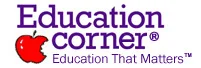

Top 7 Science Experiments with Hydrogen Peroxide

Welcome to our carefully curated collection of hydrogen peroxide science experiments. This roundup invites you to journey through experiments showing you this simple compound’s versatile nature.
Hydrogen peroxide is a common household item known for its antiseptic properties. Yet, beneath its seemingly mundane identity lies a treasure trove of chemical wonders waiting to be explored. With its reactive nature and ability to break down into simpler molecules, hydrogen peroxide is a captivating subject for many scientific experiments.
Note : Students should know the concentration of hydrogen peroxide and understand its potential hazards. These experiments should be conducted in a controlled manner, adhering to the provided procedure and under the supervision of an adult.
1. Elephant Toothpaste
One experiment that is sure to captivate the minds of both students and teachers alike is the famous “Elephant Toothpaste” experiment using hydrogen peroxide.
Elephant Toothpaste experiment is a must-try for any classroom, sparking excitement and curiosity while reinforcing fundamental chemistry principles.
2. Genie in a Bottle
This experiment is an absolute must-try for students, as it offers a hands-on journey into the world of chemical reactions.
By delving into “Genie in a Bottle,” you’ll unleash your curiosity, hone critical thinking skills, and witness the power of chemistry firsthand.
3. DIY Pasta Rocket Engine
The DIY Pasta Rocket Engine experiment using hydrogen peroxide (H2O2) is a captivating and exciting activity that students and teachers should definitely try.
This experiment provides an excellent opportunity for students to explore the principles of chemical reactions, combustion, and propulsion in a hands-on and engaging manner.
4. Remove Stains Using Hydrogen Peroxide
Learning how to remove stains using hydrogen peroxide is a practical and useful experiment that both students and teachers should try. Hydrogen peroxide possesses excellent stain-removing properties due to its oxidizing nature, making it a valuable tool for tackling a wide range of stains.
5. Flame Light Relight – Science Magic
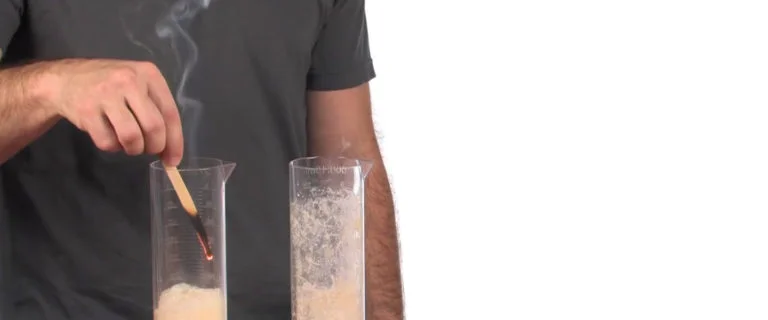
The Flame Light Relight experiment is an intriguing and educational experience that students and teachers should approach with caution.
By engaging in the Flame Light Relight experiment responsibly, students can gain a deeper understanding of the science behind fire and chemical reactions while reinforcing the importance of safety measures and responsible experimentation.
Learn more: Flame Light Relight
6. Potato Catalyzed H2O2 Decomposition
The Potato Catalyzed H2O2 Decomposition experiment is a fascinating and educational activity that students and teachers should definitely try. In this experiment, the natural enzymes present in a potato act as a catalyst to accelerate the decomposition of hydrogen peroxide.
7. Boiled Versus Fresh Liver with Hydrogen Peroxide
The Boiled Versus Fresh Liver with Hydrogen Peroxide experiment is a captivating and informative activity that students and teachers should consider trying.
By comparing the reaction of hydrogen peroxide with boiled and fresh liver, students can explore the effects of heat on enzymatic activity.
Similar Posts:
- 68 Best Chemistry Experiments: Learn About Chemical Reactions
- 35 of the BEST Educational Apps for Teachers (Updated 2024)
- 37 Water Science Experiments: Fun & Easy
Leave a Comment Cancel reply
Save my name and email in this browser for the next time I comment.
Sciencing_Icons_Science SCIENCE
Sciencing_icons_biology biology, sciencing_icons_cells cells, sciencing_icons_molecular molecular, sciencing_icons_microorganisms microorganisms, sciencing_icons_genetics genetics, sciencing_icons_human body human body, sciencing_icons_ecology ecology, sciencing_icons_chemistry chemistry, sciencing_icons_atomic & molecular structure atomic & molecular structure, sciencing_icons_bonds bonds, sciencing_icons_reactions reactions, sciencing_icons_stoichiometry stoichiometry, sciencing_icons_solutions solutions, sciencing_icons_acids & bases acids & bases, sciencing_icons_thermodynamics thermodynamics, sciencing_icons_organic chemistry organic chemistry, sciencing_icons_physics physics, sciencing_icons_fundamentals-physics fundamentals, sciencing_icons_electronics electronics, sciencing_icons_waves waves, sciencing_icons_energy energy, sciencing_icons_fluid fluid, sciencing_icons_astronomy astronomy, sciencing_icons_geology geology, sciencing_icons_fundamentals-geology fundamentals, sciencing_icons_minerals & rocks minerals & rocks, sciencing_icons_earth scructure earth structure, sciencing_icons_fossils fossils, sciencing_icons_natural disasters natural disasters, sciencing_icons_nature nature, sciencing_icons_ecosystems ecosystems, sciencing_icons_environment environment, sciencing_icons_insects insects, sciencing_icons_plants & mushrooms plants & mushrooms, sciencing_icons_animals animals, sciencing_icons_math math, sciencing_icons_arithmetic arithmetic, sciencing_icons_addition & subtraction addition & subtraction, sciencing_icons_multiplication & division multiplication & division, sciencing_icons_decimals decimals, sciencing_icons_fractions fractions, sciencing_icons_conversions conversions, sciencing_icons_algebra algebra, sciencing_icons_working with units working with units, sciencing_icons_equations & expressions equations & expressions, sciencing_icons_ratios & proportions ratios & proportions, sciencing_icons_inequalities inequalities, sciencing_icons_exponents & logarithms exponents & logarithms, sciencing_icons_factorization factorization, sciencing_icons_functions functions, sciencing_icons_linear equations linear equations, sciencing_icons_graphs graphs, sciencing_icons_quadratics quadratics, sciencing_icons_polynomials polynomials, sciencing_icons_geometry geometry, sciencing_icons_fundamentals-geometry fundamentals, sciencing_icons_cartesian cartesian, sciencing_icons_circles circles, sciencing_icons_solids solids, sciencing_icons_trigonometry trigonometry, sciencing_icons_probability-statistics probability & statistics, sciencing_icons_mean-median-mode mean/median/mode, sciencing_icons_independent-dependent variables independent/dependent variables, sciencing_icons_deviation deviation, sciencing_icons_correlation correlation, sciencing_icons_sampling sampling, sciencing_icons_distributions distributions, sciencing_icons_probability probability, sciencing_icons_calculus calculus, sciencing_icons_differentiation-integration differentiation/integration, sciencing_icons_application application, sciencing_icons_projects projects, sciencing_icons_news news.
- Share Tweet Email Print
- Home ⋅
- Science Fair Project Ideas for Kids, Middle & High School Students ⋅
Hydrogen Peroxide Experiments
The Effects of pH on Catechol Oxidase
Chemically, hydrogen peroxide has a similar composition to water, except its molecule has an additional oxygen atom. Simple experiments, some of which you can do at home, involve breaking down hydrogen peroxide into water and oxygen, using catalysts to quicken the reaction. Other experiments show the presence of oxygen. Hydrogen peroxide, in combination with other products, can produce visible chemical reactions.
TL;DR (Too Long; Didn't Read)
You can do simple experiments with drugstore hydrogen peroxide at home, breaking it down into water and oxygen.
Hydrogen Peroxide and Yeast
Hydrogen peroxide is relatively unstable, so over time it breaks down into water and oxygen. In this experiment, yeast is added to hydrogen peroxide to speed up its decomposition process, which is normally slow. You can perform the experiment at home in a sink. You'll need an empty large soda bottle, 3 percent hydrogen peroxide from a grocery store, one packet of active yeast, liquid dish soap and warm water. Mix about 113 grams (4 ounces) of the hydrogen peroxide with 56 grams (2 ounces) of dish soap in the soda bottle. Set aside and mix the packet of yeast with warm water, letting it sit for about five minutes. Pour the yeast mixture into the soda bottle. The reaction produces oxygen gas and the addition of liquid detergent creates foam.
Hydrogen Peroxide and Bleach
The mixture of hydrogen peroxide and bleach creates oxygen gas, salt (sodium chloride) and water. The bleach must contain sodium hypochlorite for this experiment to work. The solutions do not need to be concentrated to get a quick reaction. You will need 3 percent hydrogen peroxide, approximately 6 percent household bleach and a beaker. Pour 56 grams (2 ounces) of bleach into the beaker and the equivalent of hydrogen peroxide. Once the two are mixed, the reaction will occur quickly, producing bubbling.
Hydrogen Peroxide and Burning Sulfur
This experiment doesn't decompose hydrogen peroxide but merely shows that it contains oxygen. You expose a rose to burning sulfur and then dip it in hydrogen peroxide. You'll need two drinking cups, a rose with a small stem, tape, foil, sulfur and hydrogen peroxide. Tape the rose to the inside of the first cup and place a small pile of sulfur on a piece of aluminum foil. Add flame to the sulfur until it starts to smolder -- turn the cup with the rose upside down over the burning sulfur. The rose is exposed to sulfur dioxide gas, turning the petals of the rose to white as the gas combines with the oxygen in the colored part of the rose. Remove the rose from the cup and dip it into a cup filled halfway with hydrogen peroxide. The hydrogen peroxide provides oxygen to the flower, restoring its color.
Safety Considerations
Make sure to wear protective eyewear when conducting any of these experiments, whether at home or in a classroom or lab setting. If hydrogen peroxide comes in contact with your eyes, it can result in damage or blindness. It is imperative to seek medical attention if this happens. Make sure to wear an apron and clothing that covers your skin. According to the Agency for Toxic Substances and Disease Registry website, hydrogen peroxide can cause skin irritation -- there may also be skin burns with blisters with exposure to concentrated solutions. The peroxide you buy in the drug store is typically 3 percent, whereas chemists and other professionals might use stronger concentrations of 35 to 50 percent. Flush your skin with water if it is exposed to hydrogen peroxide.
Related Articles
Simple photosynthesis activities, how-to science experiments for kids with iodine and..., chemical reactions that cause color change, fun chemistry experiments for high schools, school projects with magnesium, how to make a copper sulfate solution, how to make a volcano out of cardboard, exploding experiments for kids, experiments with salt and vinegar, how to make colorful smoke bombs, science fair project ideas on candles, easy science projects for kindergarten, chemical reaction experiments for middle school students, chemistry projects with lemon, food coloring experiments, how to make a bromothymol blue solution, how to make an ecosystem for kids with pop bottles, test tube science experiments for kids, how to extract dna from oranges.
- Agency for Toxic Substances and Disease Registry: ToxFAQs for Hydrogen Peroxide
- Lansing Community College: The reaction of bleach and hydrogen peroxide
About the Author
Based in New Hamburg, Ontario, Mary Margaret Peralta has been writing for websites since 2010. She has developed a company website and a health and safety manual for a past employer. Peralta obtained her Bachelor of Arts in sociology from the University of Waterloo in Waterloo, Ontario.
Find Your Next Great Science Fair Project! GO
FREE SHIPPING ON ORDERS OF $125 or MORE!!!
Phone: 602-584-5845 | Contact Us
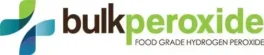
Fun DIY Science Experiments for Kids at Home| Hydrogen Peroxide
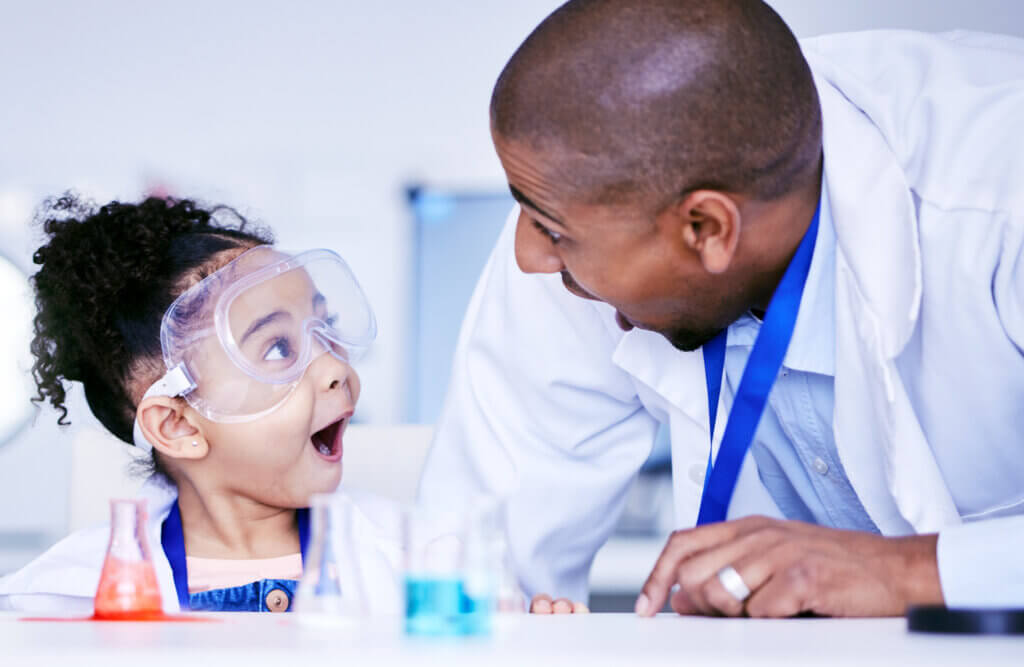
Table of Contents
Essential safety precautions for diy science projects with hydrogen peroxide.
Engaging kids in DIY science projects can be a thrilling family activity, sparking wonder and providing cool science experiments that enrich a child’s learning experience. Conducting fun science experiments at home or in a school lab transforms the theoretical into tangible fun science activities. Hydrogen peroxide, a common household compound found in many medicine cabinets and stores, often stars in these simple and easy homemade experiments due to its reactive properties. However, it’s vitally important to adhere to safety precautions to ensure these fun experiments remain a source of joy and not harm. Let’s explore the essential safety measures that must be followed before embarking on any science experiment involving hydrogen peroxide.
Firstly, children should always be supervised by an adult when conducting any cool science experiment. Kids’ natural curiosity can sometimes lead them to venture into unsafe practices which will need an adult’s guidance to navigate. Simple, yet effective, safety gear such as gloves, goggles, and aprons should be worn to protect skin and eyes from potential splashes during these fun science activities. A lab coat or an old shirt can also serve as a barrier between the children’s clothing and any spills. It is also wise to prepare the experiment area by laying down newspaper or plastic covers, not just for easy clean-ups, but also to protect surfaces from any accidental hydrogen peroxide contact.
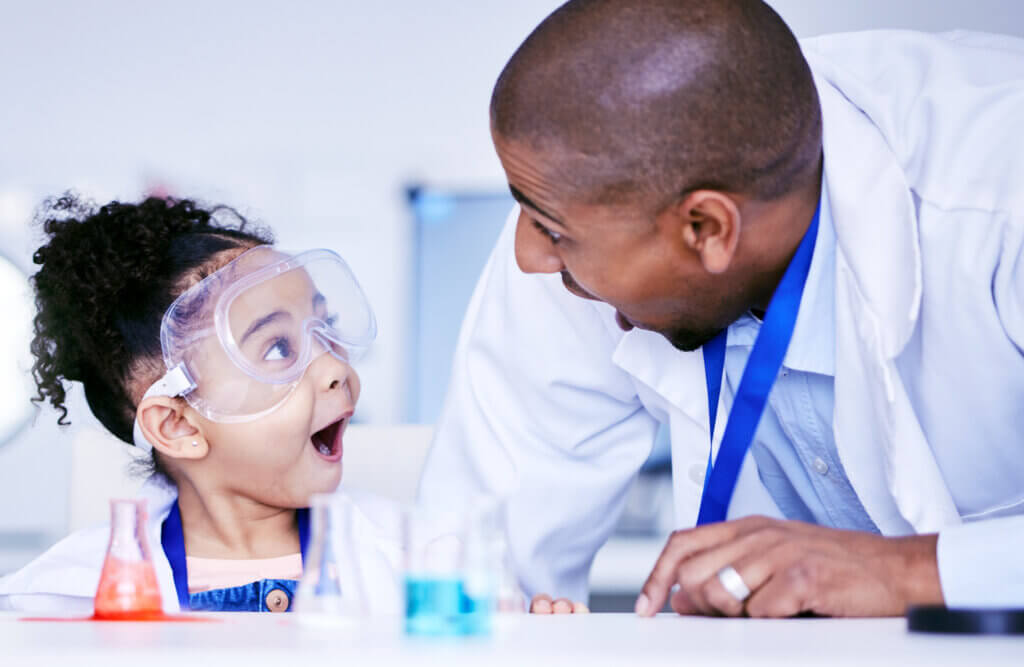
When you’re ready to start your homemade fun experiment with hydrogen peroxide, always ensure you’re in a well-ventilated area, whether it’s a room with open windows or outdoors. This helps to dissipate any fumes that may be produced. It’s also crucial to use only the concentrations of hydrogen peroxide recommended in the experiment’s guidelines, which are typically available in a science project book or reputable online resources. Store-bought hydrogen peroxide is usually a 3% solution, which is generally safe for use in these home science experiments but still requires caution.
By rigorously following these precautions, you can continually create safe and entertaining environments for fun science experiments with kids. Whether you’re a parent, teacher, or simply a science enthusiast, fostering a love for science in an easy and responsible manner is a rewarding endeavor. Through these cool science experiments with hydrogen peroxide, children not only learn scientific principles but also the importance of caution and respect for chemicals, even during fun science activities — lessons that are invaluable both inside and outside the lab.
Elephant Toothpaste
How it Works
Elephant toothpaste is a captivating and visually striking experiment that showcases a rapid decomposition reaction. The primary ingredients involved are hydrogen peroxide (H2O2), a catalyst typically in the form of potassium iodide (KI), and a surfactant like dish soap. When hydrogen peroxide is mixed with the catalyst, it rapidly decomposes into water (H2O) and oxygen gas (O2). This reaction is exothermic, meaning it releases heat, and the soap helps trap the oxygen gas bubbles, creating the foamy eruption resembling toothpaste squirting out of a tube. The potassium iodide serves as a catalyst, expediting the breakdown of hydrogen peroxide into water and oxygen. This vibrant and engaging demonstration vividly illustrates the fascinating chemical reaction between hydrogen peroxide and the catalyst, resulting in an exciting eruption of foam.
What You Need
- 35% hydrogen peroxide
- Food coloring
- A small spoonful of yeast
- Lots of space
- A mixing utensil
- A clear jar/cup
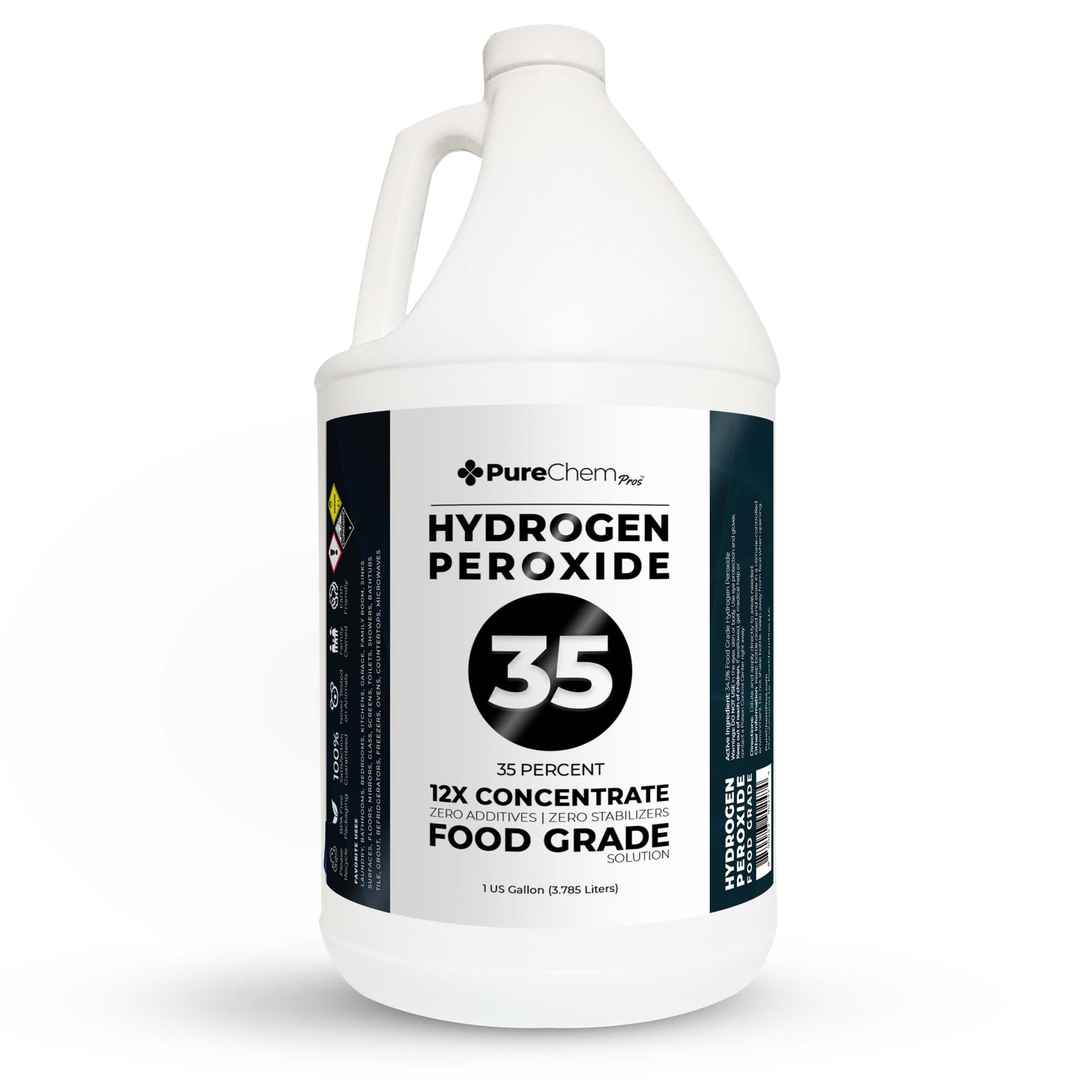
Step-by-Step Walkthrough
- Safety First: Put on your protective gear – goggles and gloves are a must. Even a small amount of hydrogen peroxide can cause irritation, so ensure safety measures are in place.
- Prepare the Workspace: Choose a spacious area as this experiment tends to create a foam eruption. Clear a table or designated space to conduct the experiment safely.
- Gather Materials : Collect all the necessary materials – a clear jar or cup, 3% hydrogen peroxide , food coloring (optional), dish soap, a favorite fragrance (optional), yeast, warm water, a mixing spoon, and a whisk.
- Set Up the Jar/Cup: Pour 3% hydrogen peroxide into the clear jar or cup. Add a few drops of dish soap for a colorful effect. Optionally, add a few drops of your chosen fragrance to enhance the experience. Gently mix these components together.
- Prepare the Yeast Solution: Create a yeast solution by mixing yeast with warm water until it’s thoroughly dissolved.
- Combine Ingredients : Once the yeast solution is ready, swiftly pour it into the jar or cup containing the hydrogen peroxide mixture. Stir the ingredients together, ensuring they’re well combined.
- Observe the Reaction: Step back and watch the magical chemical reaction unfold! As the yeast catalyzes the decomposition of hydrogen peroxide, bubbles of oxygen are released, resulting in a dramatic foamy eruption resembling toothpaste.
Caution: Maintain a safe distance and be prepared for the colorful, bubbly eruption that will take over the jar or cup, showcasing the impressive ‘elephant toothpaste’ effect.
Remember, safety precautions are crucial, and handling chemicals should be done with care and attention to prevent any mishaps. Enjoy the exciting visual display of this scientific experiment!
Creating a Volcano Lava Potion
The Volcano Lava Potion experiment demonstrates the rapid decomposition of hydrogen peroxide when mixed with a catalyst. The catalyst initiates the breakdown of hydrogen peroxide into water and oxygen gas. This decomposition reaction is highly exothermic, releasing heat, and the dish soap helps to trap the oxygen gas released, creating a foamy eruption. The foaming action showcases the liberation of oxygen as the hydrogen peroxide breaks down, resulting in an exciting visual representation of a chemical reaction. This experiment not only entertains but also educates about chemical reactions in a captivating and engaging manner, making it a thrilling learning experience for kids and adults alike.
- Clean bottle
- Hydrogen peroxide
- Catalyst (yeast or potassium iodide)
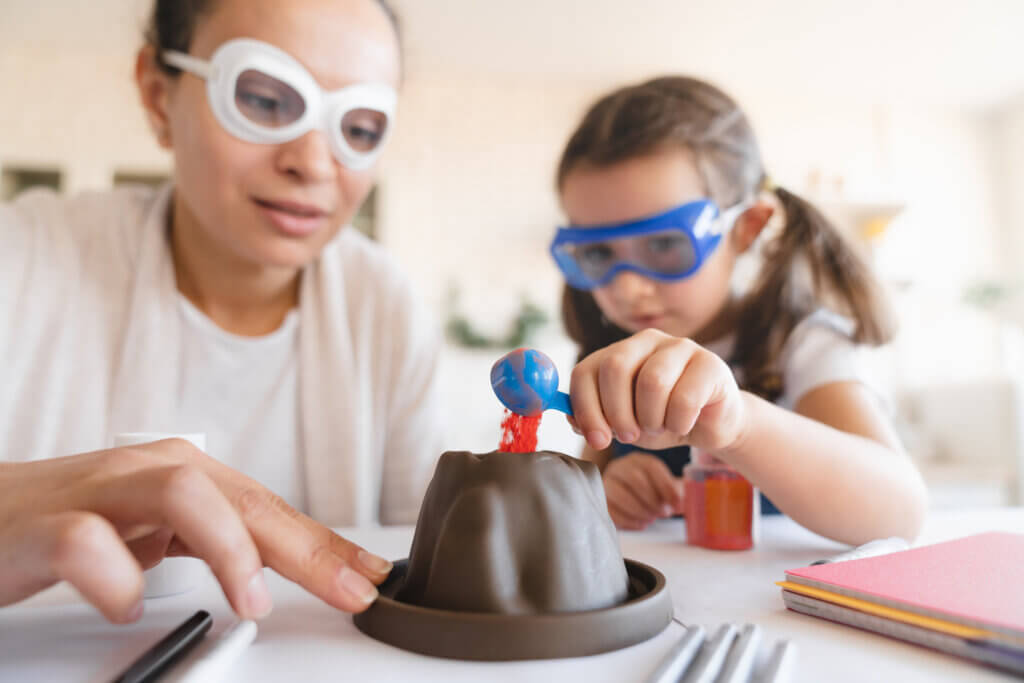
- Safety First: Ensure safety measures are in place, including wearing safety goggles and preparing the workspace.
- Set Up the Jar/Cup: Take a clean bottle and add a portion of hydrogen peroxide into it.
- Combine liquids : Add a squirt of dish soap into the bottle, which will trap the oxygen released during the reaction and create foam.
- Combine Ingredients: Introduce the catalyst, either yeast or potassium iodide, to kick-start the reaction. You can use a diluted solution of the catalyst for this purpose.
- Observe the Reaction: Observe the awe-inspiring chemical reaction as the hydrogen peroxide decomposes rapidly, generating oxygen, and resulting in a foamy eruption resembling a volcano’s lava flow.
Creating a Piranha Solution
The Piranha Solution may sound fierce, and rightly so—it’s a powerful concoction made by combining concentrated hydrogen peroxide with sulfuric acid.
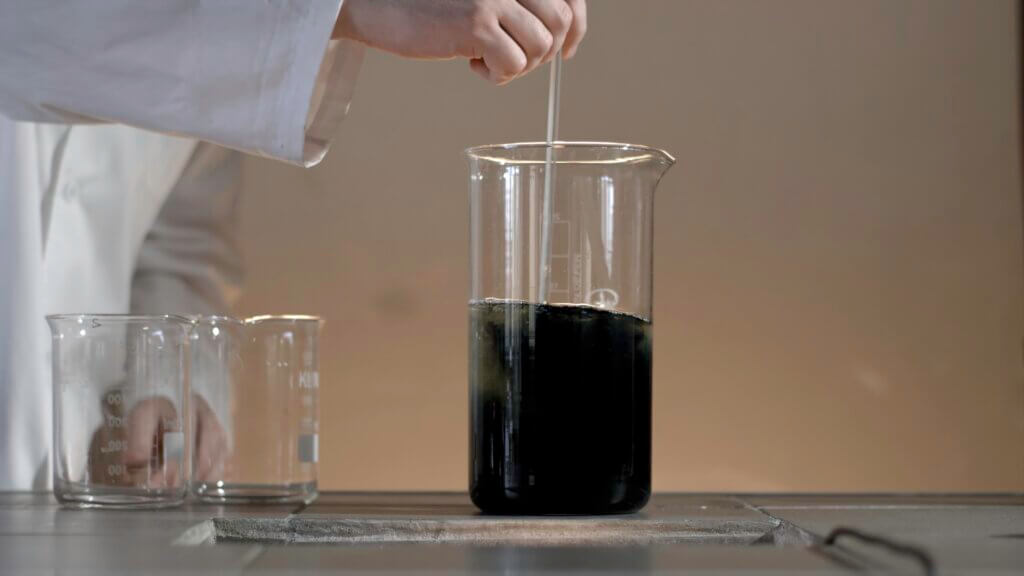
This mix is no ordinary cleaner; it’s like the superhero of lab cleaning solutions, capable of tackling stubborn residues on glassware and surfaces.
When these two ingredients come together, the sulfuric acid triggers the hydrogen peroxide to break down rapidly. This reaction releases a lot of heat and creates oxygen, which, when unleashed, has a remarkable ability to zap away organic residues, leaving surfaces sparkling clean.
However, a word of caution: think of the Piranha Solution as a fire-breathing dragon. It’s incredibly strong and can cause serious harm if mishandled. That’s why it’s crucial to handle it with the utmost care—gloves, goggles, and protective clothing are your knight’s armor when dealing with this potent mix. Always mix the acid into the peroxide slowly and stir gently to prevent an explosive reaction. And after its superhero duty is done, make sure to properly neutralize and dispose of it according to safety guidelines—this powerful solution needs to be handled with respect.
- Safety equipment (gloves, goggles, and protective clothing)
- Hydrogen peroxide (high concentration, typically 35%)
- Sulfuric acid
- Water (for dilution, if necessary)
- Glass or plastic container for mixing
- Stirring rod or glass stirring stick
- Cotton Ball
- Safety First: Prioritize safety by wearing gloves, goggles, and protective clothing before handling any of the chemicals.
