. Predicting and comparing C–M and O–M bond strengths for adsorption on transition metal surfaces. J. Phys. Chem. C 118 (5), 2666–2672. https://doi.org/10.1021/jp5001418 (2014).
Tran, R. et al. Surface energies of elemental crystals. Sci. Data 3 (1), 160080. https://doi.org/10.1038/sdata.2016.80 (2016).
Article MathSciNet CAS PubMed PubMed Central Google Scholar
Lousada, C. M. & Korzhavyi, P. A. Oxygen adsorption onto pure and doped Al surfaces—The role of surface dopants. Phys. Chem. Chem. Phys. 17 (3), 1667–1679. https://doi.org/10.1039/C4CP04277H (2015).
Kresse, G. & Furthmüller, J. Efficient iterative schemes for ab initio total-energy calculations using a plane-wave basis set. Phys. Rev. B 54 (16), 11169–11186 (1996).
Perdew, J. P., Burke, K. & Ernzerhof, M. Generalized gradient approximation made simple. Phys. Rev. Lett. 77 (18), 3865–3868 (1996).
Perdew, J. P., Burke, K. & Ernzerhof, M. Generalized gradient approximation made simple. Phys. Rev. Lett. 78 (7), 1396–1396 (1997).
Blöchl, P. E. Projector augmented-wave method. Phys. Rev. Lett. 50 (24), 17953–17979 (1994).
Google Scholar
Kresse, G. & Joubert, D. From ultrasoft pseudopotentials to the projector augmented-wave method. Phys. Rev. B 59 (3), 1758–1775 (1999).
Hanh, T. T. T., Takimoto, Y. & Sugino, O. First-principles thermodynamic description of hydrogen electroadsorption on the Pt(111) surface. Surf. Sci. 625 , 104–111. https://doi.org/10.1016/j.susc.2014.03.006 (2014).
Zhao, M. & Anderson, A. B. Theory of hydrogen deposition and evolution on Cu(111) electrodes. J. Electrochem. Soc. 164 (9), H691–H695. https://doi.org/10.1149/2.1621709jes (2017).
Lousada, C. M. & Korzhavyi, P. A. Hydrogen sorption capacity of crystal lattice defects and low Miller index surfaces of copper. J. Mater. Sci.. 55 (15), 6623–6636. https://doi.org/10.1007/s10853-020-04459-z (2020).
Kurth, S., Perdew, J. P. & Blaha, P. Molecular and solid-state tests of density functional approximations: LSD, GGAs, meta-GGAs. Int. J. Quantum Chem. 75 (4–5), 889–909 (1999).
Haas, P., Tran, F. & Blaha, P. Calculation of the lattice constant of solids with semilocal functionals. Phys. Rev. B 79 (8), 085104. https://doi.org/10.1103/PhysRevB.79.085104 (2009).
Grimme, S., Antony, J., Ehrlich, S. & Krieg, H. A consistent and accurate ab initio parametrization of density functional dispersion correction (DFT-D) for the 94 elements H–Pu. J. Chem. Phys. 132 (15), 154104. https://doi.org/10.1063/1.3382344 (2010).
You, Y.-W. et al. Dissolving, trapping and detrapping mechanisms of hydrogen in bcc and fcc transition metals. AIP Adv. 3 (1), 012118. https://doi.org/10.1063/1.4789547 (2013).
Lousada, C. M., Sophonrat, N. & Yang, W. Mechanisms of formation of H, HO, water and of water desorption in the early stages of cellulose pyrolysis. J. Phys. Chem. C 122 (23), 12168–12176. https://doi.org/10.1021/acs.jpcc.8b02173 (2018).
Heinola, K., Ahlgren, T., Nordlund, K. & Keinonen, J. Hydrogen interaction with point defects in tungsten. Phys. Rev. B 82 (9), 094102. https://doi.org/10.1103/PhysRevB.82.094102 (2010).
Lousada, C. M., Johansson, A. J. & Korzhavyi, P. A. Thermodynamics of H2O splitting and H2 formation at the Cu(110)–water interface. J. Phys. Chem. C 119 (25), 14102–14113. https://doi.org/10.1021/acs.jpcc.5b01154 (2015).