- Prepare the Workspace: Choose a well-ventilated area to perform the experiment and ensure a stable work surface.
- Gather Materials : Pour the required amount of hydrogen peroxide into the glass or plastic container. Note: Piranha solution is highly reactive and dangerous; extreme caution is necessary when handling concentrated hydrogen peroxide.
- Combine liquids : Gradually add the sulfuric acid to the hydrogen peroxide. Take extreme care and add the acid slowly while stirring gently with a glass stirring rod or stick. Always pour the acid into the peroxide, not the other way around, to prevent vigorous reactions.
- Observe the Reaction: As the solution reacts vigorously, producing heat and emitting fumes. The mixture will heat up significantly as the reaction progresses, hence the name “Piranha solution.” Next add a cotton ball to the solution. Once the cotton ball comes in contact with the solution it burns right there and then, and the whole jar is taken over a black color. Pretty amazing right? You can spend the whole day adding other fun stuff and watch it burn. Kids love this stuff.
Allow the solution to cool before use, ensuring it’s properly neutralized and safe for disposal according to hazardous waste disposal guidelines
Start a Fire Without Matches
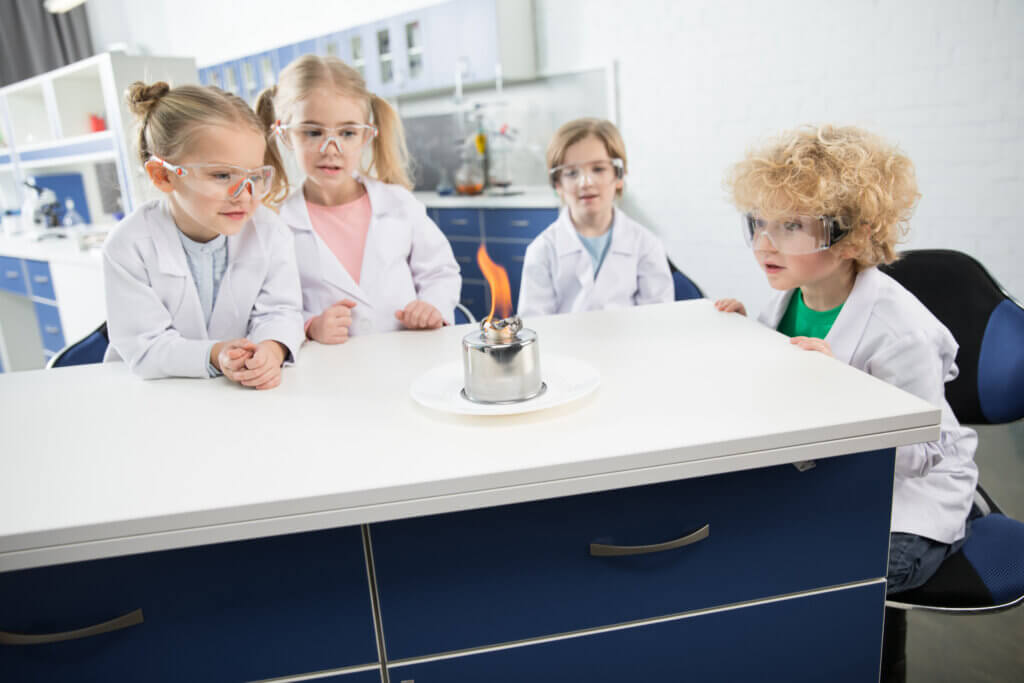
The fascinating reaction that enables starting a fire without matches using hydrogen peroxide and potassium permanganate involves the oxidation of glycerin by hydrogen peroxide. Though glycerin is not explicitly mentioned in the materials, it’s formed as a byproduct when potassium permanganate reacts with hydrogen peroxide. Glycerin is highly susceptible to oxidation and readily undergoes combustion. When the concentrated hydrogen peroxide comes into contact with potassium permanganate, it rapidly decomposes, releasing oxygen. This oxygen, in turn, reacts with the glycerin formed, causing a quick exothermic reaction that produces heat and smoke, resembling the visual effect of fire. The chemical reaction is an illustrative example of how certain compounds, when combined in the right conditions, can create an exciting spectacle that mimics fire without the need for conventional ignition sources like matches.
- Splash-free goggles
- Potassium permanganate
- White lab coat
- A clear small bowl
- Ceramic plate
- Prioritize safety by wearing splash-free goggles and a white lab coat to protect yourself during the experiment.
- Take a small clear bowl and add potassium permanganate into it.
- Using a syringe, fill it with 35% hydrogen peroxide, ensuring it’s not filled up to the brim.
- Place the bowl containing potassium permanganate onto a ceramic plate.
- Slowly and steadily inject the hydrogen peroxide into the bowl by pressing the syringe gently.
- Once the hydrogen peroxide comes into contact with the potassium permanganate, observe as smoke begins to rise, indicating a chemical reaction that mimics fire.
- For a larger-scale experiment, you can use an open large flask following the same procedure as mentioned earlier.
- After completing the experiment, ensure proper ventilation by opening windows to eliminate any burning smell. Additionally, wash the used bowl under lukewarm water to remove any residues.
Potato Catalase Experiment
When hydrogen peroxide (H2O2) comes in contact with an uncooked potato, a fascinating reaction occurs due to the presence of an enzyme called catalase in the potato. Catalase is abundant in living organisms, including plants , and it helps break down hydrogen peroxide into water (H2O) and oxygen (O2) gas. This enzyme speeds up the decomposition of hydrogen peroxide into its constituents, creating bubbles of oxygen gas that you observe as foam. However, in a cooked potato, the high temperature from cooking denatures the catalase enzyme, rendering it inactive. As a result, the cooked potato doesn’t produce the same bubbling reaction with hydrogen peroxide as the enzyme responsible for catalyzing the breakdown of hydrogen peroxide has been altered and can no longer facilitate the reaction.
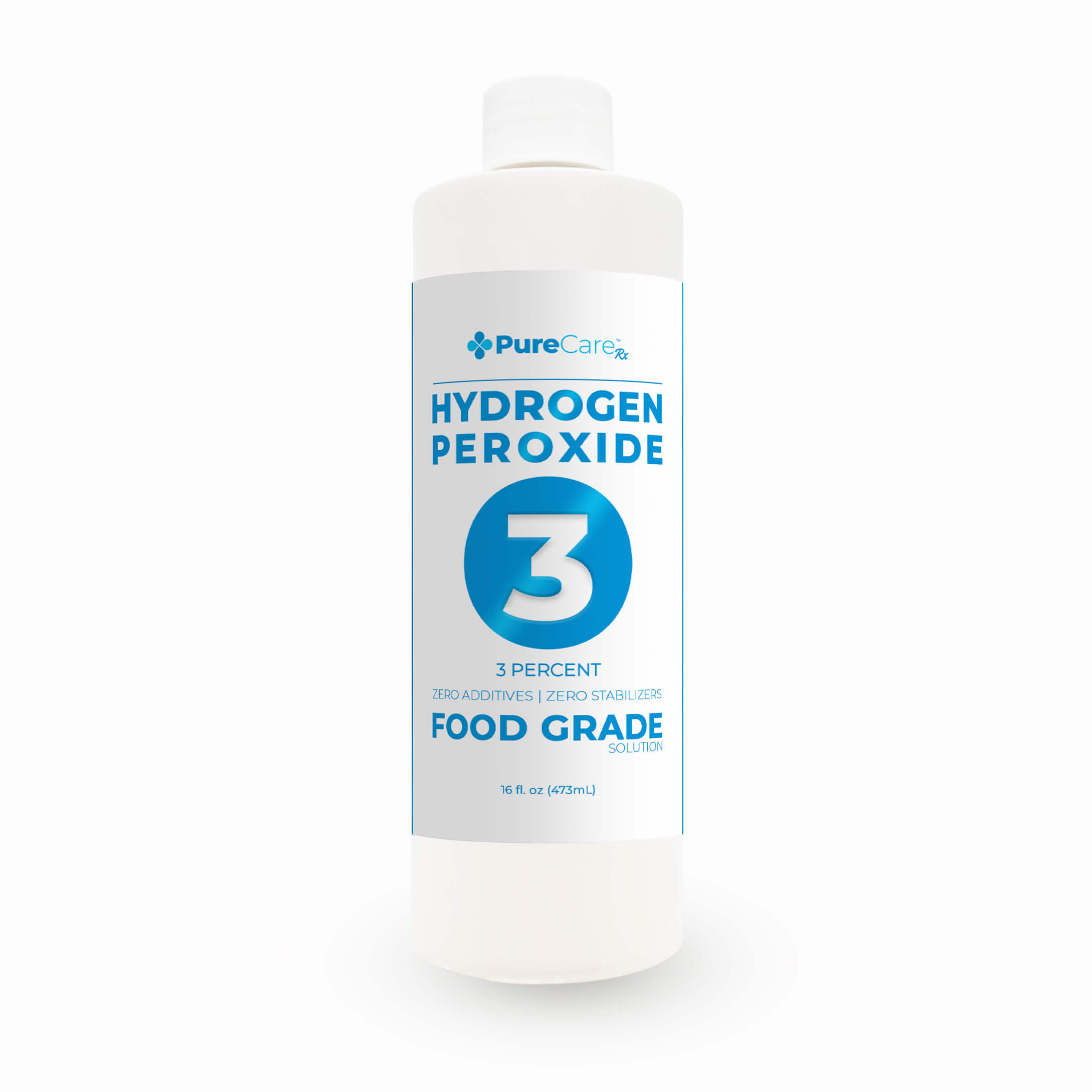
- Hydrogen peroxide 3%
- Uncooked potato
- Cooked potato
- Cutting board and knife
- Bowl or container for mixing
- Spoon or spatula for stirring
- Gather the materials: hydrogen peroxide, an uncooked potato, and a cooked potato.
- Place the uncooked potato on a cutting board and carefully cut it into small pieces or slices.
- Take a separate bowl or container and pour some hydrogen peroxide into it.
- Add the cut pieces or slices of the uncooked potato into the bowl containing hydrogen peroxide.
- Observe as the uncooked potato reacts with the hydrogen peroxide, producing bubbles of oxygen due to the presence of the catalase enzyme.
- Next, cut the cooked potato into similar-sized pieces or slices.
- Place the cut pieces of the cooked potato into a different bowl containing hydrogen peroxide.
- Notice that the cooked potato does not produce the same bubbling reaction as the catalase enzyme in the cooked potato has been altered by the cooking process and cannot catalyze the breakdown of hydrogen peroxide.
Fingerprint Enhancement
This experiment is an entertaining and straightforward way to engage in mind-stimulating activities with children, creating a scenario resembling a crime scene, complete with different characters, to fully immerse in the experience of revealing hidden fingerprints.When 35% hydrogen peroxide is mixed with a small amount of distilled white vinegar, it creates an acidified peroxide solution. This solution has a remarkable ability to react with the natural oils present in human skin, which are often transferred onto surfaces when a person touches them. These latent fingerprints, typically invisible to the naked eye, become visible when exposed to the acidified peroxide solution, making it a handy method for revealing hidden fingerprints on surfaces.
- Distilled white vinegar
- Measurement jar
- Begin by obtaining a measurement jar and pour 35% hydrogen peroxide into it, filling it up to 400 ml.
- Add a small quantity of distilled white vinegar, precisely 1 ml, into the measurement jar containing the hydrogen peroxide.
- For testing purposes, place the object or material to be inspected—such as a ring or a fake bullet—into the jar containing the prepared solution.
- Allow the material to soak in the solution for a duration of 12 minutes.
- After the allotted time, use gloves to retrieve the material from the jar.
- Inspect the material under bright light, and you’ll observe previously unseen fingerprints become visible all over the surface.
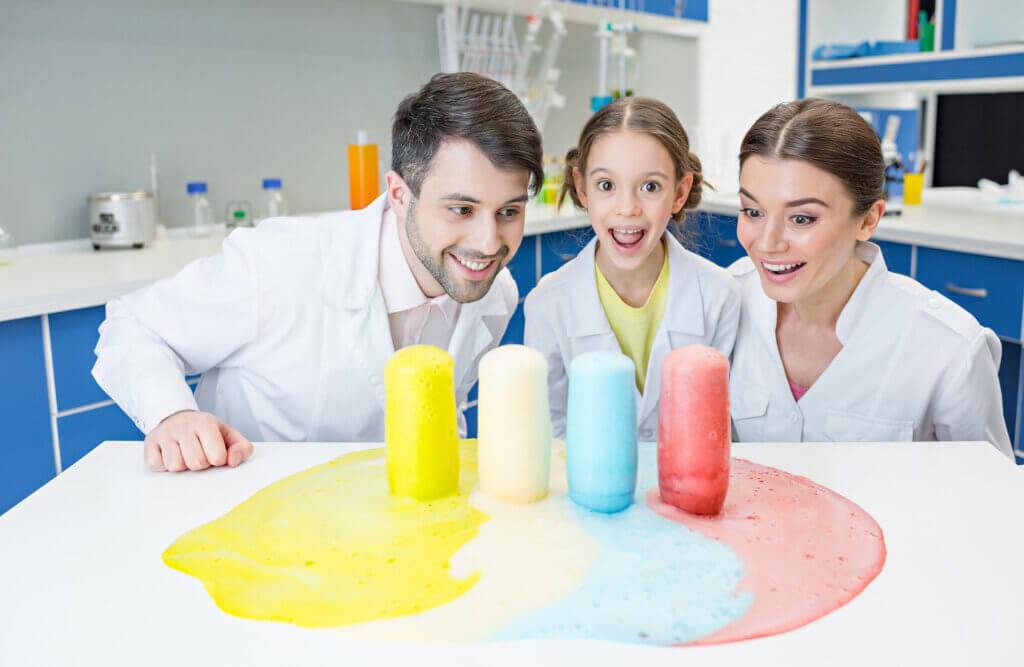
The Takeaway
The experiments detailed above offer engaging and accessible experiences suitable for conducting at home with children, serving as wonderful entry-level introductions to scientific exploration. Safety precautions are paramount when dealing with chemicals, ensuring a secure environment for both you and your children during experimentation. Selecting the appropriate high-quality hydrogen peroxide, specific to the required concentration for these experiments, is crucial. While hydrogen peroxide is commonly found in households, acquiring the right grade and concentration is key to achieving optimal and safe results. Prioritizing purity and quality is essential for a secure and successful experiment.
So, why wait? Secure your kit today for a fulfilling and safe science exploration journey!
Order you set today!
- Disinfecting
- Hydrogen Peroxide
All product reviews are from actual verified buyers. The information and statements made by reviewers are for education purposes and are not intended to be medical advise or to replace the information given by your doctor. Bulk Peroxide does not give medical advice of any kind or diagnose illness. Further we do not advocate the advise of 3rd party references. If you have a medical condition please consult your doctor.
These statements have not been evaluated the Food and Drug Administration (FDA). These products are not intended to diagnose, treat, cure or prevent any disease.
Your browser is not supported
Sorry but it looks as if your browser is out of date. To get the best experience using our site we recommend that you upgrade or switch browsers.
Find a solution
- Skip to main content
- Skip to navigation
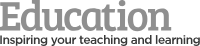
- Back to parent navigation item
- Primary teacher
- Secondary/FE teacher
- Early career or student teacher
- Higher education
- Curriculum support
- Literacy in science teaching
- Periodic table
- Interactive periodic table
- Climate change and sustainability
- Resources shop
- Collections
- Remote teaching support
- Starters for ten
- Screen experiments
- Assessment for learning
- Microscale chemistry
- Faces of chemistry
- Classic chemistry experiments
- Nuffield practical collection
- Anecdotes for chemistry teachers
- On this day in chemistry
- Global experiments
- PhET interactive simulations
- Chemistry vignettes
- Context and problem based learning
- Journal of the month
- Chemistry and art
- Art analysis
- Pigments and colours
- Ancient art: today's technology
- Psychology and art theory
- Art and archaeology
- Artists as chemists
- The physics of restoration and conservation
- Ancient Egyptian art
- Ancient Greek art
- Ancient Roman art
- Classic chemistry demonstrations
- In search of solutions
- In search of more solutions
- Creative problem-solving in chemistry
- Solar spark
- Chemistry for non-specialists
- Health and safety in higher education
- Analytical chemistry introductions
- Exhibition chemistry
- Introductory maths for higher education
- Commercial skills for chemists
- Kitchen chemistry
- Journals how to guides
- Chemistry in health
- Chemistry in sport
- Chemistry in your cupboard
- Chocolate chemistry
- Adnoddau addysgu cemeg Cymraeg
- The chemistry of fireworks
- Festive chemistry
- Education in Chemistry
- Teach Chemistry
- On-demand online
- Live online
- Selected PD articles
- PD for primary teachers
- PD for secondary teachers
- What we offer
- Chartered Science Teacher (CSciTeach)
- Teacher mentoring
- UK Chemistry Olympiad
- Who can enter?
- How does it work?
- Resources and past papers
- Top of the Bench
- Schools' Analyst
- Regional support
- Education coordinators
- RSC Yusuf Hamied Inspirational Science Programme
- RSC Education News
- Supporting teacher training
- Interest groups
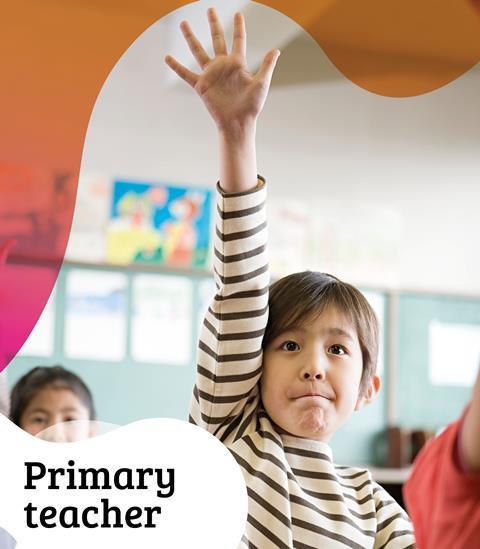
- More navigation items
Hydrogen peroxide decomposition using different catalysts
- Four out of five
- No comments
From fresh liver, to powdered manganese, create different catalysts to explore the effervescent world of hydrogen peroxide decomposition
Your shopping list might look strange, but this practical will be well worth it. Supporting student to understand reaction rates, catalysis, and enzymes.
This experiment should take 5 minutes.
Equipment
- Eye protection
- Measuring cylinders, 250 cm 3 , x1 for each catalyst
- Large tray for spills
- Hydrogen peroxide solution, 75 cm 3 ,100 vol
- Powdered manganese(IV) oxide (manganese dioxide, MnO 2 ), 0.5 g
- Lead(IV) oxide (lead dioxide, PbO 2 ), 0.5 g
- iron(III) oxide (red iron oxide, Fe 2 O 3 ), 0.5 g
- Potato, 1 cm 3
- Liver, 1 cm 3
Health, safety and technical notes
- Read our standard health and safety guidance .
- Always wear eye protection.
- Hydrogen peroxide is corrosive, see CLEAPSS Hazcard HC050 .
- Manganese oxide is harmful if swallowed or inhaled, see CLEAPSS Hazcard HC060 .
- Lead dioxide is a reproductive toxin, harmful if swallowed or inhaled, a Specific Target Organ Toxin and hazardous to the aquatic environment, see CLEAPSS Hazcard HC056 .
- Avoid contact of the catalysts with aluminium and other metal powders, explosive reactions can occur.
Before the demonstration
- Line up five 250 cm 3 measuring cylinders in a tray.
- Add 75 cm 3 of water to the 75 cm 3 of 100 volume hydrogen peroxide solution to make 150 cm 3 of 50 volume solution.
The demonstration
- Place about 1 cm 3 of washing up liquid into each of the measuring cylinders.
- To each one add the amount of catalyst specified above.
- Then add 25 cm 3 of 50 volume hydrogen peroxide solution to each cylinder. The addition of the catalyst to each cylinder should be done as nearly simultaneously as possible – using two assistants will help.
- Start timing.
- Foam will rise up the cylinders.
- Time how long each foam takes to rise to the top (or other marked point) of the cylinder.
- The foam from the first three cylinders will probably overflow considerably.
- Place a glowing spill in the foam; it will re-light, confirming that the gas produced is oxygen.
The lead dioxide will probably be fastest, followed by manganese dioxide and liver. Potato will be much slower and the iron oxide will barely produce any foam. This order could be affected by the surface areas of the powders.
Some students may believe that the catalysts – especially the oxides – are reactants because hydrogen peroxide is not noticeably decomposing at room temperature.
The teacher could point out the venting cap on the peroxide bottle as an indication of continuous slow decomposition.
Alternatively, s/he could heat a little hydrogen peroxide in a conical flask with a bung and delivery tube, collect the gas over water in a test-tube and test it with a glowing spill to confirm that it is oxygen.
This shows that no other reactant is needed to decompose hydrogen peroxide.
NB: Simply heating 50 volume hydrogen peroxide in a test-tube will not succeed in demonstrating that oxygen is produced. The steam produced will tend to put out a glowing spill. Collecting the gas over water has the effect of condensing the steam. It is also possible to ‘cheat’ by dusting a beaker with a tiny, almost imperceptible, amount of manganese dioxide prior to the demonstration and pouring hydrogen peroxide into it. Bubbles of oxygen will be formed in the beaker.
The reaction is :
2H 2 O 2 (aq) → 2H 2 O(l) + O 2 (g)
This is catalysed by a variety of transition metal compounds and also by peroxidase enzymes found in many living things.
- Repeat the experiment, but heat the liver and the potato pieces for about five minutes in boiling water before use.
- There will be almost no catalytic effect, confirming that the catalyst in these cases is an enzyme that is denatured by heat.
- Investigate the effect of using lumpy or powdered manganese dioxide.
- The powdered oxide will be more effective because of its greater surface area.
- Try using other metal oxides or iron filings as catalysts.
- Animal blood may be used instead of liver if local regulations allow this.
- One teacher suggested measuring the height of the foam over suitable time intervals and plotting a graph.
More resources
Add context and inspire your learners with our short career videos showing how chemistry is making a difference .
Hydrogen peroxide decomposition using different catalysts - teacher notes
Additional information.
This practical is part of our Classic Chemistry Demonstrations collection.
- 14-16 years
- 16-18 years
- Demonstrations
- Reactions and synthesis
- Rates of reaction
Specification
- Catalysts are substances that speed up chemical reactions but can be recovered chemically unchanged at the end of the reaction.
- (d) catalysts as substances that increase the rate of a reaction while remaining chemically unchanged and that they work by lowering the energy required for a collision to be successful (details of energy profiles are not required)
- (e) characteristics of a catalyst
- 2.3.2 suggest appropriate practical methods to measure the rate of a reaction and collect reliable data (methods limited to measuring a change in mass, gas volume or formation of a precipitate against time) for the reaction of: metals with dilute acid;…
- 2.3.2 suggest appropriate practical methods to measure the rate of a reaction and collect reliable data (methods limited to measuring a change in mass, gas volume or formation of a precipitate against time) for the reaction of: metals with dilute acid…
- Rate of reaction.
- (ii) catalysts.
- Enzymes as catalysts produced by living cells (two examples).
- WS.3.5 Interpreting observations and other data (presented in verbal, diagrammatic, graphical, symbolic or numerical form), including identifying patterns and trends, making inferences and drawing conclusions.
- Catalysts change the rate of chemical reactions but are not used up during the reaction. Different reactions need different catalysts.
- Enzymes act as catalysts in biological systems.
- Factors which affect the rates of chemical reactions include: the concentrations of reactants in solution, the pressure of reacting gases, the surface area of solid reactants, the temperature and the presence of catalysts.
- WS3.5 Interpreting observations and other data (presented in verbal, diagrammatic, graphical, symbolic or numerical form), including identifying patterns and trends, making inferences and drawing conclusions.
- Recall that enzymes act as catalysts in biological systems.
- Describe the characteristics of catalysts and their effect on rates of reaction.
- 3e Interpreting observations and other data (presented in verbal, diagrammatic, graphical, symbolic or numerical form), including identifying patterns and trends, making inferences and drawing conclusions
- 7.6 Describe a catalyst as a substance that speeds up the rate of a reaction without altering the products of the reaction, being itself unchanged chemically and in mass at the end of the reaction
- 7.8 Recall that enzymes are biological catalysts and that enzymes are used in the production of alcoholic drinks
- IaS2.11 in a given context interpret observations and other data (presented in diagrammatic, graphical, symbolic or numerical form) to make inferences and to draw reasoned conclusions, using appropriate scientific vocabulary and terminology to communicat…
- C6.2.4 describe the characteristics of catalysts and their effect on rates of reaction
- C6.2.5 identify catalysts in reactions
- C6.2.14 describe the use of enzymes as catalysts in biological systems and some industrial processes
- C6.2.13 describe the use of enzymes as catalysts in biological systems and some industrial processes
- WS.1.3e interpreting observations and other data
- C5.1f describe the characteristics of catalysts and their effect on rates of reaction
- C5.1i recall that enzymes act as catalysts in biological systems
- C5.2f describe the characteristics of catalysts and their effect on rates of reaction
- C5.2i recall that enzymes act as catalysts in biological systems
Related articles
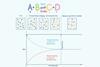
Help learners master equilibrium and reversible reactions
2024-06-24T06:59:00Z By Emma Owens
Use this poster, fact sheet and storyboard activity to ensure your 14–16 students understand dynamic equilibrium
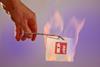
Non-burning paper: investigate the fire triangle and conditions for combustion
2024-06-10T05:00:00Z By Declan Fleming
Use this reworking of the classic non-burning £5 note demonstration to explore combustion with learners aged 11–16 years
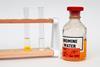
Everything you need to introduce alkenes
2024-06-04T08:22:00Z By Dan Beech
Help your 14–16 learners to master the fundamentals of the reactions of alkenes with these ideas and activities
No comments yet
Only registered users can comment on this article., more experiments.
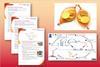
‘Gold’ coins on a microscale | 14–16 years
By Dorothy Warren and Sandrine Bouchelkia
Practical experiment where learners produce ‘gold’ coins by electroplating a copper coin with zinc, includes follow-up worksheet
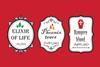
Practical potions microscale | 11–14 years
By Kirsty Patterson
Observe chemical changes in this microscale experiment with a spooky twist.
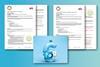
Antibacterial properties of the halogens | 14–18 years
By Kristy Turner
Use this practical to investigate how solutions of the halogens inhibit the growth of bacteria and which is most effective
- Contributors
- Email alerts
Site powered by Webvision Cloud
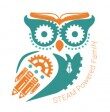
Elephant Toothpaste
Create a giant, foaming reaction that will astound kids with this Elephant Toothpaste Science Experiment. This simple science experiment is a another erupting science that thrills kids of all ages. Students will learn about chemical reactions that are exothermic. Go beyond baking soda and vinegar reactions and try Elephant Toothpaste.
How To Make Elephant Toothpaste
What you will discover in this article!

Disclaimer: This article may contain commission or affiliate links. As an Amazon Influencer I earn from qualifying purchases. Not seeing our videos? Turn off any adblockers to ensure our video feed can be seen. Or visit our YouTube channel to see if the video has been uploaded there. We are slowly uploading our archives. Thanks!
This classic science experiment has been wowing kids for generations. At the science centre or on TV you have probably seen spectacular elephant toothpaste experiments that shoot up feet into the air. Here we provide you with a simple version of Elephant Toothpaste using items from your home.
Check out our video set to music demonstrating Elephant Toothpaste in action!
Watch this fun experiment in action. If you can’t see the video, please turn off your adblockers as they also block our video feed. You can also find this video on the STEAM Powered Family YouTube Channel .
What is Elephant Toothpaste?
Elephant toothpaste is a safe, fantastic foamy science experiment using Hydrogen Peroxide and Yeast. When done in a beaker or bottle that narrows at the top, it oozes out like a great big tube of toothpaste. Perfectly sized for an elephant!
Depending on where you are in the world, Elephant’s Toothpaste has also been called Devil’s Toothpaste, Demon’s Toothpaste
It packs huge wow factor with the kids. It also provides a sensory experience while teaching kids important scientific principles such as:
Chemistry Catalyst Surface tension Exothermic reaction Temperature Liquid/Gas (states of matter) Mixture
Is Elephant Toothpaste Safe?
Elephant Toothpaste is safe when using 3% hydrogen peroxide! The foam is composed of soap, oxygen and water. It makes a great sensory science experience.
NOTE: Although we call this toothpaste, it is not toothpaste and should not be tasted. Ensure appropriate supervision is provided at all times.
Elephant Toothpaste Ingredients
250mL Glass Erlenmeyer Flask or an empty plastic bottle Dry yeast Warm water Liquid dish soap 3% hydrogen peroxide * Liquid food colouring Measuring cups Measuring spoons Safety goggles or glasses Large tub or tray (something with a lip) Funnel (optional – can help kids with pouring) Infrared non-contact thermometer (optional, helps with measuring the exothermic reaction)
*Higher percentage concentration hydrogen peroxide are dangerous and can cause skin irritation. Ensure you are using 3% with your kids in this experiment.
Elephant Toothpaste Experiment
Pick a place to do this experiment that is easy to clean up. If you do this in a nice big, high lipped tray that should contain most of foam, but in my experience kids love to play and touch the foam, so things can get messy quickly. Have your child put on safety glasses and clothes they don’t mind splashing.
Start by mixing one packet (about 1 tablespoon) of yeast with 1/4 cup warm water in cup to create the first mixture . Let it sit for about 30 seconds while you prep the beaker.
Into the flask add 150 mL of Hydrogen Peroxide, a tablespoon of dish soap (after a few tries we just used a squirt of dish soap, it doesn’t need to be exact), and about 15 drops of food coloring (if desired).
If you are taking temperatures, take a temperature of each of the liquids now.
Now pour the yeast mixture into the hydrogen peroxide solution.
Watch the reaction!
If you are doing temperatures, make sure you take a temperature read during the reaction too!
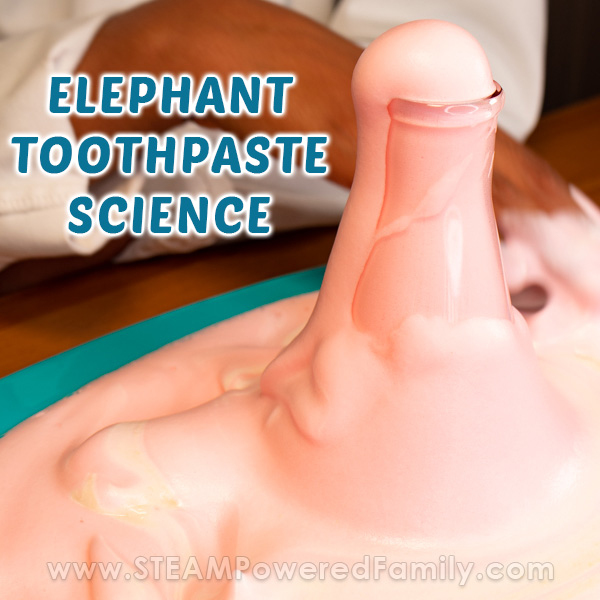
Elephant Toothpaste Science
The main reactor in this experiment is 3% Hydrogen Peroxide a liquid made from hydrogen atoms and oxygen atoms (chemical formula H 2 O 2 ), which is used in first aid as an antiseptic. Normally it comes in a dark bottle because H 2 O 2 or hydrogen peroxide breaks down in light, turning into oxygen (O 2 ) and water (H 2 O). Normally this reaction happens very slowly, but we can speed it up with a little science!
Yeast, which we use to make bread , is an organism that contains a special chemical called catalase that can act as a catalyst to help break down hydrogen peroxide. This means that if you mix yeast with hydrogen peroxide, the hydrogen peroxide will rapidly break down into liquid water and oxygen gas . During this process energy is also released in the form of heat, which is known as an exothermic reaction .
Elephant Toothpaste Chemical Formula
Here is the chemical reaction for Elephant Toothpaste: 2 H 2 O 2 (aq) → 2 H 2 O (l) + O 2 (g)
Those gas bubbles would normally pop and disappear, but by adding a bit of dish soap, we can trap lots of bubbles thanks to an increase in surface tension . We explore this scientific concept in our Magic Milk experiment . This means the foam is simply soap, oxygen and water. Perfectly safe to touch and explore!
The result is a spectacular eruption of foam known as Elephant Toothpaste.
Elephant Toothpaste Extension Activities
There are a few things you can do to dig deeper into this reaction:
- Use an infrared no touch thermometer to measure the exothermic reaction.
- Why stop at a single color? Make multi-colored Elephant’s Toothpaste by dropping different colors of liquid food coloring down the inside of the bottle rather than mixing it into the solution. The result will be colored foam stripes.
- Try different shaped containers to see how the reaction changes. Maybe a plastic soda bottle or a mason jar with a wider neck. How does the experiment results change?
- Alter the ratio of yeast to hydrogen peroxide. What if you increase to one cup of hydrogen peroxide? What effect does it have on the reaction?
- What happens if you remove the dish soap?
- Visit your local science center and ask when they will be doing their next Elephant Toothpaste demonstration. They use a different reaction that produces spectacular results with lots of foam!
- Only do this next extension with responsible middle or high school students, and under direct adult supervision – Visit the hair supply store and get 6% or higher hydrogen peroxide. How does the higher concentration hydrogen peroxide change the results? ( Hint … bigger and messier!)
Don’t forget to try our Valentine’s Day Science Experiment Blooming Roses Elephant Toothpaste !
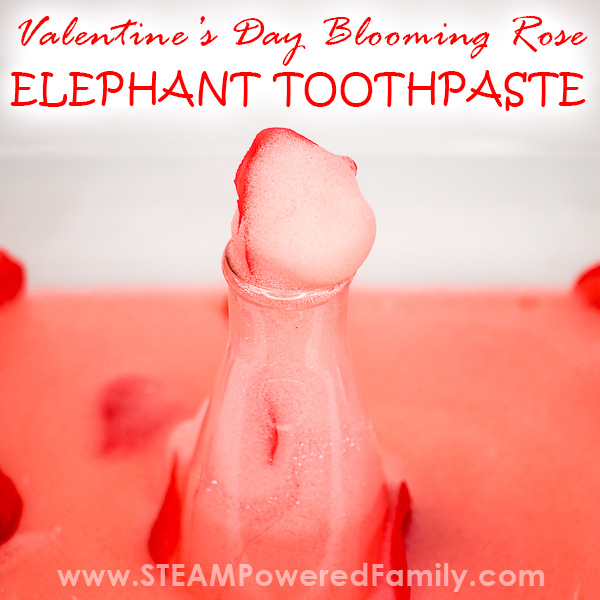
How To Clean Up Elephant Toothpaste
Once you are done with the experiment and playing with the foam, you can wash everything up in the sink . In fact, it cleans up easily thanks to the soap.
Sensory Experience
While doing this experiment ensure you discuss the sensory inputs provided by this fantastic foaming science.
First they will experience the sense of smell from the yeast. Have the kids discuss this smell and what it reminds them of.
Next we have the sensory experience of touch . This is done in two ways. Have them touch the sides of the beaker before and after the experiment starts. This way they can experience the exothermic reaction (the beaker will feel warm, but as long as you are using 3% hydrogen peroxide, it is not hot). You can reinforce this exploration by using a non-contact thermometer. The second touch experience is with the foam. The foam is perfectly safe to touch. As we explored in the science portion, the foam is just soap, water and oxygen.
Elephant toothpaste is a fantastic sensory science experience.

Elephant Toothpaste Classic Science
Creating Elephant Toothpaste is a quick and easy science experiment that can be easily adjusted to suit the learning needs of upper elementary through middle school. It has great WOW factor, and kids around the world have loved creating these foamy fountains we affectionately call, Elephant Toothpaste.
Enjoy this classic science experiment!
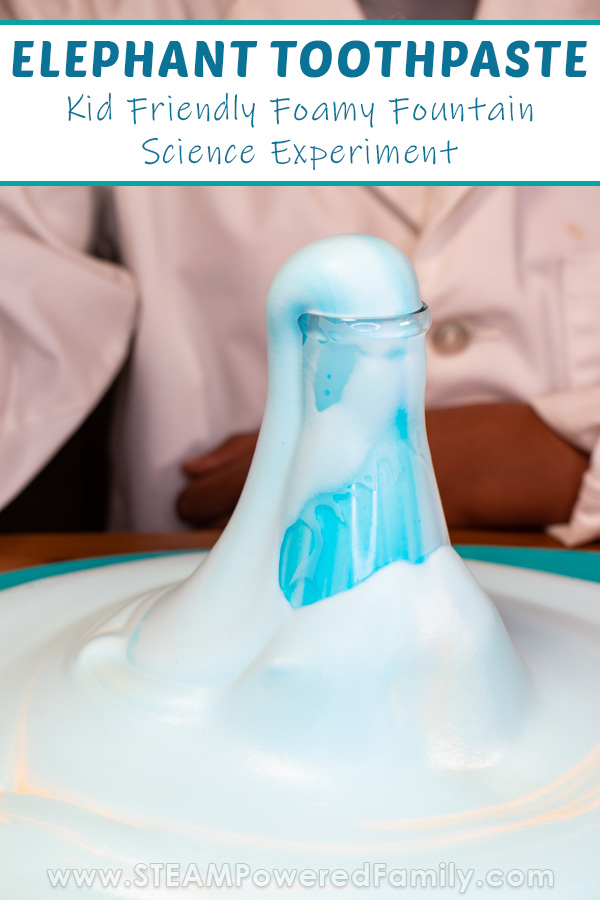
More Science Experiments For Kids

5 Days of Smart STEM Ideas for Kids
Get started in STEM with easy, engaging activities.
- Experiments
- Secret Science of Stuff
- Science ABCs
- Warm Up to Some Cool Reactions
- You are here:
- American Chemical Society
- Adventures in Chemistry
Some chemical reactions change temperature, change color, produce a gas, or make a solid from two liquids. Try this reaction and see what it does!
Here's what to do:
- Pour 1 tablespoon of hydrogen peroxide into a cup. Place the thermometer into the cup.
- Hold the thermometer and the cup so they do not fall over. Read the temperature and write it down as your “Starting Temperature”.
- Measure 1 teaspoon of yeast. While the thermometer is still in the cup, dump all the yeast into the cup. Gently swirl the cup while you look at the temperature.
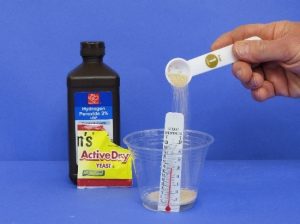
What did you observe?
What to expect
The yeast and hydrogen peroxide will produce bubbles and the temperature will increase.
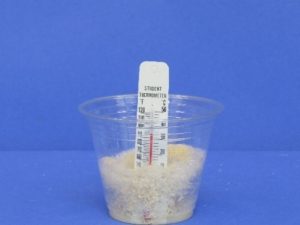
What's happening in there?
When yeast was added to hydrogen peroxide, a chemical in the yeast causes a reaction in which the hydrogen peroxide breaks apart to form oxygen gas and water. The oxygen was in the bubbles you saw. This reaction causes the temperature to go up.
Choose an Account to Log In

Notifications
Science project, hydrogen peroxide and yeast.
Chemistry produces some of the most amazing compounds. You’ve probably used vinegar and baking soda to make a volcano. You’ve definitely made your own foam with soap and water.
Foam is usually created by chemical reactions occurring between two or more liquids. And when you put the right two solutions together, you don’t just get foam—you get MEGA-foam!
This mega-foam is made with hydrogen peroxide and yeast. But not all mega-foams are created equal! Some will be more “mega” than others, depending on the kind of peroxide you use.
Which peroxide will make the biggest Mega Foam?
- 2 soda bottles
- 2 cookie sheets
- 4 tablespoons warm water
- 2 teaspoon yeast
- 1/2 cup hydrogen peroxide 3%
- 1/2 cup hydrogen peroxide 6%
- 8 drops of food coloring of choice
- liquid dishwashing soap
- 2 small dishes
- Label soda bottles “6” and “3.” Bottle 6 is for the 6% hydrogen peroxide and bottle 3 is for the 3% hydrogen peroxide.
- Add ½ cup of the appropriate type of hydrogen peroxide to each empty bottle.
- Add a squirt of dishwashing soap to each bottle.
- Add 4 drops of food coloring to each.
- In 2 small dishes, add to each 1 teaspoon of yeast to 2 tablespoons of warm water.
- Stir to dissolve.
- Add a yeast mixture to each peroxide mixture.
- Stand back and watch to see which peroxide produced the biggest Mega Foam!
- Record what you saw happen.
The 6% hydrogen peroxide solution should have given you the biggest Mega Foam, but you still should have gotten some impressive results with the 3% solution, too.
Hydrogen peroxide is breaks down into water and oxygen. That’s why it’s stored in dark containers: to slow this process down. Adding the yeast to the hydrogen peroxide helps this breaking-down process occur much faster. As the process progresses, the dishwashing soap catches the oxygen that is released by the peroxide. The final result is tons of bubbles.
Digging Deeper
How can you take this experiment further? Find different ways to speed up the reaction. Try using different dish washing detergents. What if you changed the temperature of the solutions before mixing them? There are hundreds of ways to get some cool insights from this colossal foam. Just remember: change only thing at a time, and record your results!
Related learning resources
Add to collection, create new collection, new collection, new collection>, sign up to start collecting.
Bookmark this to easily find it later. Then send your curated collection to your children, or put together your own custom lesson plan.

- Science Notes Posts
- Contact Science Notes
- Todd Helmenstine Biography
- Anne Helmenstine Biography
- Free Printable Periodic Tables (PDF and PNG)
- Periodic Table Wallpapers
- Interactive Periodic Table
- Periodic Table Posters
- Science Experiments for Kids
- How to Grow Crystals
- Chemistry Projects
- Fire and Flames Projects
- Holiday Science
- Chemistry Problems With Answers
- Physics Problems
- Unit Conversion Example Problems
- Chemistry Worksheets
- Biology Worksheets
- Periodic Table Worksheets
- Physical Science Worksheets
- Science Lab Worksheets
- My Amazon Books
5 Ways to Make a Volcano

There is more than one way to make a chemical volcano. Actually, there are several methods. Here are some of the best, from the tried-and-true baking soda and vinegar volcano to the most exotic dry ice volcano.
Make the Volcano Cone
You can use a bottle or can or really any container for your volcanic eruption, but it’s easy to make the volcano shape by coating your container with clay or papier mache. Here is a simple recipe for a homemade clay volcano:
- 6 cups flour
- 2 cups salt
- 2 cups water
- 4 tablespoons cooking oil
- Mix the ingredients together in a large bowl. It’s easiest if you stir the flour, salt, and oil together first and then mix in the water. You can add more water if needed. You want a firm, smooth dough.
- Stand an empty soda bottle or can in a pie tin or baking pan (so your ‘lava’ won’t make a mess) and mold the dough into a volcano shape. Be sure you don’t drop dough into the bottle or cover the opening.
- If you want to paint the volcano, wait until the dough is dry.
Now for the recipes! Most use common ingredients that you have at home.
Baking Soda and Vinegar Volcano
This is the classic science fair project volcano. The baking soda (sodium bicarbonate) reacts with the vinegar (weak acetic acid) to produce carbon dioxide gas. The detergent traps the gas, which is heavier than air, so it flows down the side of the volcano.
- liquid dishwashing detergent
- red or orange food coloring
- baking soda
- Pour warm water into the volcano until it is 1/2 to 3/4 of the way full.
- Add several drops of food coloring.
- Add a squirt of detergent. This helps the ‘lava’ foam up and flow.
- Add a couple of spoonfuls of baking soda.
- When you are ready to start the eruption, pour vinegar into your volcano.
- You can recharge the volcano with more baking soda and vinegar.
Note: If you don’t have vinegar, you can use another acidic liquid, like lemon juice or orange juice.
Yeast and Peroxide Volcano
- packet of quick-rise yeast
- hydrogen peroxide (3% sold in stores or can use 6% from beauty supply stores)
- food coloring
- Pour the hydrogen peroxide solution into the volcano until it is nearly full. The 3% household peroxide is safe to handle, but wear gloves and use extreme caution if you use the 6% peroxide, which can give you chemical burns!
- Add several drops of food coloring for your lava.
- When you are ready for the eruption, add the packet of yeast to the volcano.
Ketchup and Vinegar Volcano

This volcano bubbles and oozes lava. The eruption is not so dramatic, but is interesting and long-lasting. The acidity of the vinegar and tomatoes in the ketchup reacts with the baking soda to produce carbon dioxide gas, which gets trapped as bubbles by the detergent.
- dishwashing liquid
- Mix together ketchup, warm water, and a squirt of detergent to make lava.
- Pour the mixture into the volcano so it is nearly full.
- When you are ready for the eruption, add baking soda.
Mentos and Diet Soda Volcano