McNaught, A. D. & Wilkinson, A. Compendium of chemical terminology. In The Gold Book 2nd edn (ed. Kani, B.) (WileyBlackwell, 1997).
Zhang, J.-M., Ma, F. & Xu, K.-W. Calculation of the surface energy of FCC metals with modified embedded-atom method. Appl. Surf. Sci. 229 (1), 34–42. https://doi.org/10.1016/j.apsusc.2003.09.050 (2004).
Lousada, C. M. & Korzhavyi, P. A. Hydrogen at symmetric tilt grain boundaries in aluminum: Segregation energies and structural features. Sci. Rep. 12 (1), 19872. https://doi.org/10.1038/s41598-022-23535-9 (2022).
Lausche, A. C. et al. On the effect of coverage-dependent adsorbate–adsorbate interactions for CO methanation on transition metal surfaces. J. Catal. 307 , 275–282. https://doi.org/10.1016/j.jcat.2013.08.002 (2013).
Kitchin, J. R. Correlations in coverage-dependent atomic adsorption energies on Pd(111). Phys. Rev. B 79 (20), 205412. https://doi.org/10.1103/PhysRevB.79.205412 (2009).
Lousada, C. M., Johansson, A. J. & Korzhavyi, P. A. Molecular and dissociative adsorption of water at a defective Cu(110) surface. Surf. Sci. 658 , 1–8. https://doi.org/10.1016/j.susc.2016.11.012 (2017).
Lousada, C. M., Johansson, A. J. & Korzhavyi, P. A. Molecular and dissociative adsorption of water and hydrogen sulfide at perfect and defective Cu(110) surfaces. Phys. Chem. Chem. Phys. 19 (11), 8111–8120. https://doi.org/10.1039/c6cp07732c (2017).
Eone, J. R. A magnetic tight-binding model: Surface properties of transition metals and cobalt nanoparticules. 2022, hal-01949869v3. https://hal.science/hal-01949869v3 (2022).
Per, H. & Mats, P. Long-ranged adsorbate-adsorbate interactions mediated by a surface-state band. J. Phys.Condens. Matter. 12 (1), L13. https://doi.org/10.1088/0953-8984/12/1/103 (2000).
Stepanyuk, V. S. et al. Quantum interference and long-range adsorbate-adsorbate interactions. Phys. Rev. B 68 (20), 205410. https://doi.org/10.1103/PhysRevB.68.205410 (2003).
Quaino, P., Juarez, F., Santos, E. & Schmickler, W. Volcano plots in hydrogen electrocatalysis—Uses and abuses. Beilstein J. Nanotechnol. 5 , 846–854. https://doi.org/10.3762/bjnano.5.96 (2014).
Ooka, H., Huang, J. & Exner, K. S. The Sabatier principle in electrocatalysis: Basics, limitations, extensions. Front. Energy Res. https://doi.org/10.3389/fenrg.2021.654460 (2021).
Laplaza, R., Das, S., Wodrich, M. D. & Corminboeuf, C. Constructing and interpreting volcano plots and activity maps to navigate homogeneous catalyst landscapes. Nat. Protoc. 17 (11), 2550–2569. https://doi.org/10.1038/s41596-022-00726-2 (2022).
Laursen, A. B. et al. Electrochemical hydrogen evolution: Sabatier’s principle and the volcano plot. J. Chem. Educ. 89 (12), 1595–1599. https://doi.org/10.1021/ed200818t (2012).
Trasatti, S. Work function, electronegativity, electrochemical behaviour of metals: III. Electrolytic hydrogen evolution in acid solutions. J. Electroanal. Chem. Interfac. Electrochem. 39 (1), 163–184. https://doi.org/10.1016/S0022-0728(72)80485-6 (1972).
Derry, G. N., Kern, M. E. & Worth, E. H. Recommended values of clean metal surface work functions. J. Vacuum Sci. Technol. A 33 (6), 060801. https://doi.org/10.1116/1.4934685 (2015).
Bronsted, J. N. Acid and basic catalysis. Chem. Rev. 5 (3), 231–338. https://doi.org/10.1021/cr60019a001 (1928).