This volcano erupts instantly and spectacularly. For a truly memorable volcano, use diet tonic water instead of diet cola and shine a black light on the volcano. This produces a vivid blue glowing eruption!
- diet soda (regular soda works too, but produces a sticky mess)
- Mentos candies
- Fill the volcano full of soda (or you could have molded the volcano around a full soda bottle.
- When you are ready for the eruption, drop all of the Mentos candies into the mouth of the bottle at once. One easy way to do this is to roll a sheet of paper around the candies, put your finger beneath them to hold them in place, and release the candies over the hole. Be prepared for a major splash!
Dry Ice Volcano
This volcano appears to smoke, releasing a cascade of bubble lava.
- Fill the volcano with warm water.
- Add a bit of dishwashing liquid.
- When you are ready to start the eruption, use gloves or tongs to drop a piece of dry ice into the volcano.
Do you need more ways to make a volcano ? You can bake a souffle to model the geological processes or make a realistic wax volcano .
Related Posts
Science Bob
- Experiments
- Science Fair Ideas
- Science Q&A
- Research Help
- Experiment Blog
Crazy Foam Experiment
Fun with foam.
Foam is fun! Check out this video to see our Fantastic Foamy Fountain in action. The experiment uses Hydrogen peroxide and dry yeast. Hydrogen peroxide is similar to water but it has an extra oxygen atom. This makes it more dangerous and only adults should handle the hydrogen peroxide.
When you add the yeast, it acts as a catalyst (a helper) to release the extra oxygen gas and the soap helps to create all the foam. Try it out yourself!
The second experiment with the giant foam eruption at the end used a more powerful hyrogen peroxide and a different chemical for a catalyst. The reaction happens very fast and gives off quite a bit of heat. It’s pure foam fun! I mean pure science.
Click here for experiment instructions.
ADS (these ads support our free website)
Share this page.
August 1, 2019
Make Elephant Toothpaste
A bubbly science project from Science Buddies
By Science Buddies & Ben Finio
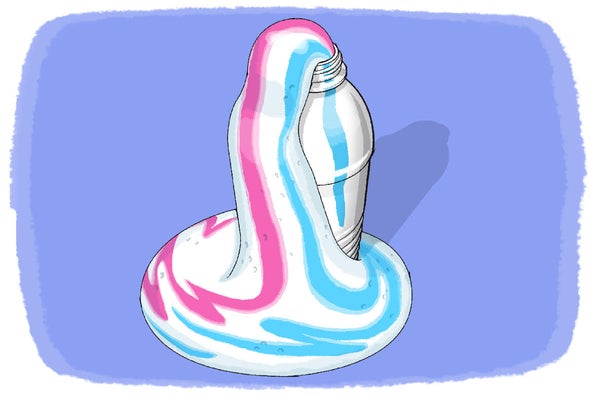
Squeeze some science: Use a little kitchen chemistry to make a fountain of "toothpaste" almost big enough for an elephant's brush!
George Retseck
Key Concepts Chemistry Biology Reaction Catalyst Surface tension
Introduction Create a giant foaming reaction, and use science to wow your friends with this classic activity. With just a few ingredients you can make something that looks like foamy toothpaste being squeezed from a tube—but so big that it looks almost fit for an elephant!
Background You might be familiar with hydrogen peroxide as an antiseptic used to clean cuts and scrapes, which it does by killing bacteria. But what is it? It is a liquid made from hydrogen atoms and oxygen atoms (its chemical formula is H 2 O 2 ). It is available in different strengths, or concentrations. You usually find it in a 3 percent concentration (although higher concentrations are available, they are more dangerous and must be handled carefully). It also breaks down when exposed to light, which is why it usually comes in dark brown bottles.
On supporting science journalism
If you're enjoying this article, consider supporting our award-winning journalism by subscribing . By purchasing a subscription you are helping to ensure the future of impactful stories about the discoveries and ideas shaping our world today.
When hydrogen peroxide breaks down, it turns into oxygen (O 2 ) and water (H 2 O). Normally this breakdown happens very slowly. But you can make that reaction happen faster! How? By adding a catalyst. Yeast is an organism that contains a special chemical called catalase that can act as a catalyst to help break down hydrogen peroxide. Catalase is present in almost all living things that are exposed to oxygen, and it helps them break down naturally occurring hydrogen peroxide.
This means that if you mix yeast with hydrogen peroxide, the hydrogen peroxide will rapidly break down into water and oxygen gas. The oxygen gas forms bubbles. These bubbles would usually escape from the liquid and pop quickly. But adding a little dish soap provides additional surface tension, allowing the bubbles to get trapped and creating lots of foam. This foam looks like a giant squeeze of toothpaste—almost big enough for an elephant!
Empty plastic bottle
Dry yeast (found in the baking section of the grocery store)
Liquid dish soap
3% hydrogen peroxide
Measuring cups
Measuring spoons
Safety glasses
Large tub or tray to catch the foam
Location for the activity that can tolerate spills (of hydrogen peroxide as well as possibly food coloring), such as a kitchen or bathroom—or an outdoor location
Liquid food coloring (optional)
Different-shaped bottles or glasses (optional)
Preparation
Put on your safety glasses to do this activity because hydrogen peroxide can irritate your eyes. (Note: although the product of this activity resembles toothpaste, it is not toothpaste, so do not attempt to use it!)
Gather your materials in the location where you plan to do your activity. Place your plastic bottle on the tray or tub so that it is easy to clean up all the foam.
Measure 1/2 cup of hydrogen peroxide, and carefully pour it into the bottle.
Add a big squirt of dish soap into the bottle, and swirl gently to mix.
If you want to make your foam a single color, add a few drops of food coloring directly into the hydrogen peroxide, and swirl the bottle gently to mix. If you want to give your foam stripes like some toothpastes, put the drops along the inside rim of the bottle’s mouth. Let them drip down the inside of the bottle, but do not mix.
In a measuring cup mix together one tablespoon of yeast and three tablespoons of warm water. Stir for about 30 seconds.
Pour the yeast mixture into the bottle then quickly step back, and watch your reaction go! What happens? How long does the reaction last?
Extra: Try the activity without the dish soap. What happens? How was the result different?
Extra: Try the activity with different-shaped containers. What happens if you use a bottle with a narrower or wider neck—or a cylindrical drinking glass with no neck?
Observations and results You probably saw lots of bubbles and foam in this activity. What makes the foam appear? When the hydrogen peroxide comes into contact with the yeast it starts breaking down into water and oxygen. Oxygen is a gas and therefore wants to escape the liquid. The dish soap that you added to your reaction, however, traps these gas bubbles, forming a foam. The reaction continues as long as there is some hydrogen peroxide and yeast left. Once one of them runs out it stops making new foam. If you tried the activity without dish soap, the reaction probably will still made bubbles—but not foam.
Cleanup Wash the foam down the sink when you are done with the activity.
More to Explore Enzymes, Foam and Hydrogen Peroxide , from Science Buddies Exploring Enzymes , from Scientific American The Liver: Helping Enzymes Help You , from Scientific American Erupting Diet Coke with Mentos , from Science Buddies Yeast Alive! Watch Yeast Live and Breathe , from Scientific American STEM Activities for Kids , from Science Buddies
This activity brought to you in partnership with Science Buddies
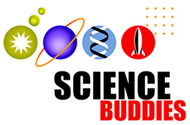
Cool Science Experiments Headquarters
Making Science Fun, Easy to Teach and Exciting to Learn!
Science Experiments
Elephant Toothpaste Science Experiment
Elephant Toothpaste anyone? Although elephants might not be too excited about the idea, your kids will be thrilled with the fun chemical reaction of this super simple science experiment.
With only a few common items that you probably already have at home, kids can see the quick and impressive results of the chemical reaction, feel the heat released from the process, and even play with the “elephant toothpaste” foam afterward.
Below you’ll find detailed and printable instructions, a materials list, a demonstration video, and an easy to understand explanation of why it works!
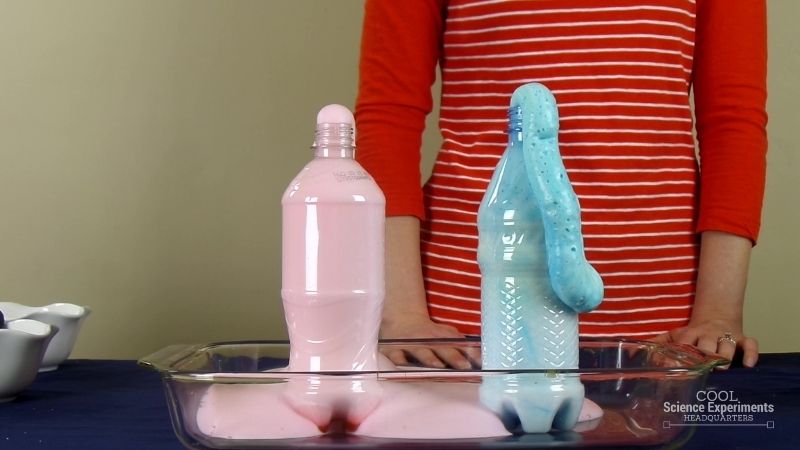
JUMP TO SECTION: Instructions | Video Tutorial | How it Works

Supplies Needed
- 2 Tablespoons Warm Water
- 1 Teaspoon Yeast
- Food Coloring
- Hydrogen Peroxide – Either 3% or 6%
- Empty Plastic Bottle
Helpful Tip: You can find the 3% at most stores, but you will need to either go to a Beauty Supply Store or order the 6% online. In the video I perform the experiment two ways. Once using the 3% peroxide and once using the 6%. It is often recommended to use 6% peroxide, but we actually preferred the reaction that happened with the 3% better.
Elephant Toothpaste Science Lab Kit – Only $5
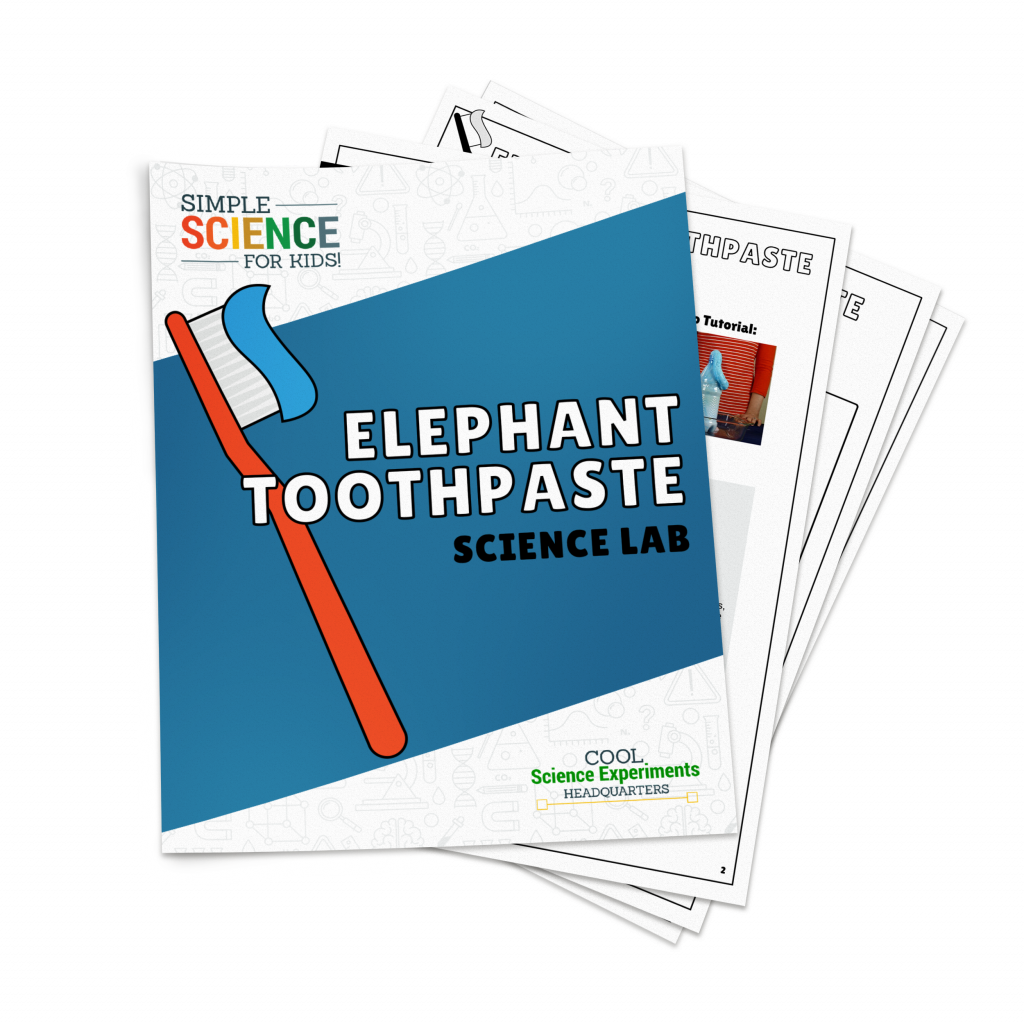
Use our easy Elephant Toothpaste Science Lab Kit to grab your students’ attention without the stress of planning!
It’s everything you need to make science easy for teachers and fun for students — using inexpensive materials you probably already have in your storage closet!
Elephant Toothpaste Experiment Instructions
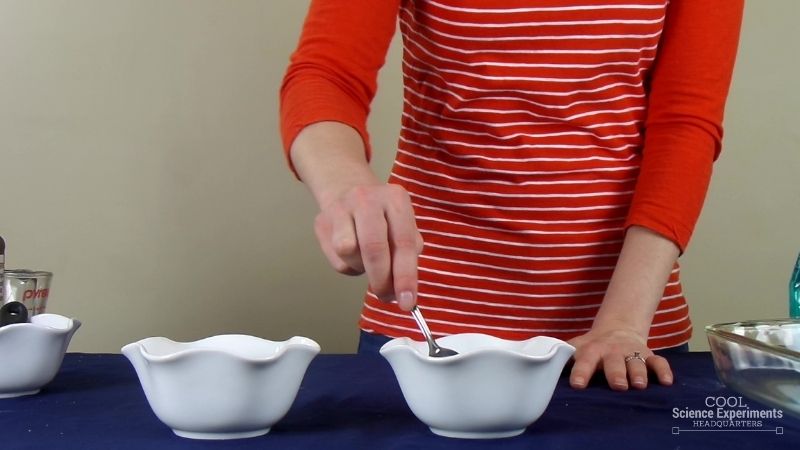
Step 1 – Combine two tablespoons of warm water with one teaspoon of yeast and mix until the yeast is completely dissolved in the water.
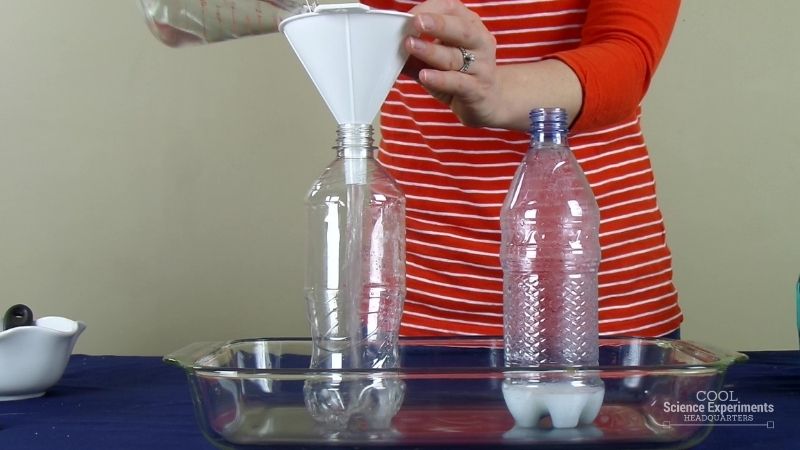
Step 2 – Pour 1/2 cup hydrogen peroxide into the empty bottle. You’ll see here that we have two bottles. The bottle pictured on the left is being filled with 3% hydrogen peroxide while the bottle pictured on the right is filled with 6% hydrogen peroxide.
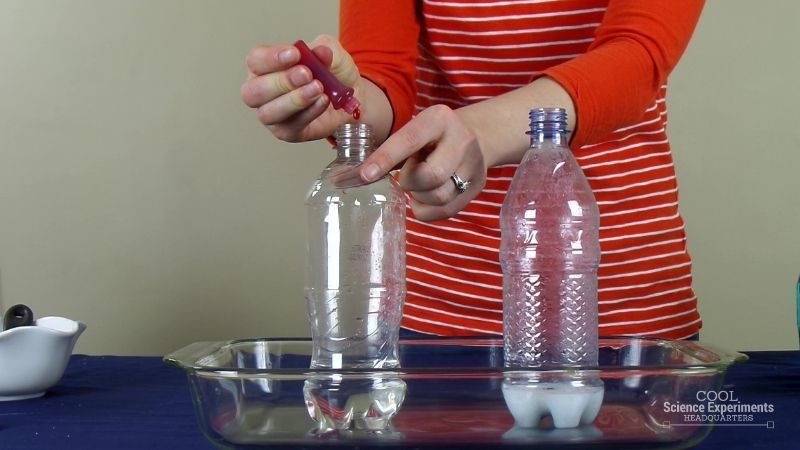
Step 3 – Add a few drops of food coloring into the bottle. Take a moment to make a few observations. What happened when you added the drops of food coloring?
Note: This step is optional, but adding the color adds a fun element to the experiment. You’ll see that we used a different color of food coloring for each bottle.
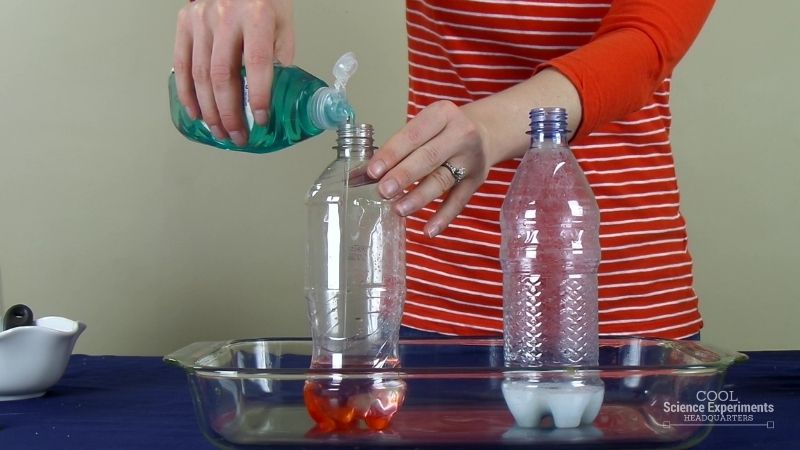
Step 4 – Next, add a squirt of dish soap into the bottle. Again, take a moment observe what happened. Did adding the dish soap appear to have any effect on the liquid already in the bottle?
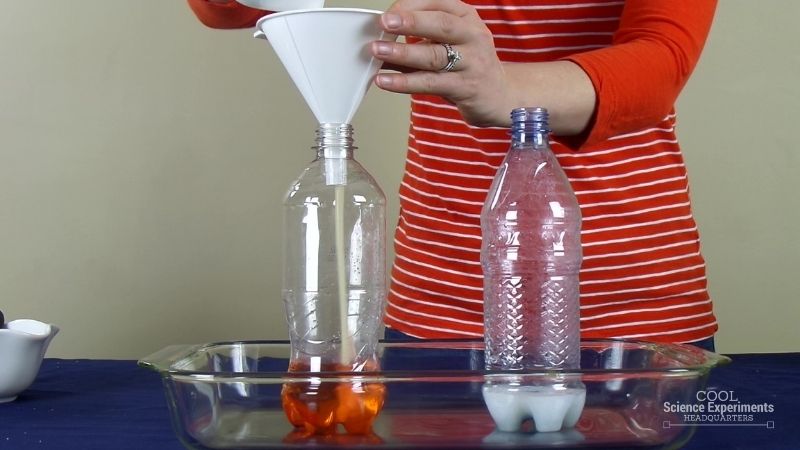
Step 5 – Finally, pour the mixture of water and yeast into the bottle. Watch carefully and observe what happens.
Helpful Tip: You may need to gently swirl the bottle in order to get the items to completely combine. We had to do that for the 6% hydrogen peroxide.
Sit back and watch what happens. Write down your observations as you watch the experiment. Can you describe what happens when you add the water and yeast mixture to the bottle. If you are using both the 3% and the 6% hydrogen peroxide like we are, is there a difference between what happens?
Wondering what caused the liquid to react that way? Find out the answer in the how does this experiment work section below.
Video Tutorial
Watch the Elephant Toothpaste Science Experiment Step by Step Instructions Video
How Does the Elephant Toothpaste Science Experiment Work
This experiment shows a very impressive and fast chemical reaction! Hydrogen peroxide is a combination of hydrogen and oxygen (H2O2). In this experiment, yeast is a catalyst that helps release oxygen molecules from the hydrogen peroxide solution.
A catalyst is a material that increases the rate of a reaction without itself being consumed
The release of oxygen molecules in this experiment happens FAST! The foam created in this chemical reaction is made up of very tiny oxygen bubbles. These bubbles result when hydrogen peroxide (H2O2) is broken down by the yeast into water (H2O) and oxygen (O2).
During the reaction, feel the bottle. It will feel warm to the touch because it is an exothermic reaction .
An exothermic reaction is a chemical reaction that releases energy through light or heat (in this case, heat).
The reaction will continue and foam will be produced until all of the hydrogen peroxide (H2O2) has been broken down into water (H2O) and oxygen (O2).
**Have fun playing with the foam after the experiment is over! It is safe to touch because all that foam is just soap, water and oxygen. Let your kids have some fun with the foam too. Just be sure to have a towel handy!
Other Ideas to Try
Try adding more or less yeast when doing the experiment again. How does this affect the amount of foam produced?
Try the experiment again, but change the size of the bottle. How does this affect the amount of foam produced?
I hope you enjoyed the experiment. Here are some printable instructions:
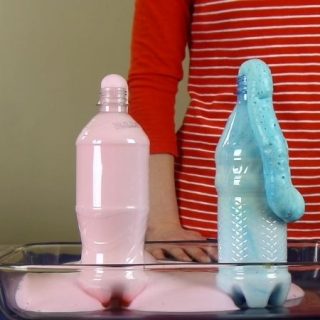
- Hydrogen Peroxide – Either 3% or 6%
Instructions
- Combine two tablespoons of warm water with one teaspoon of yeast and mix until the yeast is completely dissolved in the water.
- Pour 1/2 cup hydrogen peroxide into the empty bottle
- Add a few drops of food coloring into the bottle
- Add a squirt of dish soap into the bottle
- Pour the mixture of water & yeast into the bottle
- Sit back and watch what happens
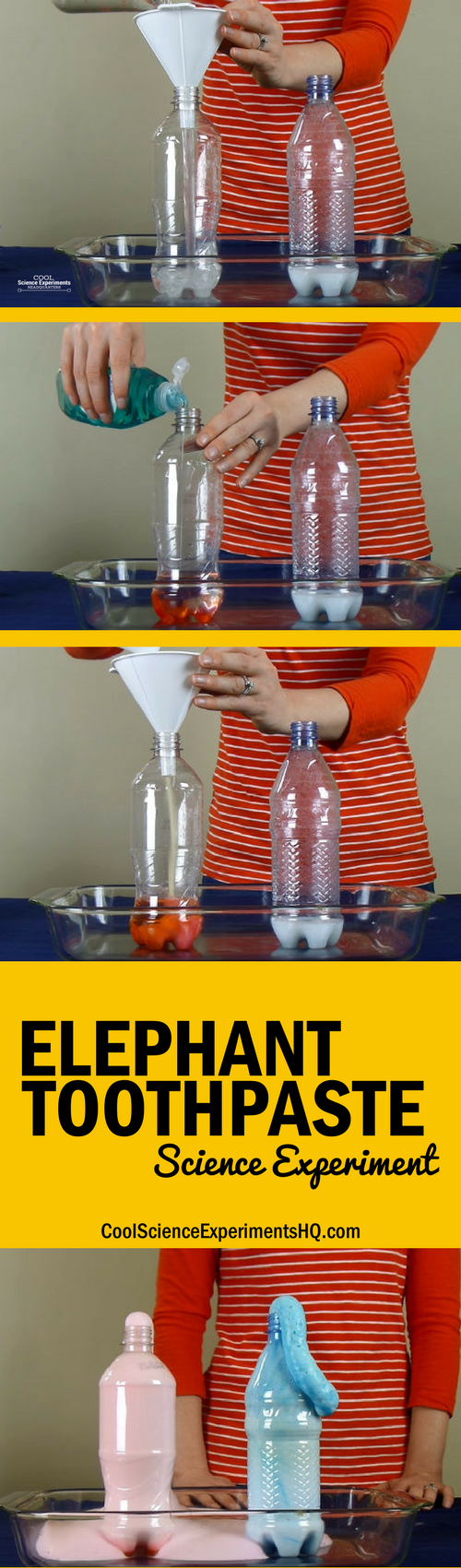
Reader Interactions
February 7, 2016 at 8:13 pm
do it was a cemical reacin
February 8, 2016 at 1:15 pm
Correct! The yeast is mixed with the hydrogen peroxide causes a chemical reaction.
Which version did you like better, the one that reacted fast or the one that reacted slow?
May 17, 2018 at 12:31 pm
Looks amazing! What is the chemical reaction?
March 24, 2019 at 10:40 am
I’m am going to do that science project and this was super helpful thanks so much
March 24, 2019 at 10:46 am
Thank you so much I am doing this science project and I don’t know what to do and now I know what to do j
September 1, 2019 at 8:09 am
Did you try using more yeast or more 3 % hydrogen peroxide?
Found this explanation: “The yeast acted as a catalyst; a catalyst is used to speed up a reaction. It quickly broke apart the oxygen from the hydrogen peroxide. Because it did this very fast, it created lots and lots of bubbles. Did you notice the bottle got warm. Your experiment created a reaction called an Exothermic Reaction – that means it not only created foam, it created heat! The foam produced is just water, soap, and oxygen ” The yeast is not reacting with the hydrogen peroxide. Rather it is acting as a catalyst. A catalyst is a substance that causes a chemical reaction to speed up but is not “used” up during the reaction. Hydrogen Peroxide: H2O2 hydrogen and oxygen Baker’s Yeast: C19H14O2 carbon, hydrogen and oxygen
August 18, 2020 at 2:25 pm
My son did this experiment which was great! The only problem was that he played with the foam afterwards and his hands got super itchy. Can you be allergic to the yeast? Just a warning in case this happens to anyone else.
August 11, 2023 at 8:49 pm
Yeah I did this with potassium iodide and 25% hydrogen peroxide in a lab and my fingers went a little white on the tips (I had gloves on after this happened) they did get itchy and I think it was just the hydrogen peroxide bleaching the skin. This is an exothermic reaction so it can get hot not sure how hot with yeast.
January 25, 2022 at 11:25 am
It did not work for me and i don’t know why maybe caused i used rubbing alcohol insted
December 6, 2023 at 3:55 pm
good helpful and fun
Leave a Reply Cancel reply
Your email address will not be published. Required fields are marked *
Save my name, email, and website in this browser for the next time I comment.
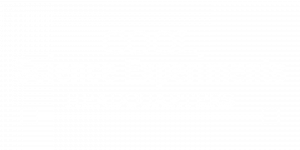
- Privacy Policy
- Disclosure Policy
Copyright © 2024 · Cool Science Experiments HQ
- Earth Science
- Physics & Engineering
- Science Kits
- Microscopes
- Science Curriculum and Kits
- About Home Science Tools
Science Projects > Chemistry Projects > Foaming Experiment | Elephant Toothpaste Science Project
Foaming Experiment | Elephant Toothpaste Science Project
Make a dramatic demonstration of a chemical reaction with this spinoff of the classic elephant toothpaste experiment.
This version catalyzes a chemical decomposition using only common household items, making it safer for home labs and young scientists. While the chemicals used may be ordinary, the results are exceptional!
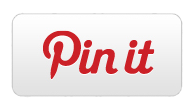
What You Need:
- Baking dish or pie plate
- Food coloring
- Erlenmeyer flask
- Graduated cylinder
- Hydrogen peroxide 30% (6% or higher)
- PPE (personal protective equipment): gloves , lab apron , and chemistry goggles
What You Do:
1. Safety first! Before beginning the experiment put on your PPE.
2. Use the graduated cylinder to measure 50 ml hydrogen peroxide, and pour it into the flask. Add a few drops of food coloring if you like.
3. Place the flask in the middle of a pie tin or baking dish.
4. Measure 10 ml dishwashing soap, and add it to the flask.
5. Open the packet of yeast, and pour its contents through the funnel into the flask. Quickly remove the funnel.
6. Feel the outside of the flask, and note its temperature. Wait a few minutes to see foam come streaming out of the flask.
7. Dispose of the remaining mixture by pouring it down the sink and rinsing with hot water.
What Happened:
Hydrogen peroxide’s chemical formula is H 2 O 2 . H 2 O is liquid water, and O 2 is oxygen gas. In this experiment, we observed the chemical decomposition of hydrogen peroxide. Chemical decomposition is the separation of a chemical compound into elements or simpler compounds. In this case, hydrogen peroxide decomposed into water and oxygen. The chemical expression for what happened is: 2 H 2 O 2 → 2 H 2 O + O 2 .
Adding the yeast sped up the process. Enzymes in the yeast called catalase acted as a catalyst —a substance added to a chemical mixture that speeds up the chemical reaction time. The yeast wasn’t necessary to make foam — only water, soap, and a whole lot of oxygen gas was necessary. But adding the yeast made the hydrogen peroxide decompose (break down) much more quickly than it would normally, releasing oxygen and water faster. The dish soap made the production of oxygen more noticeable because of the foam. This reaction also created heat, so it’s what’s known as an exothermic reaction , a reaction that releases heat energy.
Another interesting fact about hydrogen peroxide: it is sometimes used as rocket fuel. When hydrogen peroxide breaks down rapidly, it produces a lot of oxygen gas, which propels the rocket into the air. The hydrogen peroxide 30% HST sells is a solution of water and hydrogen peroxide, as is the kind most of us keep in our medicine cabinets—30% and 3% respectively. Hydrogen peroxide that powers rockets is at least 90% concentration.
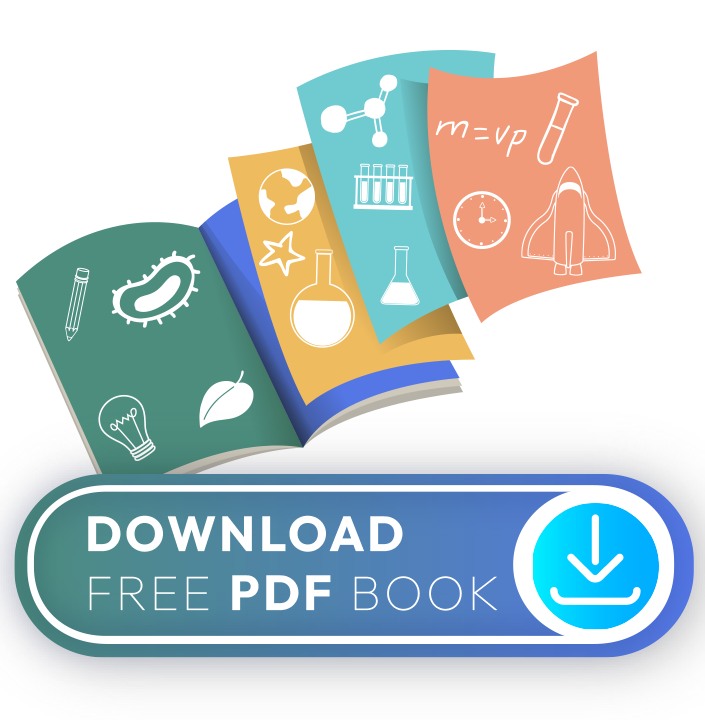
Teaching Homeschool
Welcome! After you finish this article, we invite you to read other articles to assist you in teaching science at home on the Resource Center, which consists of hundreds of free science articles!
Shop for Science Supplies!
Home Science Tools offers a wide variety of science products and kits. Find affordable beakers, dissection supplies, chemicals, microscopes, and everything else you need to teach science for all ages!
Related Articles
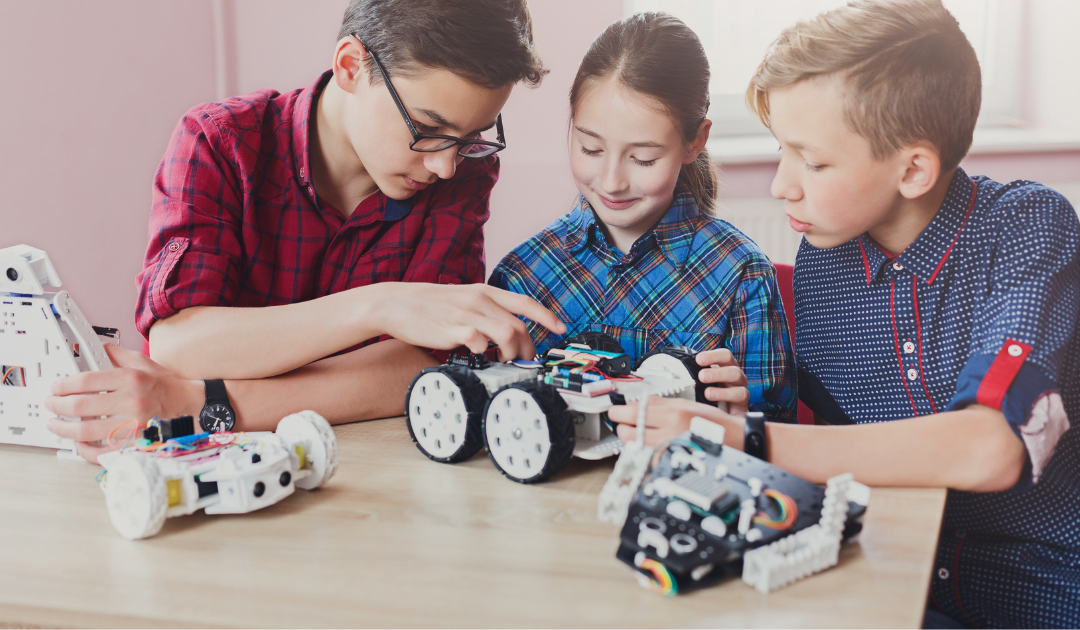
Engaging STEM Activities for Teens
Engaging STEM Activities for Teens Teens can explore many different concepts through fun and interactive activities. STEM lessons for teens can include experimenting, engineering, and more. If you have STEM toys for ten to twelve-year-olds, but your child is starting...
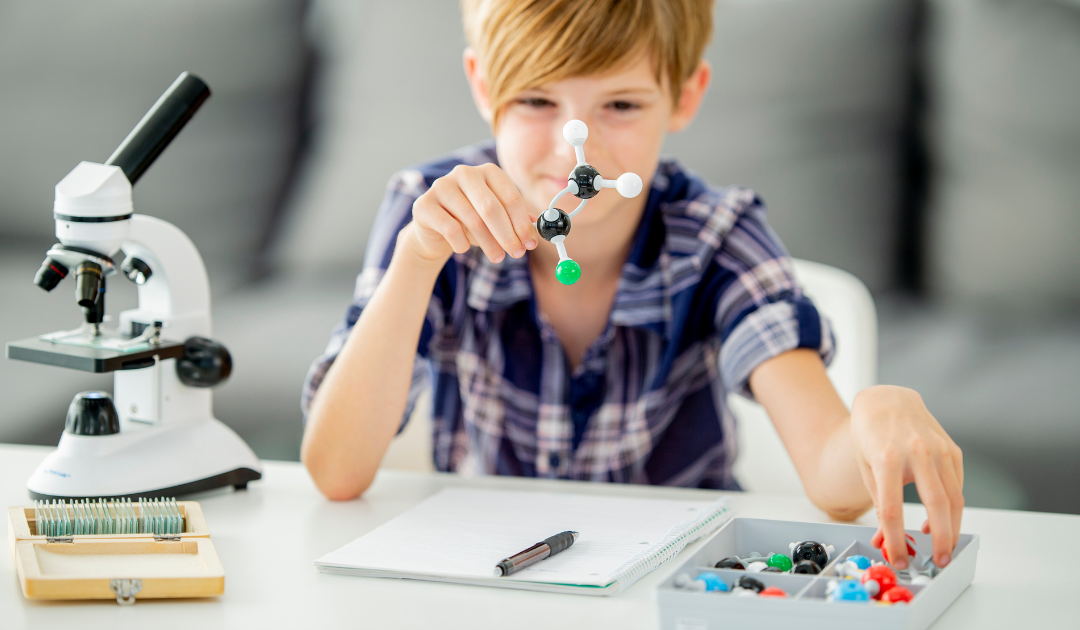
Fun STEM Activities for Third Graders
Fun STEM Activities for Third Graders STEM education is essential for young learners. The benefits go far beyond the traditional science fields. It can inspire children to pursue other interests and turn them into confident problem solvers. Some hands-on STEM...