Evans, M. G. & Polanyi, M. Inertia and driving force of chemical reactions. Trans. Faraday Soc. https://doi.org/10.1039/TF9383400011 (1938).
Bligaard, T. et al. The Brønsted–Evans–Polanyi relation and the volcano curve in heterogeneous catalysis. J. Catal. 224 (1), 206–217. https://doi.org/10.1016/j.jcat.2004.02.034 (2004).
Nørskov, J. K. et al. Trends in the exchange current for hydrogen evolution. J. Electrochem. Soc. 152 (3), J23. https://doi.org/10.1149/1.1856988 (2005).
Lousada, C. M., Johansson, A. J. & Korzhavyi, P. A. Cover feature: Adsorption of hydrogen sulfide, hydrosulfide and sulfide at Cu(110)—Polarizability and cooperativity effects first stages of formation of a sulfide layer. ChemPhysChem 19 (17), 2120–2120. https://doi.org/10.1002/cphc.201800756 (2018).
Rojas, K. I. M., Villagracia, A. R. C., Narido, S. C., Moreno, J. L. V. & Arboleda, N. B. First principles study of H 2 adsorption on Ni-decorated silicene. Mater. Res. Express 6 (5), 055509. https://doi.org/10.1088/2053-1591/ab0294 (2019).
Watkins, W. L. & Borensztein, Y. Mechanism of hydrogen adsorption on gold nanoparticles and charge transfer probed by anisotropic surface plasmon resonance. Phys. Chem. Chem. Phys. 19 (40), 27397–27405. https://doi.org/10.1039/C7CP04843B (2017).
Kayode, G. O., Zhang, S. & Montemore, M. M. Linking electronic structure to adsorption energies: metal surfaces and single-atom catalysts. In Catalysis (eds Spivey, J. et al. ) 34 (The Royal Society of Chemistry, 2022).
Schumann, J., Stamatakis, M., Michaelides, A. & Réocreux, R. Ten-electron count rule for the binding of adsorbates on single-atom alloy catalysts. Nat. Chem. 16 (5), 749–754. https://doi.org/10.1038/s41557-023-01424-6 (2024).
Venkataramanan, N. S., Suvitha, A., Mizuseki, H. & Kawazoe, Y. A theoretical study of the effects of transition metal dopants on the adsorption and dissociation of hydrogen on nickel clusters. Int. J. Quantum Chem. 113 (15), 1940–1948. https://doi.org/10.1002/qua.24418 (2013).
Hammer, B. & Nørskov, J. K. Electronic factors determining the reactivity of metal surfaces. Surf. Sci. 343 (3), 211–220. https://doi.org/10.1016/0039-6028(96)80007-0 (1995).
Wang, G.-C., Li, J., Xu, X.-F., Li, R.-F. & Nakamura, J. The relationship between adsorption energies of methyl on metals and the metallic electronic properties: A first-principles DFT study. J. Comput. Chem. 26 (9), 871–878. https://doi.org/10.1002/jcc.20225 (2005).
Bligaard, T. & Nørskov, J. K. Chapter 4—heterogeneous catalysis. In Chemical Bonding at Surfaces and Interfaces (eds Nilsson, A. et al. ) 255–321 (Elsevier, 2008).
Chapter Google Scholar
Nilsson, A. & Pettersson, L. G. M. Chapter 2—Adsorbate electronic structure and bonding on metal surfaces. In Chemical Bonding at Surfaces and Interfaces (eds Nilsson, A. et al. ) 57–142 (Elsevier, 2008).
Geerlings, P., De Proft, F. & Langenaeker, W. Conceptual density functional theory. Chem Rev. 103 (5), 1793–1874. https://doi.org/10.1021/cr990029p (2003).
Download references
Acknowledgements
Cláudio M. Lousada acknowledges financial support from the Erasmus+ program. The computations were performed on resources provided by the Swedish National Infrastructure for Computing (SNIC) and NAISS at the High-Performance Computing Center North (HPC2N), Umeå and at the PDC Center for High Performance Computing at the KTH—Royal Institute of Technology, Stockholm, partially funded by the Swedish Research Council through grant agreement no. 2018-05973 and partially funded by the Swedish Research Council through grant agreement no. 2022-06725.