Hands-On STEM Activities for Second Graders
Hands-On STEM Activities for Second Graders Hands-on STEM activities are a great way to spark a lifelong love for learning. For second graders, having lessons they can participate in can help them better understand complex subjects. There are countless activities to...
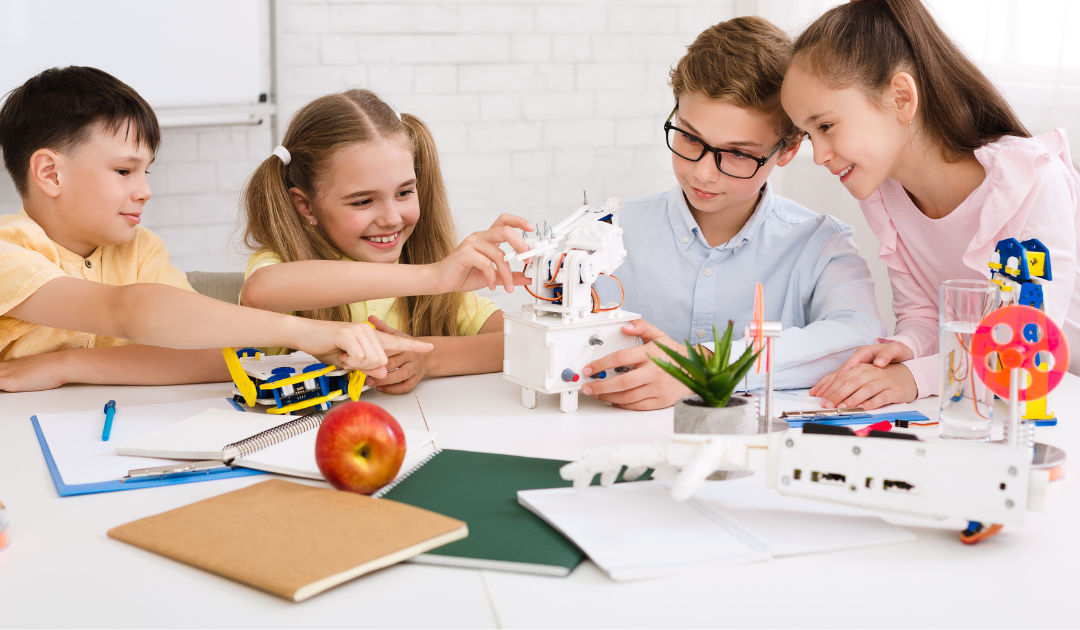
Technology Activities for Elementary Students
Technology Activities for Elementary Students As advanced as tech-savvy youngsters may be, there’s always more to learn. Elementary school is the perfect time for students to learn how to respect and understand how technology works, the ways it can be used, and how to...
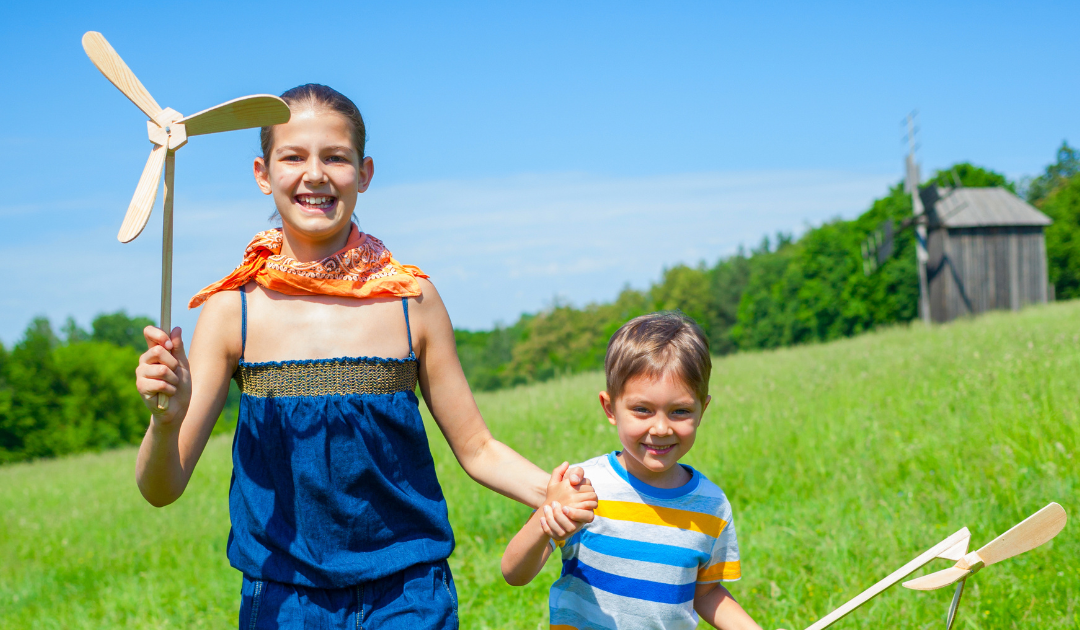
Engineering Activities for Students
Engineering Activities for Students Why Do Engineering Activities with Students? With the rapid expanding of technology and engineer-related fields of study, educators are always seeking new ways to keep their classrooms up to date! And with new standards like the...
JOIN OUR COMMUNITY
Get project ideas and special offers delivered to your inbox.
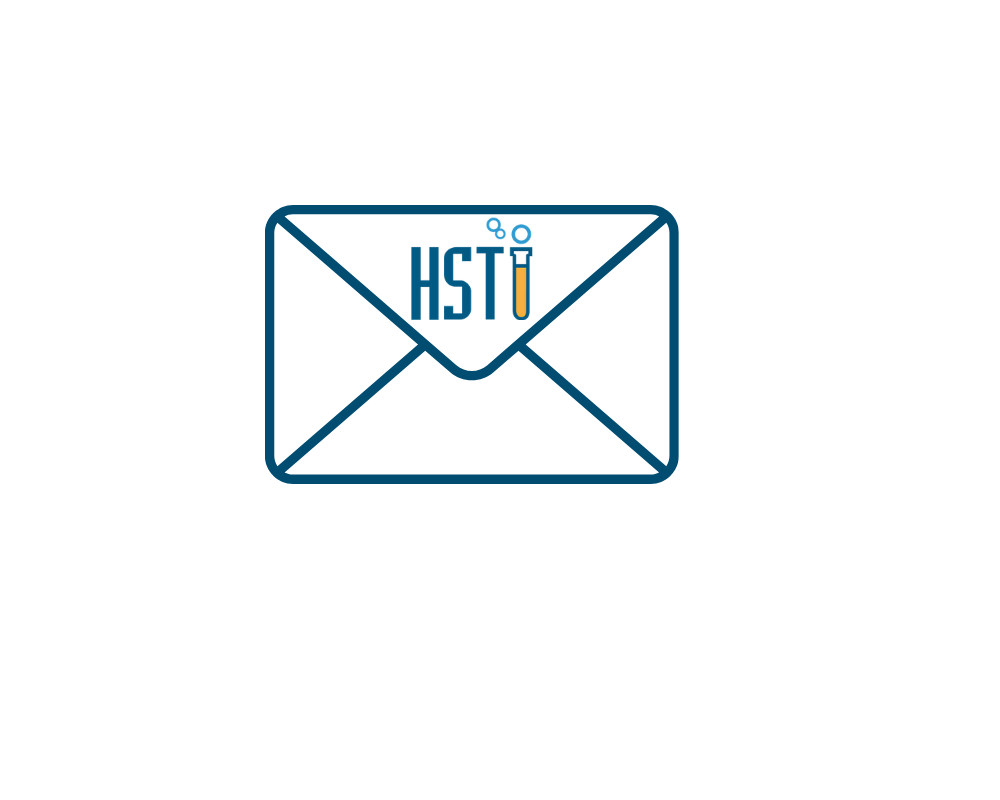

Decomposition Experiments with Hydrogen Peroxide
Ever wondered why hydrogen peroxide is stored in dark bottles science interpreter al explains chemical decomposition. don't try this at home. .
You will find out what happens to hydrogen peroxide when it comes in contact with light! You will explore how things break down in decomposition reactions, and how we can speed up those reactions using a catalyst.
- Buy Tickets
- TFI Transformation
- Accessibility
- Frequently Asked Questions
- Itineraries
- Daily Schedule
- Getting Here
- Where to Eat & Stay
- All Exhibits & Experiences
- The Art of the Brick
- Wondrous Space
- Science After Hours
- Heritage Days
- Franklin Fright
- Events Calendar
- Staff Scientists
- Benjamin Franklin Resources
- Scientific Journals of The Franklin Institute
- Professional Development
- The Current: Blog
- About Awards
- Ceremony & Dinner
- Sponsorship
- The Class of 2024
- Call for Nominations
- Committee on Science & The Arts
- Next Generation Science Standards
- Title I Schools
- Neuroscience & Society Curriculum
- STEM Scholars
- GSK Science in the Summer™
- Missions2Mars Program
- Children's Vaccine Education Program
- Franklin @ Home
- The Curious Cosmos with Derrick Pitts Podcast
- So Curious! Podcast
- A Practical Guide to the Cosmos
- Archives & Oddities
- Ingenious: The Evolution of Innovation
- The Road to 2050
- Science Stories
- Spark of Science
- That's B.S. (Bad Science)
- Group Visits
- Plan an Event
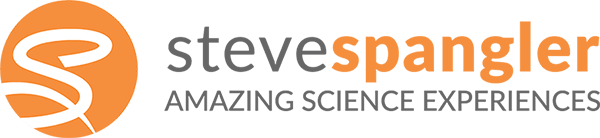
Flame Light Relight – Science Magic
Use carbon dioxide and oxygen gases to extinguish and ignite fire.
Print this Experiment
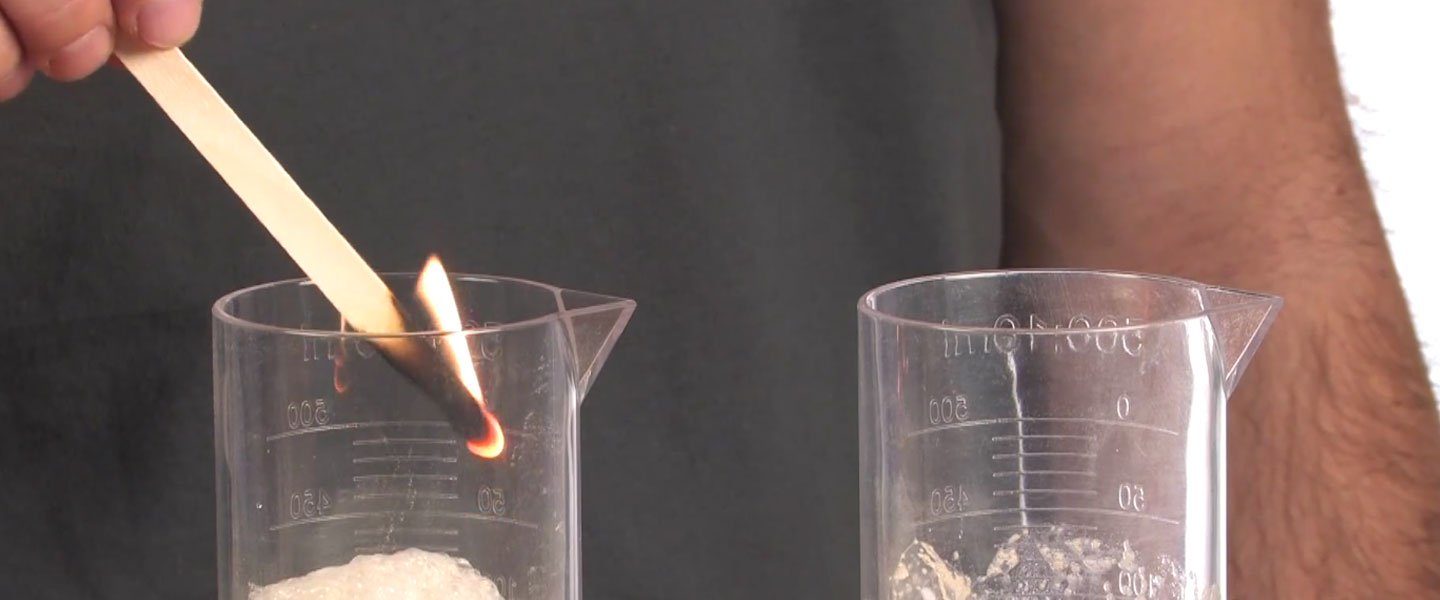
There’s a lot of chemistry behind the simple lighting or extinguishing of a flame. Would you believe us if we told you that you could extinguish a flame just by placing it partway into a graduated cylinder? What if we told you that you could relight the fire without a match or lighter? Both propositions seem unlikely, if not downright impossible. With the Flame Light Relight experiment, however, you’ll see how a few household items can mix together and create a fire-based experience you have to see to believe.
Experiment Videos
Here's What You'll Need
Two graduated cylinders (or similar containers), measuring spoons, popsicle stick, hydrogen peroxide, white vinegar, yeast (mystery powder #1), baking soda (mystery powder #2), safety glasses, adult supervision, let's try it.
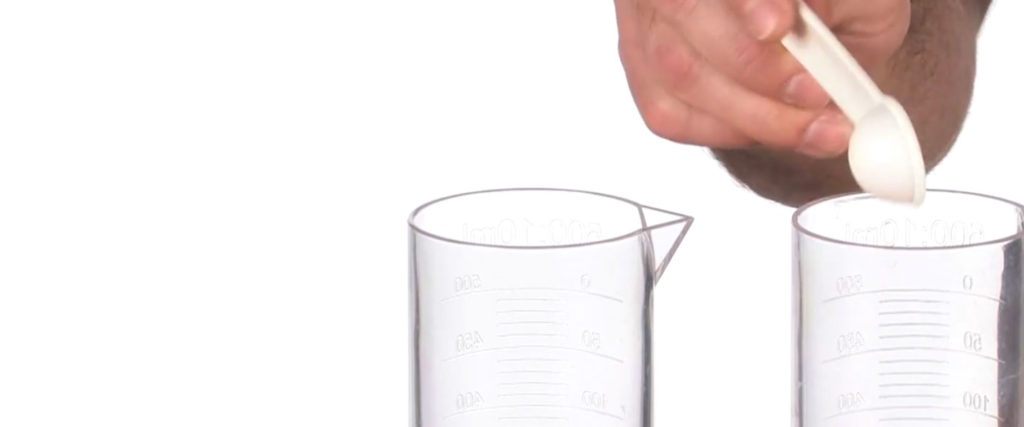
Add 1 teaspoon of baking soda to each of the two graduated cylinders.
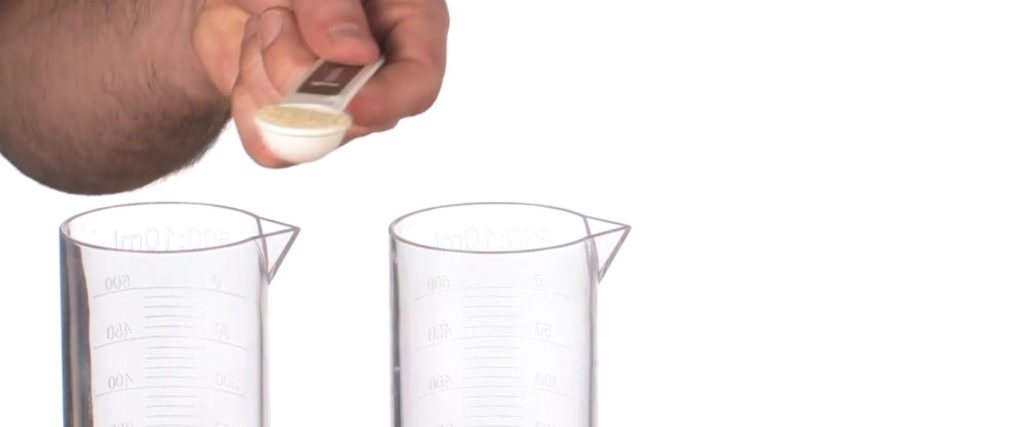
Add 1 teaspoon of yeast to both each of the graduated cylinders.
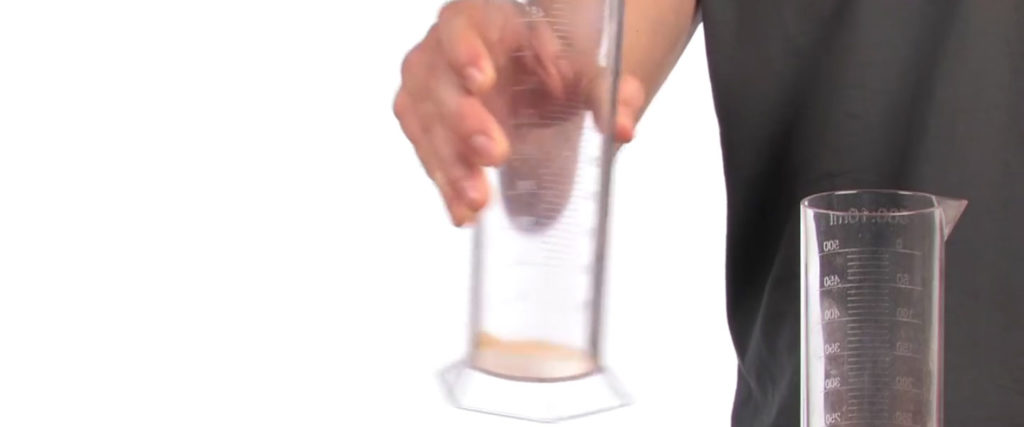
Shake, spin, and twirl the graduated cylinders to mix up the baking soda and yeast combination.
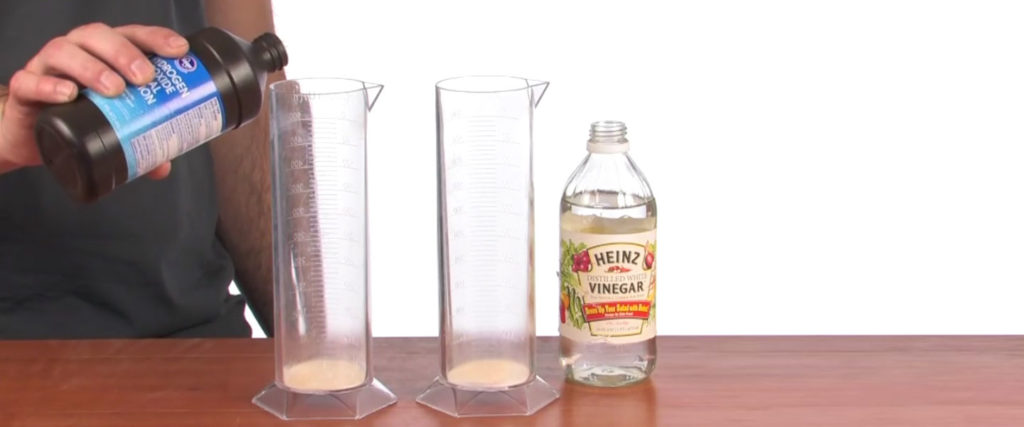
Add a generous splash of hydrogen peroxide to one of the graduated cylinders.
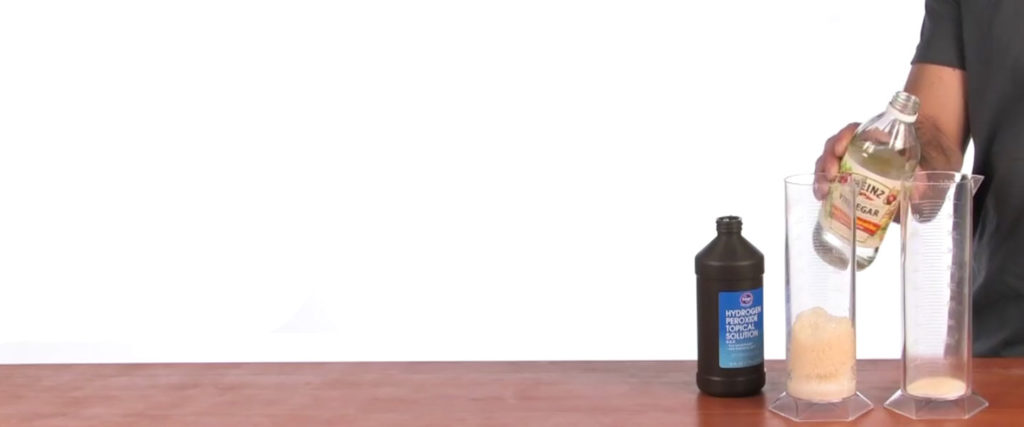
In the other graduated cylinder, pour in a generous amount of white vinegar.
The addition of hydrogen peroxide and vinegar to their respective cylinders creates a reaction with bubbling and fizzing in each of the cylinders. This is how you know you’re on the right track.
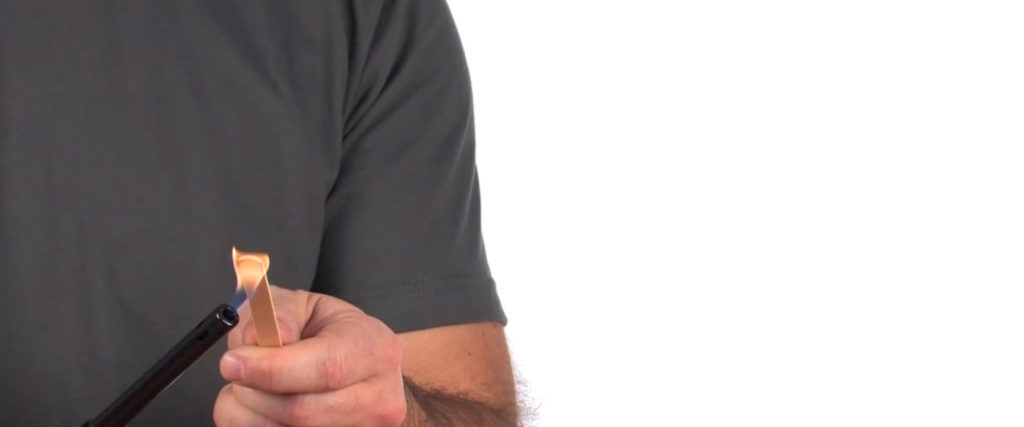
Use a lighter to ignite a popsicle stick. Make sure that you have a strong, consistent flame on the popsicle stick before continuing.
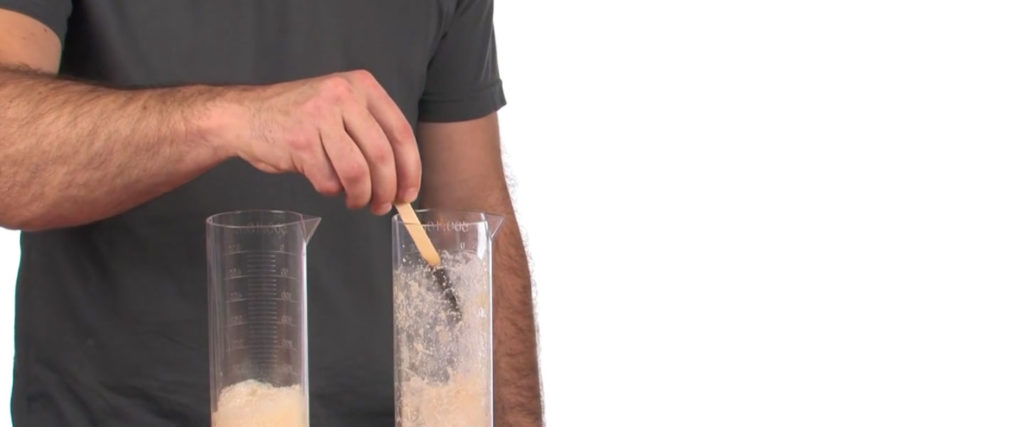
Stick the lit end of the popsicle stick down into the graduated cylinder that contains vinegar. You do not need to touch the bubbles or liquid. The flame will extinguish.
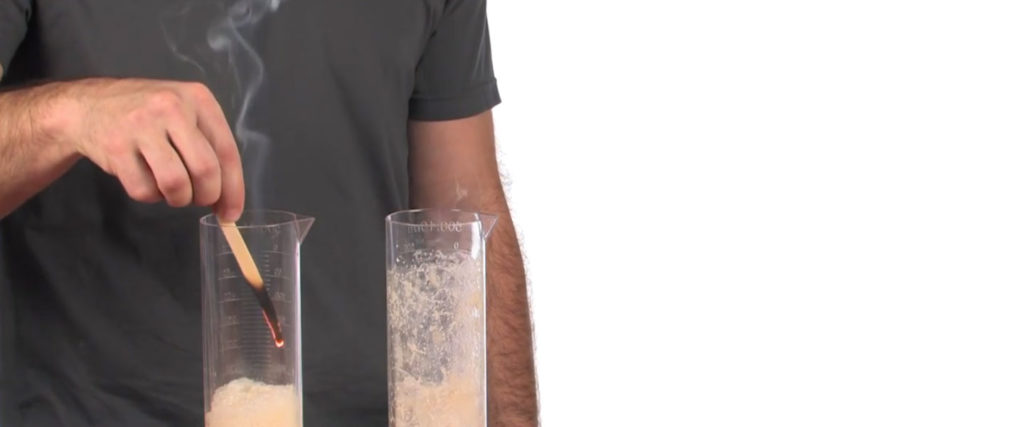
Now, move the still-smoking popsicle stick into the cylinder that contains hydrogen peroxide. Again, no need to touch the bubbles or liquid in the cylinder. The ember where a flame once was will begin to glow more intensely as it stays in the cylinder until the flame relights!
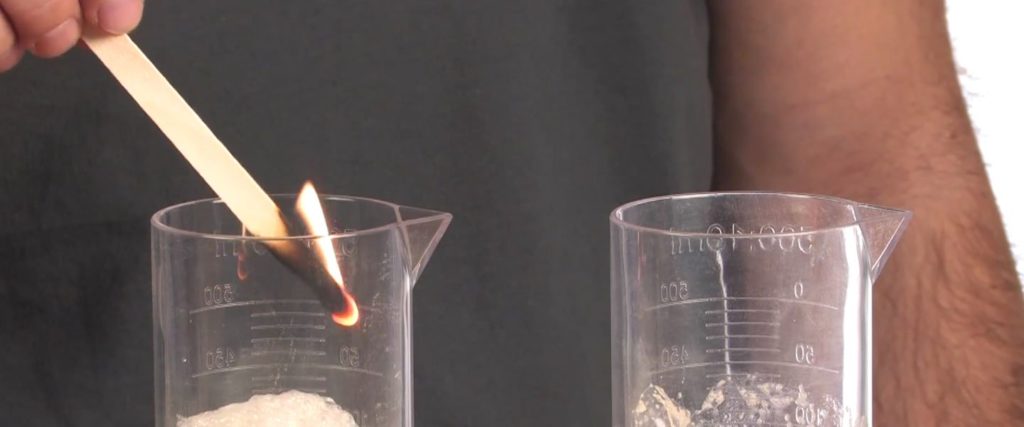
Repeat placing the popsicle stick into the vinegar cylinder and the flame will once again extinguish. Replace the popsicle stick in the hydrogen peroxide cylinder and, once again, the flame is reignited.
How Does It Work
Most flames require oxygen, fuel, and sufficient heat to ignite and stay lit. These three components of fire are referred to as the fire triangle or combustion triangle. Removal of any of the three components will cause the flame to extinguish or “go out.”
In the instance of the popsicle flame, the three necessary components are present during the initial lighting of the fire. The heat generates from a separate flame, the lighter. The wood of the popsicle stick provides the fuel. Finally, the oxygen level present in the atmosphere is enough to sustain a flame.
When you stick the flaming popsicle stick into the first graduated cylinder it extinguishes. That means one of the three components of the fire triangle is missing, but which one?
The secret lies in the bubbling mixture in the cylinder. The baking soda (also known as sodium bicarbonate) is a base. The vinegar, or acetic acid, is a weak acid. When baking soda and vinegar are combined, the immediate acid-base reaction creates carbonic acid. Carbonic acid is unstable and decomposes into carbon dioxide (CO 2 ) and water (H 2 O). The bubbling that you see inside of the cylinder is the production of the CO 2 gas. When you dip the popsicle stick into the cylinder, you’re exposing the flame to concentrated CO 2 gas. The lack of oxygen extinguishes the flame.
Upon placing the popsicle stick into the second graduated cylinder, the ember begins to glow more intensely until the flame reignites. The invisible property at work is the reintroduction of oxygen (O 2 ). The high concentration of O 2 in the cylinder makes the heat source more intense until the flame ignites again.
Hydrogen peroxide (H 2 O 2 ) is fairly unstable and always trying to decompose into water (H 2 O) and oxygen (O 2 ). When yeast is mixed with hydrogen peroxide, it acts as a catalyst to the decomposition. This creates oxygen at a much faster rate which you can see bubbling inside of the graduated cylinder. Placing the partially glowing ember into the concentrated oxygen completes the fire triangle and reignites the flame.
Science Fair Connection
Igniting and extinguishing a flame in a cylinder is cool, but it isn’t a science fair project. You can create a science fair project by identifying a variable, or something that changes, in this experiment. Let’s take a look at some of the variable options that might work:
- Try using different brands of yeast or baking soda to see if it changes the rate at which the flame ignites or extinguishes.
That’s just one idea, but you aren’t limited to that! Try coming up with different ideas of variables and give them a try. Remember, you can only change one thing at a time.
Related Experiments
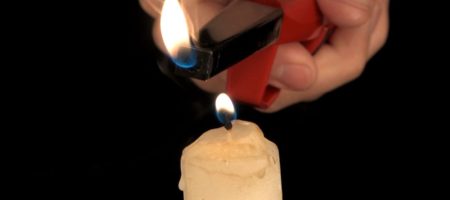
Magic Traveling Flame
You may think it’s impossible or that we have completely lost it, but we are going to show you a simple trick that will surprise […]
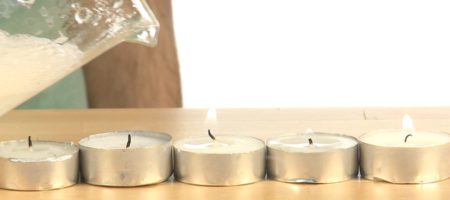
CO2 Fire Extinguisher
Carbon dioxide (CO2) is a gas that humans interact with every day. For instance, you exhale it from your lungs. Drive a vehicle and it’s in the engine […]
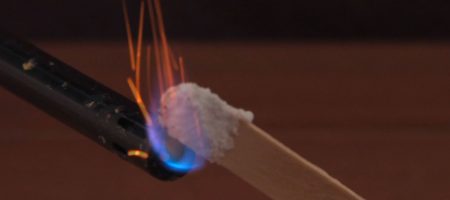
Flame Test - Colorful Elements
Blue, orange, and yellow flames are pretty common. What about green or purple flames? When you’re used to the everyday colors of flames, colorful changes […]
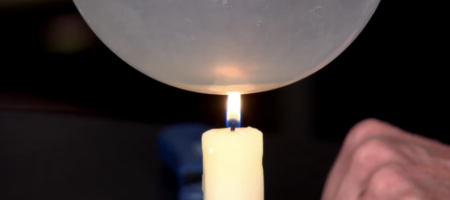
Fireproof Balloon
Common sense tells you that it’s impossible to boil water in a paper bag, but this classic parlor trick was a favorite of Victorian magicians. […]
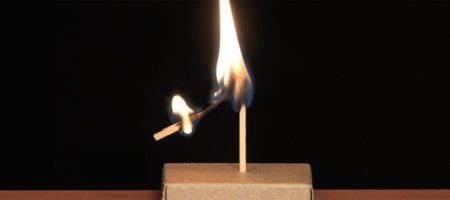
Match Levitation
Whether watching that movie about wizards or catching up on our magic tricks, we love it when things mysteriously rise into the air. We are […]
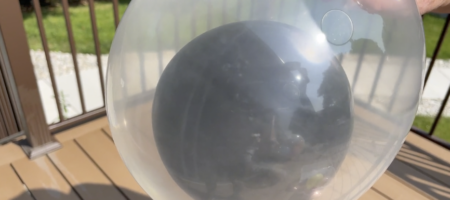
Mystery Balloon Pop
One balloon is blown up inside of another when you use a magnifying glass to focus light onto their surfaces. You expect the balloons to […]
Browse more experiments by concept:
Elephant toothpaste
If an elephant used toothpaste, this is probably what it'd look like! Learn about chemical reactions by watching this heat-producing mixture bubble and overflow for up to half an hour. The experiment comes from pages of the Nat Geo Kids book Try This! Extreme .
DON'T FORGET YOUR SAFETY EQUIPMENT: safety goggles, lab apron, nitrile gloves
Stand the soda bottle in a pan.
Insert funnel in neck of soda bottle.
Add ½ cup peroxide, detergent, and food coloring.
UM, DID YOU SEE THE WARNING ABOVE? Check it out again!
In measuring cup, beaker, or plastic cup, combine yeast and warm water. Combine with plastic spoon.
Pour yeast mixture into soda bottle and remove funnel.
WARNING: In case you missed it the first time, avoid touching or getting the chemicals on skin or clothing. And don't get too close, as heat and steam can be dangerous.
WHAT'S GOING ON
Hydrogen peroxide normally decomposes (breaks down into separate elements), and combining it with detergent and yeast (a catalyst) speeds up the process.
As the oxygen emanates from the reaction, it creates bubbles. The detergent speeds up the foaming. The reaction is "exothermic," meaning it produces heat as well as steam.
WARNING: This experiment uses chemicals that can irritate skin and damage clothes, so make sure to use safety goggles, lab apron, and nitrile gloves. Avoid touching or getting the chemicals on skin or clothing. And don't get too close, as heat and steam can be dangerous.
Science Lab
(ad) try this extreme: 50 fun & safe experiments for the mad scientist in you, (ad) make this: building thinking, and tinkering projects for the amazing maker in you, (ad) try this: 50 fun experiments for the mad scientist in you.
- Terms of Use
- Privacy Policy
- Your California Privacy Rights
- Children's Online Privacy Policy
- Interest-Based Ads
- About Nielsen Measurement
- Do Not Sell My Info
- National Geographic
- National Geographic Education
- Shop Nat Geo
- Customer Service
- Manage Your Subscription
Copyright © 1996-2015 National Geographic Society Copyright © 2015-2024 National Geographic Partners, LLC. All rights reserved
Thank you for visiting nature.com. You are using a browser version with limited support for CSS. To obtain the best experience, we recommend you use a more up to date browser (or turn off compatibility mode in Internet Explorer). In the meantime, to ensure continued support, we are displaying the site without styles and JavaScript.
- View all journals
- Explore content
- About the journal
- Publish with us
- Sign up for alerts
- Open access
- Published: 13 September 2024
Perfluoroalkyl-modified covalent organic frameworks for continuous photocatalytic hydrogen peroxide synthesis and extraction in a biphasic fluid system
- Chaochen Shao 1 , 2 ,
- Xiaohan Yu 1 , 2 ,
- Yujin Ji ORCID: orcid.org/0000-0003-3177-2073 1 , 2 ,
- Jie Xu ORCID: orcid.org/0000-0002-4506-9701 3 ,
- Yuchen Yan 1 , 2 ,
- Yongpan Hu 1 , 2 ,
- Youyong Li ORCID: orcid.org/0000-0002-5248-2756 1 , 2 ,
- Wei Huang ORCID: orcid.org/0000-0003-3808-8258 1 , 2 &
- Yanguang Li ORCID: orcid.org/0000-0003-0506-0451 1 , 2 , 4
Nature Communications volume 15 , Article number: 8023 ( 2024 ) Cite this article
Metrics details
- Organic–inorganic nanostructures
- Photocatalysis
H 2 O 2 photosynthesis represents an appealing approach for sustainable and decentralized H 2 O 2 production. Unfortunately, current reactions are mostly carried out in laboratory-scale single-phase batch reactors, which have a limited H 2 O 2 production rate (<100 μmol h −1 ) and cannot operate in an uninterrupted manner. Herein, we propose continuous H 2 O 2 photosynthesis and extraction in a biphasic fluid system. A superhydrophobic covalent organic framework photocatalyst with perfluoroalkyl functionalization is rationally designed and prepared via the Schiff-base reaction. When applied in a home-built biphasic fluid photo-reactor, the superhydrophobicity of our photocatalyst allows its selective dispersion in the oil phase, while formed H 2 O 2 is spontaneously extracted to the water phase. Through optimizing reaction parameters, we achieve continuous H 2 O 2 photosynthesis and extraction with an unprecedented production rate of up to 968 μmol h −1 and tunable H 2 O 2 concentrations from 2.2 to 38.1 mM. As-obtained H 2 O 2 solution could satisfactorily meet the general demands of household disinfection and wastewater treatments.
Similar content being viewed by others
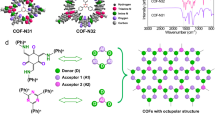
Covalent organic frameworks for direct photosynthesis of hydrogen peroxide from water, air and sunlight