Open access funding provided by Royal Institute of Technology.
Author information
Authors and affiliations.
Department of Materials Science and Engineering, KTH Royal Institute of Technology, SE-100 44, Stockholm, Sweden
Cláudio M. Lousada & Atharva M. Kotasthane
You can also search for this author in PubMed Google Scholar
Contributions
CML conceptualized the study, performed the computations, treated the data, and wrote the first draft. AK performed the literature search, treated the data, and wrote the first draft. All authors have revised and approved the text.
Corresponding author
Correspondence to Cláudio M. Lousada .
Ethics declarations
Competing interests.
The authors declare no competing interests.
Additional information
Publisher's note.
Springer Nature remains neutral with regard to jurisdictional claims in published maps and institutional affiliations.
Supplementary Information
Supplementary information., rights and permissions.
Open Access This article is licensed under a Creative Commons Attribution 4.0 International License, which permits use, sharing, adaptation, distribution and reproduction in any medium or format, as long as you give appropriate credit to the original author(s) and the source, provide a link to the Creative Commons licence, and indicate if changes were made. The images or other third party material in this article are included in the article's Creative Commons licence, unless indicated otherwise in a credit line to the material. If material is not included in the article's Creative Commons licence and your intended use is not permitted by statutory regulation or exceeds the permitted use, you will need to obtain permission directly from the copyright holder. To view a copy of this licence, visit http://creativecommons.org/licenses/by/4.0/ .
Reprints and permissions
About this article
Cite this article.
Lousada, C.M., Kotasthane, A.M. Hydrogen adsorption on fcc metal surfaces towards the rational design of electrode materials. Sci Rep 14 , 20972 (2024). https://doi.org/10.1038/s41598-024-71703-w
Download citation
Received : 10 April 2024
Accepted : 30 August 2024
Published : 09 September 2024
DOI : https://doi.org/10.1038/s41598-024-71703-w
Share this article
Anyone you share the following link with will be able to read this content:
Sorry, a shareable link is not currently available for this article.
Provided by the Springer Nature SharedIt content-sharing initiative
By submitting a comment you agree to abide by our Terms and Community Guidelines . If you find something abusive or that does not comply with our terms or guidelines please flag it as inappropriate.
Quick links
- Explore articles by subject
- Guide to authors
- Editorial policies
Sign up for the Nature Briefing newsletter — what matters in science, free to your inbox daily.


IMAGES
VIDEO
COMMENTS
1 Q-tip. A small quantity of liquid soap. Required steps. Take the paper clip and place it on top of the water surface of the glass. Try to balance it on the water surface. If it sinks, take it out from the glass. Now, place the piece of small tissue paper on the water surface and then put the paper clip on it.
Surface tension exists on the surface of water because water molecules like to stick to each other. This force is so strong that it can help things sit on top of the water instead of sinking into it, like our pepper and soap experiment below. The high surface tension of water allows a paper clip, with much higher density, to float on water.
Surface tension is a fascinating phenomenon that is often overlooked. It is the force that allows insects to walk on water, and it is also responsible for the formation of bubbles. In this article, you will learn about the science behind the interesting force and how to perform simple surface tension experiments to demonstrate its effects.. Surface tension is a powerful force that can have ...
Another surface tension experiment is where you make a shape on the surface of the water with cocktail sticks and drop some washing-up liquid in the centre to force the sticks apart. Watch how water behaves on the space station with this NASA video. Try filling a bowl half full with water and carefully placing a paperclip on the top, so it floats.
Surface tension holds the surface molecules of liquids tightly together and makes for some fun experiments! Instagram: http://instagram.com/thephysicsgirlFac...
Kids can place the paperclip gently on the surface of the water. They'll observe how the water's surface tension allows the paperclip to float. #2 - Magic Milk. For this experiment, you'll need a shallow dish, milk, food coloring, and liquid dish soap. Pour a small amount of milk into the dish, add a few drops of different food coloring ...
Surface tension is defined as the amount of energy required to increase the surface area of a liquid by a unit amount. ... In this experiment, you will be making and using a single beam balance to measure the force exerted by surface tension on a needle, floating on the surface of the water. ... This can be a simple box or dish folded from ...