Membrane-based TBADT recovery as a strategy to increase the sustainability of continuous-flow photocatalytic HAT transformations
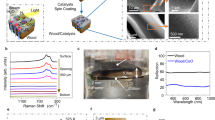
Boosting photocatalytic hydrogen production from water by photothermally induced biphase systems
Introduction.
Hydrogen peroxide (H 2 O 2 ) is one of the most essential basic chemicals for synthetic industry, environmental remediation, and medical disinfection, with an annual global demand of 4.4 million tons and a market size of USD 3.2 billion in 2022 1 , 2 . During the recent COVID-19 pandemic, its demand has risen substantially owing to its wide use in the formulation of disinfectant products 3 . At present, over 95% of commercial H 2 O 2 is manufactured through the well-established anthraquinone oxidation process in centralized plants, involving substantial energy consumption and waste emission 4 . It generally yields highly concentrated H 2 O 2 , whose storage, transportation, and handling may pose significant safety risks. On many occasions, however, end-users only need dilute H 2 O 2 solution: for example, <0.1 wt% (or ~30 mM) H 2 O 2 is usually sufficient for water treatments and antibacterial purposes 5 , 6 . This notable gap between production and consumption stimulates us to search for alternative processes to enable the on-site, on-demand production of dilute H 2 O 2 solution 7 , 8 .
Solar-driven H 2 O 2 photosynthesis from oxygen and water has emerged as a promising route 9 , 10 , 11 . It uses solar energy as the sole energy input and generates no waste chemicals throughout the reaction process. Over recent years, a variety of photocatalysts have been developed with many exciting progresses 12 , 13 , 14 , 15 . They, unfortunately, have been predominantly investigated in laboratory-scale single-phase batch reactors, in which catalyst powders are dispersed in an O 2 -saturated aqueous solution (Fig. 1a ) 14 , 16 . While such a batch configuration is easy to operate and permits quick catalyst screening, its often limited size (solution volume <50 mL) and H 2 O 2 production rate (<100 μmol h −1 ) are far from amenable to practical applications 17 . Moreover, all the batch reactors only operate intermittently, and necessitate repetitive catalyst separation and recycling at intervals as short as a few hours to extract H 2 O 2 solution, resulting in low productivity and added expenses. Several attempts have been made to address this limitation. For example, biphasic batch reactors containing liquid water and oil phases have been shown to facilitate the spontaneous separation and collection of H 2 O 2 18 , 19 . Supporting photocatalyst powders on porous hydrophobic substrates floating on the solution surface creates abundant triple-phase boundaries, and promotes O 2 mass transfer and hence H 2 O 2 production 20 , 21 . Despite some performance gains, none of them could enable the continuous photosynthesis and extraction of H 2 O 2 at practically meaningful concentrations for directly connecting to the end users.
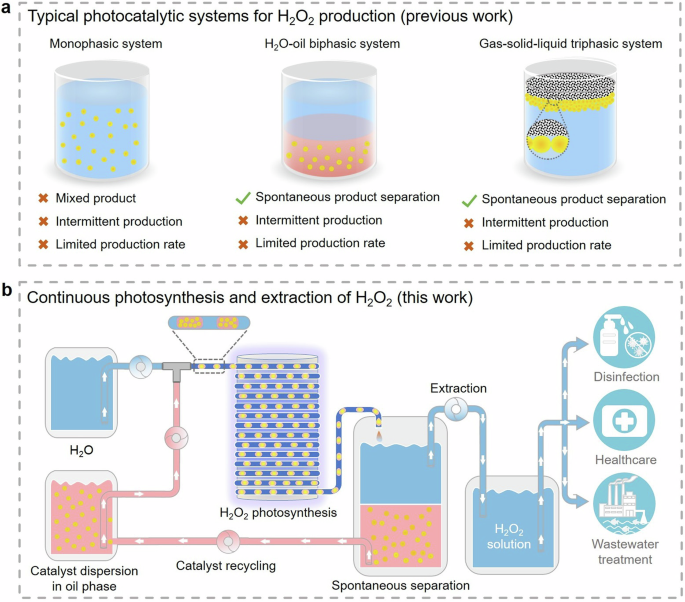
a Previously reported photocatalytic systems for H 2 O 2 production including the monophasic system, H 2 O-oil biphasic system, and gas-solid-liquid triphasic system. The colors yellow, blue, and pink represent the photocatalyst, water phase, and oil phase, respectively, while the gray grids denote the hydrophobic support. b Biphasic fluid system that enables continuous H 2 O 2 photosynthesis, separation, and extraction. The colors yellow, blue, and pink represent the photocatalyst, water phase, and oil phase, respectively.
We envision that a biphasic fluid system represents a promising solution to the above challenge (Fig. 1b ). Fluid reactors have the demonstrated potential for the continuous electrosynthesis or photosynthesis of a variety of valuable chemicals 22 , 23 , 24 , 25 , 26 . The introduction of biphasic water-oil reaction solution within fluid systems may benefit spontaneous H 2 O 2 separation while being continuously produced. To achieve so, desirable photocatalyst materials should have strong surface hydrophobicity in order to be stably and selectively dispersed in organic phases. Covalent organic frameworks (COFs)—a class of crystalline and porous polymer semiconductors—are appealing candidates by virtue of their versatile structural diversity, and tunable optoelectronic and surface properties 27 , 28 , 29 , 30 , 31 . Their interactions with solvents could, in principle, be modified by incorporating proper functional building blocks 32 . Based on the above reasoning, we here prepare a superhydrophobic COF photocatalyst via a judiciously designed Schiff-base reaction between tritopic amine and tetratopic aldehyde monomers. Their symmetry mismatch leaves periodical uncondensed aldehyde sites, which are subsequently grafted with perfluoroalkyl chains to afford the product with superhydrophobicity. This surface property allows the photocatalyst to be stably dispersed in the oil phase within a biphasic fluid photo-reactor. By properly adjusting reaction parameters, we achieve continuous production and extraction of pure H 2 O 2 solution with an exceptional production rate of up to 968 μmol h −1 and tunable H 2 O 2 concentrations from 2.2 to 38.1 mM. As-prepared H 2 O 2 solution can be used for household disinfection and environmental remediation.
Preparation and characterizations of BTTA-COF and PF-BTTA-COF
As schematically illustrated in Fig. 2a , our catalyst was prepared through a [4+3] Schiff-base condensation reaction between 5,5’-(benzo[ c ][1,2,5]thiadiazole-4,7-diyl)diisophthalaldehyde (BTDIPA) and 2,4,6-tris(4-aminophenyl)-1,3,5-triazine (TAPT) under a solvothermal condition (see the synthetic details in the Supplementary Information ). The as-prepared sample from this step is denoted as BTTA-COF. Previous studies indicated that benzothiadiazole (BT) and triazine moieties favored the two-electron oxygen reduction reaction (2e − ORR) 33 , 34 , 35 . Their abundant incorporation into polymeric frameworks is expected to accelerate H 2 O 2 photosynthesis. Moreover, the symmetry mismatch between tritopic amines and tetratopic aldehydes leaves uncondensed aldehyde sites after the reaction, which could be subsequently functionalized with other molecules for regulating the photocatalyst surface wettability. Here in order to afford BTTA-COF with superhydrophobicity, its unreacted aldehyde sites were further condensed with 1H,1H-undecafluorohexylamine (UFHA) via the Schiff-base reaction. The final product is denoted as PF-BTTA-COF to reflect its perfluoroalkyl functionalization.
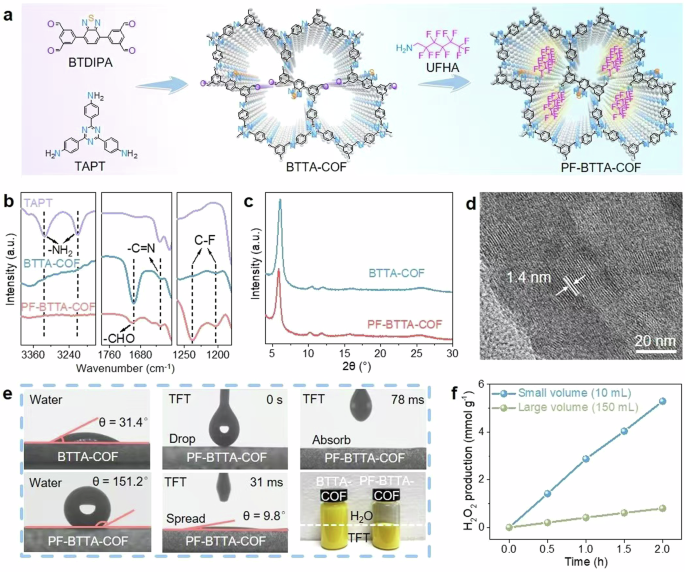
a Schematic synthetic procedure for BTTA-COF and PF-BTTA-COF. b FT-IR spectra of BTTA-COF, PF-BTTA-COF, and TAPT. c XRD patterns of BTTA-COF and PF-BTTA-COF. d TEM image of PF-BTTA-COF. e Water or α,α,α-trifluorotoluene (TFT) contact angle measurements of BTTA-COF and PF-BTTA-COF as well as the photograph showing their dispersion in biphasic H 2 O-TFT mixture. f Time-dependent H 2 O 2 evolution on BTTA-COF within batch reactors of two different sizes while retaining the same catalyst concentration.
The molecular structures of both samples were interrogated by spectroscopic characterizations. In the Fourier-transform infrared (FT-IR) spectrum of BTTA-COF, the emergence of the signature imine signal at 1627 cm −1 attests to the successful condensation between monomers (Supplementary Fig. 1a ) 36 . Its moderate signal at 1696 cm −1 indicates the existence of uncondensed aldehyde sites owing to the monomer symmetry mismatch as explained above, while no unreacted amine signal is noted in BTTA-COF (Fig. 2b ) 37 , 38 . The molar percentage of residual aldehydes is estimated to be 15%, close to the theoretical value (Supplementary Fig. 2a–c ). The subsequent introduction of perfluoroalkyl functionalities in PF-BTTA-COF does not disrupt the overall molecular structure (Supplementary Fig. 1b ). New peaks, however, are observed at 1237 cm −1 and 1201 cm −1 assignable to the C-F bonding in PF-BTTA-COF, and the signal intensity of uncondensed aldehydes significantly attenuates after modification, evidencing that perfluoroalkyl groups are successfully grafted to the polymeric frameworks 39 . Energy-dispersive X-ray spectroscopy (EDS) analysis reveals a fluorine content of 5.3 wt%, indicating that around one-third of unreacted aldehydes are modified by UFHA (Supplementary Fig. 2d, e ) 40 , 41 . The condensed molecular structure and incorporation of perfluoroalkane are also corroborated by the solid-state 13 C (Supplementary Fig. 3a ) and 19 F nuclear magnetic resonance (NMR) results (Supplementary Fig. 3b ).
Both BTTA-COF and PF-BTTA-COF feature great structural crystallinity. Their X-ray diffraction patterns (XRD) exhibit intense peaks that can be simulated by the eclipsed (AA) stacking of two-dimensional (2D) molecular layers (Fig. 2c and Supplementary Fig. 4 ). The strongest signal at 2θ = 6.1° corresponds to the (100) diffraction and evidences the in-plane ordering with a d -spacing of 14.4 Å—close to the pore-to-pore distance of the proposed structure (14 Å). N 2 sorption isotherms reveal their microporous nature (Supplementary Fig. 5a ). The Brunauer‐Emmett‐Teller (BET) specific surface area is calculated to be 1090 m 2 g −1 for BTTA-COF and 462 m 2 g −1 for PF-BTTA-COF, with an average pore size of 1.39 and 1.27 nm, respectively (Supplementary Fig. 5b ). The decreased surface area and pore size of PF-BTTA-COF result from its pore filling with perfluoroalkane as expected. Furthermore, scanning electron microscopy (SEM) imaging shows the rod-like morphology of both samples (Supplementary Fig. 6 ). High-resolution transmission electron microscopy (TEM) imaging unveils clear lattice fringes and supports their long-range structural ordering (Fig. 2d and Supplementary Fig. 7 ). Thermogravimetric analysis (TGA) under N 2 evidences the excellent thermal stability of both samples up to 500 °C (Supplementary Fig. 8 ).
Surface hydrophobicity is a prerequisite to the catalyst design for biphasic H 2 O 2 photosynthesis 18 . To investigate the effect of perfluoroalkyl functionalization, water contact angle (CA) measurements were conducted. As shown in Fig. 2e , unmodified BTTA-COF exhibits a static water CA of 31.4°, while the CA value is dramatically increased to 151.2° for PF-BTTA-COF. When α,α,α-trifluorotoluene (TFT)—a commonly used water-immiscible organic solvent—is dropped onto the surface of PF-BTTA-COF, the organic solvent quickly spreads and is absorbed in less than a second. These results reflect the superhydrophobicity and superoleophilicity of PF-BTTA-COF as a result of perfluoroalkyl functionalization. Thanks to this unique surface property, when its powder is added to an immiscible H 2 O-TFT mixture, PF-BTTA-COF immediately migrates to and becomes stably dispersed in the oil phase, forming a clear oil-water boundary. Such a feature is essential to the continuous H 2 O 2 production and extraction in our biphasic fluid system, as will be shown later. By sharp contrast, unmodified BTTA-COF does not form selective dispersion and can be suspended in both water and TFT.
We also examined the optoelectronic properties of our samples. The ultraviolet-visible (UV-Vis) diffuse reflectance spectrum of BTTA-COF displays an adsorption onset at 550 nm (Supplementary Fig. 9a ). This corresponds to an optical band gap ( E g ) of 2.58 eV according to the Tauc’s relation. Using Mott-Schottky and ultraviolet photoelectron spectroscopy (UPS) analyses, its conduction band (CB) and valence band (VB) positions are estimated to be −0.57 V and 2.01 V versus normal hydrogen electrode (NHE), respectively (Supplementary Fig. 9b, c ). The incorporation of perfluoroalkyl groups in PF-BTTA-COF does not noticeably modify the optoelectronic property (Supplementary Fig. 10a–c ). Based on their electronic structures, both samples are capable of driving simultaneous 2e − ORR and 4e − water oxidation reaction (4e − WOR) (Supplementary Figs. 9d and 10d ).
Photocatalytic measurements of BTTA-COF in a batch system
In order to evaluate the potential of our photocatalysts and to make a fair comparison with other competitors under similar conditions, we first conducted photocatalytic measurements of hydrophilic BTTA-COF in a conventional single-phase batch reactor. The catalyst powder was dispersed in 10 mL of pure water at an optimal concentration of 1 g L −1 (Supplementary Figs. 11 and 12 , see more photocatalysis details in the Supplementary Information ). Under visible light irradiation ( λ > 420 nm), BTTA-COF enables H 2 O 2 production and linear accumulation over time, yielding a total amount of 53 μmol after 2 h (Fig. 2f ). This corresponds to a H 2 O 2 production rate of 2650 μmol h −1 g −1 or a concentration accumulation rate of 2.65 mM h −1 . Control experiments show that H 2 O 2 is predominantly produced through the 2e − ORR by photogenerated electrons (Supplementary Fig. 13a ). This process involves the formation of both superoxide anion ( • O 2 − ) and endoperoxide (–O–O–) intermediates, as verified by electron paramagnetic resonance (EPR) and in-situ diffuse reflectance infrared Fourier-transform spectroscopy (DRIFTS) analyses (Supplementary Fig. 14 ), while photogenerated holes are responsible for driving the 4e − WOR to O 2 (Supplementary Fig. 13b ) 26 , 42 , 43 . Moreover, when benzyl alcohol (BA) is introduced as the sacrificial electron donor to accelerate hole consumption under otherwise identical conditions, the H 2 O 2 production rate and concentration accumulation rate are further boosted to 5691 μmol h −1 g −1 and 5.69 mM h −1 , respectively (Supplementary Fig. 15 ). The apparent quantum efficiency (AQE) at 420 nm is measured to be 18% in pure water (Supplementary Fig. 16a ) and 37.4% in the presence of BA (Supplementary Fig. 16b ). In addition, the solar-to-chemical energy conversion (SCC) efficiency is calculated to be 0.48% in pure water (Supplementary Fig. 17 ). All these metrics are comparable to other state-of-the-art candidates in pure water or aqueous solutions involving sacrificial electron donors under similar conditions (Supplementary Table 1 ) 19 , 26 , 42 , 43 , 44 , 45 , 46 , 47 , 48 , 49 .
BTTA-COF has the required stability for H 2 O 2 photosynthesis in pure water. It shows great compatibility with H 2 O 2 , and does not catalyze H 2 O 2 degradation even under visible light irradiation (Supplementary Fig. 18 ). The cycling photocatalytic experiment demonstrates a negligible activity loss after 6 reaction cycles and a total of 30 h (Supplementary Fig. 19 ). Characterizations of the catalyst retrieved after the cycling experiment reveal no discernable change in its structure or optoelectronic properties (Supplementary Fig. 20 ). Note that PF-BTTA-COF exhibits a comparable photocatalytic activity to BTTA-COF under identical reaction conditions, which is expected given their similar optoelectronic properties (Supplementary Fig. 21 ).
Despite their wide use in photocatalytic measurements, single-phase batch reactors are not amenable to practical applications owing to their limited solution volumes and intermittent operation. We attempted to address the first issue by enlarging the batch reactor and increasing the solution volume to 150 mL (15 times larger) while maintaining the same catalyst concentration and light illumination intensity. Unfortunately, the H 2 O 2 yield does not proportionally increase: the total amount only increases 3 times in pure water, while the normalized H 2 O 2 production rate and concentration accumulation rate are substantially lowered to 0.4 mM h −1 and 400 μmol h −1 g −1 , respectively (Fig. 2f ). This leads us to conclude that we cannot simply scale up the reaction in batch reactors owing to insufficient light penetration depth and catalyst utilization in large sized reactors 50 . More importantly, batch reactors are not suitable for continuous H 2 O 2 production and extraction, which we aim for in this study.
Continuous H 2 O 2 photosynthesis and extraction by PF-BTTA-COF in a biphasic fluid system
A biphasic fluid system was developed to address the unavoidable drawbacks of conventional batch reactors. It consists of three main components, as depicted by the photo in Fig. 3a . O 2 -saturated photocatalyst dispersion in an organic solvent and pure water are co-fed into a T-shaped mixer through peristaltic pumps at controlled flow rates. This generates a series of stable oil-water biphasic segments inside transparent tubular flow channels, which are subsequently transported into a coiled reactor (total channel length ~44 m) irradiated by a set of light-emitting diodes ( λ = 455 nm, 25 mW cm −2 ). During the reaction, the small diameter (~1.6 mm) of flow channels reduces the light penetration path when side-irradiated; the presence of abundant biphasic oil-water interfaces promotes H 2 O 2 extraction from the oil phase to the water phase (Fig. 3b ). The oil/water segment lengths and density of the biphasic interfaces could be readily tuned by varying the relative liquid feeding rates (Fig. 3c ). After passing through the coiled reactor, the H 2 O 2 solution and photocatalyst dispersion are fed to a collector, where they spontaneously separate into two layers of liquids due to their immiscibility (Supplementary Fig. 22 ). The upper H 2 O 2 solution is extracted for practical applications, while the lower photocatalyst dispersion is pumped back for subsequent use. Such a biphasic fluid system can continuously yield pure H 2 O 2 solution with tunable concentrations.
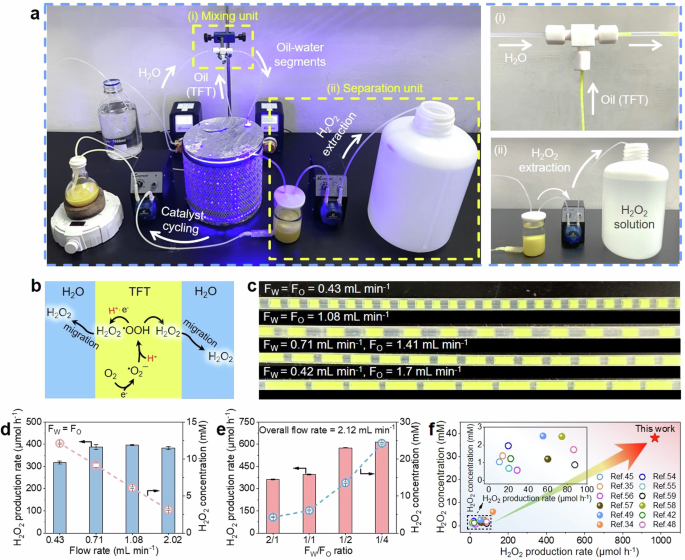
a Photographs of the home-built biphasic fluid photocatalytic system in operation. Dashed squares highlight (i) the T-shape mixer for mixing water and oil flows and (ii) spontaneous product separation and collection. b Schematic illustration of H 2 O 2 formation in TFT and migration across the oil-water interfaces. c Typical images of oil-water segments formed inside tubular flow channels at different flow rates and F W /F O ratios. d H 2 O 2 production rate and average solution concentration from the biphasic fluid system at different flow rates (F W = F O ). Error bars represent the standard deviations of three independent experiments. e H 2 O 2 production rate and average solution concentration at different F W /F O ratios (F W + F O = 2.12 mL min −1 ). Error bars represent the standard deviations of three independent experiments. f Performance comparison of PF-BTTA-COF in our biphasic fluid system with those of other state-of-the-art photocatalysts in terms of H 2 O 2 production rate and concentration. Open and filled circles represent the studies in pure water and in the presence of sacrificial electron donors, respectively.
We used superhydrophobic PF-BTTA-COF in the above-developed biphasic fluid system for continuous H 2 O 2 production as a proof of concept. TFT was chosen as the organic solvent in our study considering its excellent wetting of PF-BTTA-COF, complete immiscibility with water, and high oxygen solubility 51 , 52 . Our catalyst powder was dispersed in TFT at a concentration of 2 g L −1 . When the feeding rates of water (F W ) and TFT (F O ) are both set at 0.43 mL min −1 to start with, the catalyst retention time inside the coiled reactor is about 100 min, and our biphasic fluid system continuously produces pure H 2 O 2 solution at a rate of 99 μmol h −1 (Supplementary Fig. 23 ). The introduction of BA in TFT as the sacrificial electron donor further enhances the H 2 O 2 production rate to 318 μmol h −1 and yields H 2 O 2 solution at a concentration of 12.2 mM. Note that BA and its oxidation product benzaldehyde have much higher solubility in TFT than in water, as evidenced by the 1 H NMR analysis (Supplementary Fig. 24 ). This is essential for biphasic H 2 O 2 photosynthesis. As a result, our following optimization is approached in the presence of BA.
We examined the effect of liquid flow rates on photocatalytic activities. Increasing the flow rates is expected to reduce the catalyst retention time in the coiled reactor, and therefore decrease the attainable H 2 O 2 concentration. For example, when both F W and F O are increased to 0.71 mL min −1 , the H 2 O 2 concentration is lowered to 9.1 mM; it is further lowered to 6.1 or 3.1 mM at F W = F O = 1.08 or 2.02 mL min −1 , respectively (Fig. 3d ). Varying the overall flow rate while keeping F W = F O does not significantly change the H 2 O 2 production rate (300 ~ 400 μmol h −1 ). This is because equal F W and F O values always result in equi-length water and oil segments in tubular flow channels and thereby similar light utilization efficiency. We also investigated the effect of the F W /F O ratio while keeping the same overall flow rate (2.12 mL min −1 ). At a large F W /F O ratio of 2, the H 2 O 2 production rate is measured to be 363 μmol h −1 , and the H 2 O 2 concentration is 4.3 mM (Fig. 3e ). Both values improve with decreasing F W /F O ratios due to the enhanced light utilization efficiency by the catalyst dispersed in oil. At the lowest F W /F O ratio of 1/4 (as limited by our peristaltic pumps), the H 2 O 2 production rate and H 2 O 2 concentration are measured to be 616 μmol h −1 and 24.3 mM, respectively. Furthermore, lowering the pH value of the water phase with dilute acid is found to promote H 2 O 2 photosynthesis. For example, the H 2 O 2 production rate of PF-BTTA-COF is boosted to 847 μmol h −1 at pH = 3 and the optimal flow rates, while alkaline pH adversely affects the performance owing to spontaneous H 2 O 2 decomposition under the alkaline condition (Supplementary Fig. 25a ) 53 . At last, rising the BA content in TFT to 50 vol% further enhances the H 2 O 2 production rate and concentration to 968 μmol h −1 and 38.1 mM (0.13 wt%) respectively under the optimal flow rates and water pH (Supplementary Fig. 25b ). To our best knowledge, such extraordinary H 2 O 2 production rate is 1 ~ 2 order of magnitude greater than all earlier studies (10 ~ 100 μmol h −1 ) (Fig. 3f and Supplementary Table 1 ), thereby unambiguously underlining the unique advantage of our biphasic fluid system 34 , 35 , 45 , 48 , 49 , 54 , 55 , 56 , 57 , 58 , 59 .
We next carried out continuous H 2 O 2 photosynthesis using our biphasic fluid system at F W = 1.04 mL min −1 and F O = 2.08 mL min −1 . As shown in Fig. 4a , the total H 2 O 2 amount linearly accumulates at the first 80 h, and the increment slightly slows down as the reaction proceeds. After 100 h, more than 35 mmol of H 2 O 2 is produced with an average H 2 O 2 production rate of 357 μmol h −1 , eventually giving rise to more than 6 L of H 2 O 2 solution with a concentration of 5.7 mM (Fig. 4b ). The collected liquid product can be directly used for multiple applications. Using two bacteria Staphylococcus aureus ( S. aureus ) and Escherichia coli ( E. coli ), as examples, we find that their growth is fully inhibited with the application of 5.7 mM H 2 O 2 solution (Fig. 4c and Supplementary Fig. 26 ). Our product solution can also be employed in wastewater treatment as simulated by the Fenton reaction in the presence of methyl blue (MB) or methyl orange (MO) at practically relevant concentrations (100 ppm) 60 . Both organic dyes are observed to totally degrade within 30 s after the introduction of 5.7 mM H 2 O 2 solution (Fig. 4d, e and Supplementary Fig. 27 ). The above results showcase that the dilute H 2 O 2 solution produced from our biphasic fluid system can satisfactorily meet the demands of household disinfection and environmental remediation. The solution could be stored for over 1 month without significant degradation (Supplementary Fig. 28 ).