Place your penny on a flat, level surface that can get a little wet, like a kitchen counter. Fill a glass, cup, or small bowl with tap water. Fill the medicine dropper with water. Now, carefully add one drop of water at a time to the top of the penny. Hold the medicine dropper just above the top of the penny (not touching it), so each new drop ...
A plate, a glass, a penny, an index card, a paperclip, an eye dropper, a cup of coffee, dish soap, or some food coloring… and if you're teaming up with an adult, get a pan and stove, too. Surface tension is the energy, or work, required to increase the surface area of a liquid due to intermolecular forces. Since these intermolecular forces ...
WATER TENSION EXPERIMENT STEPS. Step 1: Fill up a cup or bowl of water. Plain tap water works great. Step 2: Take some metal paperclips and drop them into the bottom of the water to demonstrate how metal paperclips have a density greater than water so they sink. Show how metal paperclips sink in water. Step 3: Carefully place a metal paperclip ...
Water Drop Surface Tension Experiment. How to conduct water drop races. Set out a large piece of waxed paper on a flat surface. This is your race track. If you like, mark out a starting and finishing line. Using the water dropper, transfer several drops of water to the starting line.
Teach kids about science with a simple science experiment that will leave kids curious and ready to experiment. This surface tension experiment uses a couple simple materials to help teach surface tension for kids in only 5 minutes! Use this surface tension experiments with kindergarten, pre-k, first grade, 2nd grade, 3rd grade, 4th grade, 5th grade, and 6th grade students.
Learning Science Kindergartners Preschoolers Toddlers Experiment Kitchen Water Activities 4 Comments. Alisha shares this super simple surface tension science experiment for kids will leave your kiddos amazed!. In my home, we call this the Magic Finger trick. It's a great way to get your toddlers, preschoolers, and school-aged kiddos interested in the wonders and joys of science.
We always enjoy all types of science experiments for kids!Our latest science experiment is all about exploring the surface tension of water and other liquids. This simple experiment for kids really intrigued my 5 year old and inspired her to come up with all kinds of ideas!. Follow our Science for Kids Pinterest board!. Whenever I present my kids with science experiments, my main goal is to ...
More surface tension experiments. Try racing lolly sticks across a bath or large container of water. Raising Lifelong Learners has a great activity for making a paperclip float using surface tension. Watch food colouring shoot across a tray of milk. If you enjoyed this investigation, why not try one of my other easy science experiments!
Surface Tension is the ability of a liquid to allow objects to float on top of it. Here's how it works: The molecules in a liquid pull at each other from all directions. This means they have 0 force. The molecules on the surface of a liquid can't be pulled in all directions, though, because the top or surface molecules don't have anything ...
Surface tension is the reason you can fit so much water on the penny. The water molecules attract each other, pulling together so the water doesn't spill. Try this experiment with different-sized coins. Predict how many drops you can fit on a quarter compared with the penny. For one final surface tension experiment, start with a full glass of ...
Next try the classic surface tension experiment of floating a sewing needle on top of the water. To make it easier, place a small piece of the paper towel on the water first, and then place the needle on the paper towel. The paper towel should sink to the bottom and leave the needle floating on the surface. Try touching the needle, What happens?
Begin learning about bubbles and surface tension with this easy, kid-friendly experiment! You can do this simple science experiment anywhere; all you need is...
This month's simple science project will delve into the world of surface tension of water with a couple of simple experiments. Surface tension is a barrier formed on the surface of water caused by something called "cohesion." Liquids all have this force, a force that holds a material together. Some are stronger than others (liquid mercury ...
Step 2: The Water Tension With Pepper and With Milk. In this third experiment, a plate with water is sprinkled with pepper, the pepper floats thanks to the surface tension and if a drop of dishwashing liquid or soap is added, this breaks up part of the surface tension. And the pepper and push back on the edges of the plate.
Explore surface tension in this super simple and fun little science experiment. This is a great activity for kids to have fun learning about surface tension....
What Is Surface Tension? Definition and Experiments
where E (nH-M_slab) is the energy of n H-atoms bound to a specific surface of a metal M; E H is the energy of an H-atom in vacuum and E M_slab is the energy of the supercell containing the bare ...