a Total amount of H 2 O 2 produced and its concentration change over time during an uninterrupted 100 h test in our biphasic fluid system. b Photographs of the as-obtained H 2 O 2 solution. c Antibacterial tests against S. aureus and E. coli using the as-obtained H 2 O 2 solution. d , e Degradation of d MB and e MO using the as-obtained H 2 O 2 solution, insets are the photographs showing the dye decolorization.
To evaluate the economic feasibility of our biphasic fluid system, we carried out a techno-economic analysis (TEA) based on an amplified reactor device to determine the levelized cost of the product (LCP) and the end-of-life net present value (NPV) 61 , 62 . The estimation of the capital and operational costs is based on the prevailing market price of raw materials and products, as summarized in Supplementary Tables 2 and 3 . It is found that when the F W /F O ratio is set at 1/4, LCP is most economical (Supplementary Fig. 29a ). Assuming a facility lifespan of 10 years, the end-of-life NPV turns profitable by the fourth year (Supplementary Fig. 29b ). These results demonstrate the practical viability of the biphasic fluid system in H 2 O 2 production.
In summary, we here demonstrated an innovative strategy for continuous H 2 O 2 photosynthesis and extraction. The success key lies in the judicious design of both the photocatalyst material and the reactor. PF-BTTA-COF was prepared via the Schiff-base reaction between tritopic amine and tetratopic aldehyde monomers, followed by perfluoroalkyl functionalization at uncondensed aldehyde sites to afford its surface superhydrophobicity. Such a surface property allows the photocatalyst to be selectively and stably dispersed in oil instead of water. We then constructed a biphasic fluid system by co-feeding water and O 2 -saturated catalyst-dispersed TFT through transparent tubular flow channels to a coiled reactor under irradiation. The immiscibility of these two liquid phases led to the formation of a series of stable TFT-water biphasic segments with clear interfaces that promoted H 2 O 2 spontaneous extraction. By properly adjusting reaction conditions, we achieved an unprecedented H 2 O 2 production rate of up to 968 μmol h −1 and tunable H 2 O 2 concentrations from 2.2 to 38.1 mM. As-obtained H 2 O 2 solution could be directly supplied to end-users where and when it is needed, and can satisfactorily meet the practical requirements of disinfection and wastewater treatments. Moreover, our biphasic fluid system could be readily scaled up by increasing the channel length or connecting several coiled reactors in parallel or in series.
Synthesis of BTTA-COF and PF-BTTA-COF
Typically, BTDIPA (30.0 mg, 0.075 mmol) and 2,4,6-tris(4-aminophenyl)-1,3,5-triazine (TAPT) (26.6 mg, 0.075 mmol) were ultrasonically dispersed in a mixture of o -dichlorobenzene, n -butyl alcohol, and 6 M acetic acid (4.4 mL, 5/5/1, v/v/v) in a 25 mL Pyrex tube. The tube was degassed by three vacuum-N 2 filling cycles, sealed under vacuum, and heated at 120 °C for 72 h. After cooled down to room temperature, the solid was collected by centrifugation, thoroughly washed with N , N -dimethylformamide (commercial sources and purities), anhydrous tetrahydrofuran, and acetone, respectively, and finally dried under vacuum at 80 °C overnight to afford BTTA-COF as a light-yellow powder (yield: 88%). For the synthesis of PF-BTTA-COF, BTTA-COF (100 mg) was ultrasonically dispersed in ethanol (5 mL), then added with UFHA (500 µL, 2.5 mmol) and acetic acid (50 µL) under stirring. The mixture was stirred at room temperature for 5 h under N 2 . The product was isolated by filtration, thoroughly washed with ethanol, and dried under vacuum at 80 °C overnight.
Determination of H 2 O 2 concentration
The H 2 O 2 concentration was determined using a colorimetric method as described in our previous publication 34 . Typically, a ferrous ion oxidation xylenol orange (FOX) solution was prepared by dissolving Fe(NH 4 ) 2 (SO 4 ) 2 ·6H 2 O (19.61 mg), D-sorbitol (3.644 mg), and xylenol orange (XO) (14.333 mg) in deionized water (200 mL) added with ethanol (2 mL) and H 2 SO 4 (98%, 272 μL). Subsequently, 50 μL of the obtained H 2 O 2 solution (diluted if needed) was mixed with the pre-prepared FOX solution. The concentration of H 2 O 2 was quantified by monitoring the characteristic absorption peak at 550 nm via UV-Vis spectroscopy according to the calibration curve (Supplementary Fig. 11 ).
Photocatalytic H 2 O 2 production in a batch system
In a typical batch photocatalytic reaction, 10 mg of photocatalyst was dispersed in 10 mL of pure water (or with 10 vol% BA) inside a top-irradiated Pyrex reactor (120 mL). The suspension was first bubbled with O 2 for 30 min before the reactor was carefully sealed. During the photocatalytic reaction, the reactor was irradiated by a 300 W Xe-lamp (China Education Au-light, CEL-HXF300) with a cutoff filter of 420 nm. The light intensity was calibrated to be 200 mW cm −2 using a Newport light-power meter (Model 1918-R). To monitor the reaction process, the reaction solution was extracted every 1 h and filtrated through a syringe filter (0.22 μm) to remove the photocatalyst powder. The resulting H 2 O 2 concentration was quantified using the FOX solution.
Photocatalytic H 2 O 2 production in the biphasic fluid system
In a typical photocatalytic reaction, 140 mg of PF-BTTA-COF photocatalyst was ultrasonically dispersed in 70 mL of TFT (with or without 10 vol% BA). The TFT dispersion (oil phase) and distilled water (water phase) were separately bubbled with O 2 for 30 min, and then pumped through a T-shape valve at specific feeding rates by two peristaltic pumps and mixed together. This led to the formation of consecutive oil-water segments, which were fed into a coiled tubular reactor made of polypropylene tubing ( Φ 1.6 × 3.2 mm). The total tube length in the reactor is 44 m, corresponding to a total volume of ~90 mL. The solution retention time in the flow channel was controlled by the overall flow rate of water and oil. Inside the coiled reactor, the oil-water segments were side-irradiated by a set of LED arrays with a wavelength of 455 nm and a light intensity of 25 mW cm −2 . During the photocatalytic reaction, generated H 2 O 2 would migrate across abundant oil-water interfaces and accumulate in the water phase. After passing through the coiled reactor, the oil-water mixed solution was collected in a container, where phase separation occurred spontaneously due to the immiscibility of water and TFT. The upper H 2 O 2 aqueous solution was directly extracted using a peristaltic pump for subsequent uses, while the lower photocatalyst dispersion was pumped back for the next reaction cycle. Such a system achieved continuous H 2 O 2 production and extraction as well as photocatalyst recycling. The H 2 O 2 concentration at the outlet was determined every 1 h using the FOX solution.
Long-term continuous H 2 O 2 photosynthesis in the biphasic system
The long-term continuous photocatalysis in the biphasic fluid system was conducted under similar conditions. 1.2 g of PF-BTTA-COF was dispersed in 300 mL of O 2 -saturated TFT and BA (30 mL, 9:1, v/v). The flow rates of oil and water phases were kept at 1.04 mL min −1 and 2.08 mL min −1 , respectively. The H 2 O 2 concentration at the outlet was determined every 2 h using the FOX solution.
Antibacterial experiments
The antibacterial effect of the as-obtained H 2 O 2 solution was estimated by the plate colony counting method. Staphylococcus aureus ( S. aureus ) and Escherichia coli ( E. coli ) were selected as the target bacteria. An individual colony was first cultured in a fresh LB agar plate by shaking at a speed of 200 rpm at 37 °C for 12 h. Then, 30 µL of the initial colony solution was subsequently diluted 100-fold and shaken for another 3 h at 37 °C to ensure bacterial growth in the log-phase, eventually resulting in ~10 6 colony-forming units (CFU) per milliliter. Then 100 µL of the bacterial solution was incubated with the as-obtained H 2 O 2 solution (500 µL, 5.7 mM) for 3 h. The colonies were photographed after 24 h incubation at 37 °C. Control experiments were conducted under identical conditions except that H 2 O 2 solution was not added.
Dye degradation experiments
Methyl blue (MB) and methyl orange (MO) were chosen as representative organic dyes for the degradation experiments using the Fenton reaction process. In a typical experiment, a stock solution containing dye (100 ppm) and FeSO 4 (6 mM) was prepared, and its pH value was adjusted to 3.0 using diluted H 2 SO 4 . Subsequently, 2 mL of the as-obtained H 2 O 2 solution (5.7 mM) was added to 2 mL of the above-mentioned dye solution in the dark. The absorbance of the organic dye at its maximum absorption wavelength was recorded using UV-Vis spectroscopy, and its concentration was calculated based on the calibration curve with standard solutions.
Data availability
All the data that support the findings of this study are provided in the Supplementary Information . Other data are available from the corresponding author upon request. Source data are provided with this paper.
Ciriminna, R., Albanese, L., Meneguzzo, F. & Pagliaro, M. Hydrogen peroxide: a key chemical for today’s sustainable development. ChemSusChem 9 , 3374–3381 (2016).
Article CAS PubMed Google Scholar
McDonnell, G. The use of hydrogen peroxide for disinfection and sterilization applications in Patai’s Chemistry of Functional Groups , 1–34 (Wiley, 2014).
Kumar, G. Hydrogen Peroxide Price Trend, Monitor, Supply & Demand, Forecast . https://www.linkedin.com/pulse/hydrogen-peroxide-price-trend-monitor-supply-demand-kumar-gupta-fyu9c (2024).
Campos‐Martin, J. M., Blanco‐Brieva, G. & Fierro, J. L. G. Hydrogen peroxide synthesis: an outlook beyond the anthraquinone process. Angew. Chem. Int. Ed. Engl. 45 , 6962–6984 (2006).
Article PubMed Google Scholar
Pi, L. et al. Generation of H 2 O 2 by on-site activation of molecular dioxygen for environmental remediation applications: a review. Chem. Eng. J. 389 , 123420 (2020).
Article CAS Google Scholar
Xia, C., Xia, Y., Zhu, P., Fan, L. & Wang, H. Direct electrosynthesis of pure aqueous H 2 O 2 solutions up to 20% by weight using a solid electrolyte. Science 366 , 226–231 (2019).
Article ADS CAS PubMed Google Scholar
Yang, S. et al. Toward the decentralized electrochemical production of H 2 O 2 : a focus on the catalysis. ACS Catal. 8 , 4064–4081 (2018).
Sun, Y., Han, L. & Strasser, P. A comparative perspective of electrochemical and photochemical approaches for catalytic H 2 O 2 production. Chem. Soc. Rev. 49 , 6605–6631 (2020).
Hou, H., Zeng, X. & Zhang, X. Production of hydrogen peroxide by photocatalytic processes. Angew. Chem. Int. Ed. Engl. 59 , 17356–17376 (2020).
Qu, S., Wu, H. & Ng, Y. H. Clean production of hydrogen peroxide: a heterogeneous solar‐driven redox process. Adv. Energy Mater. 13 , 2301047 (2023).
Freese, T., Meijer, J. T., Feringa, B. L. & Beil, S. B. An organic perspective on photocatalytic production of hydrogen peroxide. Nat. Catal. 6 , 553–558 (2023).
Wang, L. et al. Inorganic metal‐oxide photocatalyst for H 2 O 2 production. Small 18 , 2104561 (2021).
Article Google Scholar
Kondo, Y., Kuwahara, Y., Mori, K. & Yamashita, H. Design of metal-organic framework catalysts for photocatalytic hydrogen peroxide production. Chem 8 , 2924–2938 (2022).
Yu, X., Hu, Y., Shao, C., Huang, W. & Li, Y. Polymer semiconductors: a unique platform for photocatalytic hydrogen peroxide production. Mater. Today 71 , 152–173 (2023).
Yong, Z. & Ma, T. Solar‐to‐H 2 O 2 catalyzed by covalent organic frameworks. Angew. Chem. Int. Ed. Engl. 62 , e202308980 (2023).
Zeng, X., Liu, Y., Hu, X. & Zhang, X. Photoredox catalysis over semiconductors for light-driven hydrogen peroxide production. Green. Chem. 23 , 1466–1494 (2021).
Ding, Y. et al. Emerging semiconductors and metal-organic-compounds-related photocatalysts for sustainable hydrogen peroxide production. Matter 5 , 2119–2167 (2022).
Isaka, Y., Kawase, Y., Kuwahara, Y., Mori, K. & Yamashita, H. Two‐phase system utilizing hydrophobic metal-organic frameworks (MOFs) for photocatalytic synthesis of hydrogen peroxide. Angew. Chem. Int. Ed. Engl. 58 , 5402–5406 (2019).
Zhao, W. et al. Accelerated synthesis and discovery of covalent organic framework photocatalysts for hydrogen peroxide production. J. Am. Chem. Soc. 144 , 9902–9909 (2022).
Article CAS PubMed PubMed Central Google Scholar
Li, L., Xu, L., Hu, Z. & Yu, J. C. Enhanced mass transfer of oxygen through a gas-liquid-solid interface for photocatalytic hydrogen peroxide production. Adv. Funct. Mater. 31 , 2106120 (2021).
Yan, S. et al. Photocatalytic H 2 O 2 generation reaction with a benchmark rate at air-liquid-solid joint interfaces. Adv. Mater. 36 , 2307967 (2023).
Cambié, D., Bottecchia, C., Straathof, N. J. W., Hessel, V. & Noël, T. Applications of continuous-flow photochemistry in organic synthesis, material science, and water treatment. Chem. Rev. 116 , 10276–10341 (2016).
Pieber, B., Shalom, M., Antonietti, M., Seeberger, P. H. & Gilmore, K. Continuous heterogeneous photocatalysis in serial micro‐batch reactors. Angew. Chem. Int. Ed. Engl. 57 , 9976–9979 (2018).
Byun, J., Hong, Y. & Zhang, K. A. I. Beyond the batch: process and material design of polymeric photocatalysts for flow photochemistry. Chem. Catal. 1 , 771–781 (2021).
Wen, Y. et al. Electrochemical reactors for continuous decentralized H 2 O 2 production. Angew. Chem. Int. Ed. Engl. 61 , e202205972 (2022).
Liu, R. et al. Linkage-engineered donor-acceptor covalent organic frameworks for optimal photosynthesis of hydrogen peroxide from water and air. Nat. Catal. 7 , 195–206 (2024).
Huang, W., Luo, W. & Li, Y. Two-dimensional semiconducting covalent organic frameworks for photocatalytic solar fuel production. Mater. Today 40 , 160–172 (2020).
Liu, R. et al. Covalent organic frameworks: an ideal platform for designing ordered materials and advanced applications. Chem. Soc. Rev. 50 , 120–242 (2021).
Gong, Y.-N., Guan, X. & Jiang, H.-L. Covalent organic frameworks for photocatalysis: synthesis, structural features, fundamentals and performance. Coord. Chem. Rev. 475 , 214889 (2023).
Qian, Y. et al. Computation-based regulation of excitonic effects in donor-acceptor covalent organic frameworks for enhanced photocatalysis. Nat. Commun. 14 , 3083 (2023).
Article ADS CAS PubMed PubMed Central Google Scholar
Qian, Y. & Jiang, H.-L. Structural regulation of covalent organic frameworks for catalysis. Acc. Chem. Res. 57 , 1214–1226 (2024).
Huang, W. et al. Highly crystalline and water-wettable benzobisthiazole-based covalent organic frameworks for enhanced photocatalytic hydrogen production. Natl Sci. Rev. 10 , nwac171 (2023).
Chen, L. et al. Acetylene and diacetylene functionalized covalent triazine frameworks as metal‐free photocatalysts for hydrogen peroxide production: a new two‐electron water oxidation pathway. Adv. Mater. 32 , 1904433 (2019).
Shao, C. et al. A covalent organic framework inspired by C 3 N 4 for photosynthesis of hydrogen peroxide with high quantum efficiency. Chin. J. Catal. 46 , 28–35 (2023).
Chang, J. N. et al. Oxidation‐reduction molecular junction covalent organic frameworks for full reaction photosynthesis of H 2 O 2 . Angew. Chem. Int. Ed. Engl. 62 , e202218868 (2023).
Ding, S.-Y. et al. Construction of covalent organic framework for catalysis: Pd/COF-LZU1 in Suzuki–Miyaura coupling reaction. J. Am. Chem. Soc. 133 , 19816–19822 (2011).
Liao, Q. et al. Donor-acceptor type [4+3] covalent organic frameworks: sub-stoichiometric synthesis and photocatalytic application. Sci. China Chem. 63 , 707–714 (2020).
Lu, M. et al. Confining and highly dispersing single polyoxometalate clusters in covalent organic frameworks by covalent linkages for CO 2 photoreduction. J. Am. Chem. Soc. 144 , 1861–1871 (2022).
Sun, Q. et al. Integrating superwettability within covalent organic frameworks for functional coating. Chem 4 , 1726–1739 (2018).
Enders, A. A., North, N. M., Fensore, C. M., Velez-Alvarez, J. & Allen, H. C. Functional group identification for FTIR spectra using image-based machine learning models. Anal. Chem. 93 , 9711–9718 (2021).
Dai, F., Zhuang, Q., Huang, G., Deng, H. & Zhang, X. Infrared spectrum characteristics and quantification of OH groups in coal. ACS Omega 8 , 17064–17076 (2023).
Liao, Q. et al. Regulating relative nitrogen locations of diazine functionalized covalent organic frameworks for overall H 2 O 2 photosynthesis. Angew. Chem. Int. Ed. Engl. 62 , e202310556 (2023).
Yu, H. et al. Vinyl-group-anchored covalent organic framework for promoting the photocatalytic generation of hydrogen peroxide. Angew. Chem. Int. Ed. Engl. 63 , e202402297 (2024).
Zhi, Q. et al. Piperazine-linked metalphthalocyanine frameworks for highly efficient visible-light-driven H 2 O 2 photosynthesis. J. Am. Chem. Soc. 144 , 21328–21336 (2022).
Kou, M. et al. Molecularly engineered covalent organic frameworks for hydrogen peroxide photosynthesis. Angew. Chem. Int. Ed. Engl. 61 , e202200413 (2022).
Liu, Y. et al. Fluorination of covalent organic framework reinforcing the confinement of Pd nanoclusters enhances hydrogen peroxide photosynthesis. J. Am. Chem. Soc. 145 , 19877–19884 (2023).
Cheng, H. et al. Rational design of covalent heptazine frameworks with spatially separated redox centers for high‐efficiency photocatalytic hydrogen peroxide production. Adv. Mater. 34 , 2107480 (2021).
Zhang, Y. et al. H 2 O 2 generation from O 2 and H 2 O on a near-infrared absorbing porphyrin supramolecular photocatalyst. Nat. Energy 8 , 361–371 (2023).
Article ADS CAS Google Scholar
Das, P., Roeser, J. & Thomas, A. Solar light driven H 2 O 2 production and selective oxidations using a covalent organic framework photocatalyst prepared by a multicomponent reaction. Angew. Chem. Int. Ed. Engl. 62 , e202304349 (2023).
Buzzetti, L., Crisenza, G. E. M. & Melchiorre, P. Mechanistic studies in photocatalysis. Angew. Chem. Int. Ed. Engl. 58 , 3730–3747 (2019).
Dias, A. M. A., Gonçalves, C. M. B., Legido, J. L., Coutinho, J. A. P. & Marrucho, I. M. Solubility of oxygen in substituted perfluorocarbons. Fluid Phase Equilib. 238 , 7–12 (2005).
Adamiak, W. et al. Hydrogen and hydrogen peroxide formation in trifluorotoluene-water biphasic systems. J. Phys. Chem. C 118 , 23154–23161 (2014).
Pang, Y., Xie, H., Sun, Y., Titirici, M.-M. & Chai, G.-L. Electrochemical oxygen reduction for H 2 O 2 production: catalysts, pH effects and mechanisms. J. Mater. Chem. A 8 , 24996–25016 (2020).
Luo, Y. et al. Sulfone‐modified covalent organic frameworks enabling efficient photocatalytic hydrogen peroxide generation via one‐step two‐electron O 2 reduction. Angew. Chem. Int. Ed. Engl. 62 , e202305355 (2023).
Liu, Y. et al. Substoichiometric covalent organic frameworks with uncondensed aldehyde for highly efficient hydrogen peroxide photosynthesis in pure water. Appl. Catal. B 331 , 122691 (2023).
Qin, C. et al. Dual donor-acceptor covalent organic frameworks for hydrogen peroxide photosynthesis. Nat. Commun. 14 , 5238 (2023).
Hao, F. et al. Photo‐driven quasi‐topological transformation exposing highly active nitrogen cation sites for enhanced photocatalytic H 2 O 2 production. Angew. Chem. Int. Ed. Engl. 62 , e202315456 (2023).
Mou, Y. et al. Linkage microenvironment of azoles‐related covalent organic frameworks precisely regulates photocatalytic generation of hydrogen peroxide. Angew. Chem. Int. Ed. Engl. 62 , e202309480 (2023).
Chen, D. et al. Covalent organic frameworks containing dual O 2 reduction centers for overall photosynthetic hydrogen peroxide production. Angew. Chem. Int. Ed. Engl. 62 , e202217479 (2023).
Yaseen, D. A. & Scholz, M. Textile dye wastewater characteristics and constituents of synthetic effluents: a critical review. Int. J. Environ. Sci. Technol. 16 , 1193–1226 (2019).
Zhao, H. et al. Rational design of carbon nitride for remarkable photocatalytic H 2 O 2 production. Chem. Catal. 2 , 1720–1733 (2022).
Chi, M. et al. Spatial decoupling of bromide-mediated process boosts propylene oxide electrosynthesis. Nat. Commun. 15 , 3646 (2024).
Download references
Acknowledgements
We acknowledge the financial support from National Natural Science Foundation of China (U2002213 and 52161160331), the Natural Science Foundation of Jiangsu Province (BK20220027), the Science and Technology Development Fund Macau SAR (0077/2021/A2), the Natural Science Foundation of the Jiangsu Higher Education Institutions of China (20KJA430002), and the Collaborative Innovation Center of Suzhou Nano Science and Technology. We thank Zihui Han and Prof. Liang Cheng for the antibacterial tests. We thank Yuhang Wang for techno-economic analysis.
Author information
Authors and affiliations.
Institute of Functional Nano & Soft Materials (FUNSOM), Soochow University, 215123, Suzhou, China
Chaochen Shao, Xiaohan Yu, Yujin Ji, Yuchen Yan, Yongpan Hu, Youyong Li, Wei Huang & Yanguang Li
Jiangsu Key Laboratory of Advanced Negative Carbon Technologies, Soochow University, 215123, Suzhou, China
College of Chemistry and Materials Engineering, Wenzhou University, 325035, Wenzhou, Zhejiang, China
Macao Institute of Materials Science and Engineering (MIMSE), MUST-SUDA Joint Research Center for Advanced Functional Materials, Macau University of Science and Technology, 999078, Macao, China
Yanguang Li
You can also search for this author in PubMed Google Scholar
Contributions
W.H. and Y.G.L. conceived the project and designed the experiments. C.S. synthesized the catalysts and performed the structural characterizations and photocatalytic tests. C.S. and X.Y. carried out the long-time performance evaluation. Y.J. and Y.Y.L. conducted the structural simulations. J.X. and Y.Y. performed the HR-TEM imaging and EDS mapping. Y.H. assisted on the quantification of H 2 O 2 . C.S., W.H., and Y.G.L. co-wrote the manuscript with contributions from all co-authors.
Corresponding authors
Correspondence to Wei Huang or Yanguang Li .
Ethics declarations
Competing interests.
The authors declare no competing interests.
Peer review
Peer review information.
Nature Communications thanks Pradip Pachfule, Hai-Long Jiang and Zhuofeng Hu, who co-reviewed with Jimmy Yu, for their contribution to the peer review of this work. A peer review file is available.
Additional information
Publisher’s note Springer Nature remains neutral with regard to jurisdictional claims in published maps and institutional affiliations.
Supplementary information
Supplementary information, peer review file, source data, source data, rights and permissions.
Open Access This article is licensed under a Creative Commons Attribution-NonCommercial-NoDerivatives 4.0 International License, which permits any non-commercial use, sharing, distribution and reproduction in any medium or format, as long as you give appropriate credit to the original author(s) and the source, provide a link to the Creative Commons licence, and indicate if you modified the licensed material. You do not have permission under this licence to share adapted material derived from this article or parts of it. The images or other third party material in this article are included in the article’s Creative Commons licence, unless indicated otherwise in a credit line to the material. If material is not included in the article’s Creative Commons licence and your intended use is not permitted by statutory regulation or exceeds the permitted use, you will need to obtain permission directly from the copyright holder. To view a copy of this licence, visit http://creativecommons.org/licenses/by-nc-nd/4.0/ .
Reprints and permissions
About this article
Cite this article.
Shao, C., Yu, X., Ji, Y. et al. Perfluoroalkyl-modified covalent organic frameworks for continuous photocatalytic hydrogen peroxide synthesis and extraction in a biphasic fluid system. Nat Commun 15 , 8023 (2024). https://doi.org/10.1038/s41467-024-52405-3
Download citation
Received : 20 April 2024
Accepted : 05 September 2024
Published : 13 September 2024
DOI : https://doi.org/10.1038/s41467-024-52405-3
Share this article
Anyone you share the following link with will be able to read this content:
Sorry, a shareable link is not currently available for this article.
Provided by the Springer Nature SharedIt content-sharing initiative
By submitting a comment you agree to abide by our Terms and Community Guidelines . If you find something abusive or that does not comply with our terms or guidelines please flag it as inappropriate.
Quick links
- Explore articles by subject
- Guide to authors
- Editorial policies
Sign up for the Nature Briefing newsletter — what matters in science, free to your inbox daily.

Photo-electrochemical sensor based on BiOI/ZnIn 2 S 4 heterojunction for detecting hydrogen peroxide and dopamine
- Brief Report
- Published: 11 September 2024
- Volume 191 , article number 590 , ( 2024 )
Cite this article
- Liangliang Qie 1 na1 ,
- Ye Li 2 na1 ,
- Weichang Li 2 na1 ,
- Yu Ding 1 , 4 na1 ,
- Chunsheng Li 2 na1 ,
- Yan Sun 2 na1 &
- Huimin Wu 3 na1
19 Accesses
Explore all metrics
Photoelectrochemical (PEC) detection as a potential development strategy for hydrogen peroxide and dopamine sensors has received extensive attentions. Herein, BiOI/ZnIn 2 S 4 -X (X = n (BiOI)/n(ZnIn 2 S 4 )) heterojunction was synthesized using various molar ratios via a two-step method. A series of characterization techniques were employed to analyze the composition, surface structure, valence state, and optical properties of BiOI/ZnIn 2 S 4 -X. The results show that BiOI/ZnIn 2 S 4 -X perform significantly better than both BiOI and ZnIn 2 S 4 . Furthermore, BiOI/ZnIn 2 S 4 -9% exhibits superior visible light absorption capacity and photocurrent response among all of the BiOI/ZnIn 2 S 4 -X tested. Therefore, a PEC sensor was developed using BiOI/ZnIn 2 S 4 -9% for the detection of hydrogen peroxide and dopamine. The linear detection range for hydrogen peroxide spans from to 1 ~ 40,000 µM, with the LOD of 0.036 µM (S/N = 3). For dopamine, the corresponding values are 2 ~ 250 µM for the linear detection range, and 0.017 μM for the LOD, respectively. The sensor exhibits demonstrates excellent stability, reproducibility and resistance to interference, enabling the detection of real samples and thus holds promising application potential.
This is a preview of subscription content, log in via an institution to check access.
Access this article
Subscribe and save.
- Get 10 units per month
- Download Article/Chapter or eBook
- 1 Unit = 1 Article or 1 Chapter
- Cancel anytime
Price includes VAT (Russian Federation)
Instant access to the full article PDF.
Rent this article via DeepDyve
Institutional subscriptions

Similar content being viewed by others

Ultrasensitive and specific photoelectrochemical sensor for hydrogen peroxide detection based on pillar[5]arene-functionalized Au nanoparticles and MWNTs hybrid BiOBr heterojunction
Non-enzymatic photoelectrochemical sensing of hydrogen peroxide using hierarchically structured zinc oxide hybridized with graphite-like carbon nitride.
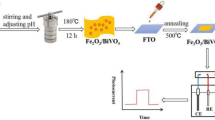
A hydrogen sulfide photoelectrochemical sensor based on BiVO 4 /Fe 2 O 3 heterojunction
Data availability.
No datasets were generated or analysed during the current study.
Li Q, Wang X, Cui X, Liu X, Wang W (2024) 3D/1D heterostructure of p-BiOBr nanoflowers modified n-TiO 2 nanorod arrays for highly sensitive photoelectrochemical determination of hydrogen peroxide. J Alloy Compd 970:172605. https://doi.org/10.1016/j.jallcom.2023.172605
Article CAS Google Scholar
Zhang T, Zheng B, Li L, Song J, Song L, Zhang M (2021) Fewer-layer conductive metal-organic Langmuir-Blodgett films as electrocatalysts enable an ultralow detection limit of H 2 O 2 . Appl Surf Sci 539:148255. https://doi.org/10.1016/j.apsusc.2020.148255
Wu W, Li Y, Jin J, Wu H, Wang S, Xia Q (2016) A novel nonenzymatic electrochemical sensor based on 3D flower-like Ni 7 S 6 for hydrogen peroxide and glucose. Sens Actuators, B Chem 232:633–641. https://doi.org/10.1016/j.snb.2016.04.006
Cheng Y, Chen C, Hu S, Liu X, Zhang L, Huang W, Song S, Xia L (2020) A facile photoelectrochemical sensor for high sensitive dopamine and ascorbic acid detection based on bi surface plasmon resonance-promoted BiVO 4 microspheres. J Electrochem Soc 167:027536. https://doi.org/10.1149/1945-7111/ab6cf3
Dashtian K, Hajati S, Ghaedi M (2022) Molecular imprinted poly(2,5-benzimidazole)-modified VO 2 –CuWO 4 homotype heterojunction for photoelectrochemical dopamine sensing. Anal Chem 94:6781–6790. https://doi.org/10.1021/acs.analchem.2c00485
Article CAS PubMed Google Scholar
Xiao F, Wang Y, Li Q, Yang D, Yang Y (2023) Fluorescence detection of dopamine based on the peroxidase-like activity of Fe 3 O 4 -MWCNTs@Hemin. Microchim Acta 190:259–270. https://doi.org/10.1007/s00604-023-05796-x
Wu W, Chang H, Tsai Y (2011) Electrocatalytic detection of dopamine in the presence of ascorbic acid and uric acid at silicon carbide coated electrodes. Chem Commun 47:6458–6460. https://doi.org/10.1039/C1CC11162K
Sun F, Zeng J, Jing M, Zhou J, Feng J, Owen SF, Luo Y, Li F, Wang H, Yamaguchi T, Yong Z, Gao Y, Peng W, Wang L, Zhang S, Du J, Lin D, Xu M, Kreitzer AC, Cui G, Li (2018) A Genetically Encoded Fluorescent Sensor Enables Rapid and Specific Detection of Dopamine in Flies, Fish, and Mice. Cell 174:481–496. https://doi.org/10.1016/j.cell.2018.06.042
Article CAS PubMed PubMed Central Google Scholar
Pradhan T, Jung HS, Jang JH, Kim TW, Kang C, Kim JS (2023) Chemical sensing of neurotransmitters. Chem Soc Rev 43:4684–4713. https://doi.org/10.1039/C3CS60477B
Article Google Scholar
Zhang L, Feng L, Li P, Chen X, Xu C, Zhang S, Zhang A, Chen G, Wang H (2020) Near-infrared light-driven photoelectrochemical sensor for mercury (II) detection using bead-chain-like Ag@Ag 2 S nanocomposites. Chem Eng J 409:128154. https://doi.org/10.1016/j.cej.2020.128154
Li M, Wang J, Zhou Y, Chen Y, Xia L (2024) Photoelectrochemical biosensor with Au@PTCA Schottky junction and multiple sandwich structures for Hg 2+ sensitive detection. Microchem Jo 196:109609. https://doi.org/10.1016/j.microc.2023.109609
Shan H-Y, Shen K-Y, Song S-S, Chen C, Ruan F-Y, Wang A-J, Yuan P-X, Feng J-J (2023) In situ synthesis of Au nanoparticles confined in 2D sheet-like metal organic frameworks to construct competitive PEC biosensor for sensitive analysis of autism spectrum disorder miRNA. Sens Actuators, B Chem 375:132849. https://doi.org/10.1016/j.snb.2022.132849
Sreedhar A, Reddy IN, Hoai Ta QT, Phuong Doan TH, Shim J, Noh J-S (2020) Unveiling the impact of interfacially engineered selective V 2 O 5 nanobelt bundles with flake-like ZnO and Co–ZnO thin films for multifunctional visible-light water splitting and toxic gas sensing. J Power Sources 478:229081. https://doi.org/10.1016/j.jpowsour.2020.229081
You F, Wei J, Cheng Y, Wen Z, Ding C, Hao N, Wang K (2021) Selective and sensitive photoelectrochemical aptasensor for streptomycin detection based on Bi 4 VO 8 Br/Ti 3 C 2 nanohybrids. J Hazard Mater 414:125539. https://doi.org/10.1016/j.jhazmat.2021.125539
Zhang Z, Liu Q, Zhang M, You F, Hao N, Ding C, Wang K (2021) Simultaneous detection of enrofloxacin and ciprofloxacin in milk using a bias potentials controlling-based photoelectrochemical aptasensor. J Hazard Mater 416:125988. https://doi.org/10.1016/j.jhazmat.2021.125988
Mao C, Qin Y, Zhou X, Dai R, Zhao L (2024) Late-model Bi-MOF&CdS-QDs materials: Excellent properties and performance in PEC sensors for lung cancer markers. Chem Eng J 490:151560. https://doi.org/10.1016/j.cej.2024.151560
Huang W, Cheng Y, Fei X, Xu W, Chang S, Song S, Huang C (2020) Designing of CuS growing on Bi 2 WO 6 nanosheet heterostructures based on a photoelectrochemical aptasensor for detecting ofloxacin. Microchim Acta 187:583. https://doi.org/10.1007/s00604-020-04516-z
Shao Z, Liu W, Zhang Y, Yang X, Zhong M (2021) Insights on interfacial charge transfer across MoS 2 /TiO 2 -NTAs nanoheterostructures for enhanced photodegradation and biosensing&gas-sensing performance. J Mol Struct 1244:131240. https://doi.org/10.1016/j.molstruc.2021.131240
Li Q, Yuan B, Zhang B, Ji Y, Xie H, Xie Y, Dong Y, Liu Z, Liu Y, Qiao L, Ke R, Yang C, Han J, He W (2023) Bi-functional Ni 3 S 2 @MoS 2 heterostructure with strong built-in field as highly-efficient electrolytic catalyst. J Electroanal Chem 931:117185. https://doi.org/10.1016/j.jelechem.2023.117185
Alhammadi S, Mun BG, Gedi S, Minnam Reddy VR, Rabie AM, Sayed MS, Shim J-J, Park H, Kim WK (2021) Effect of silver doping on the properties and photocatalytic performance of In 2 S 3 nanoparticles. J Mol Liq 344:117649. https://doi.org/10.1016/j.molliq.2021.117649
Qiu J, Li M, Yang L, Yao J (2019) Facile construction of three-dimensional netted ZnIn 2 S 4 by cellulose nanofibrils for efficiently photocatalytic reduction of Cr(VI). Chem Eng J 375:121990. https://doi.org/10.1016/j.cej.2019.121990
Chen J, Shen Z, Lv S, Shen K, Wu R, Jiang X-f, Fan T, Chen J, Li Y (2018) Fabricating sandwich-shelled ZnCdS/ZnO/ZnCdS dodecahedral cages with “one stone” as Z-scheme photocatalysts for highly efficient hydrogen production. J Mater Chem A 6:19631–19642. https://doi.org/10.1039/C8TA07362G
Huang W, Li Z, Wu C, Zhang H, Sun J, Li Q (2022) Delaminating Ti 3 C 2 MXene by blossom of ZnIn 2 S 4 microflowers for noble-metal-free photocatalytic hydrogen production. J Mater Sci Technol 120:89–98. https://doi.org/10.1016/j.jmst.2021.12.028
Hou S, Xu C, Ju X, Jin Y (2022) Interfacial Assembly of Ti 3 C 2 Tx/ZnIn 2 S 4 Heterojunction for High-Performance Photodetectors. Adv Sci 9:2204687. https://doi.org/10.1002/advs.202204687
Xu A, Zhang Y, Fan H, Liu X, Wang F, Qu X, Yang L, Li X, Cao J, Wei M (2024) WO 3 Nanosheet/ZnIn 2 S 4 S-Scheme Heterojunctions for Enhanced CO 2 Photoreduction. ACS Appl Nano Mater 7:3488–3498. https://doi.org/10.1021/acsanm.4c00147
Chen L, Wang D, Huang R, Liang R, Zhang L, Ying S, Yan G (2023) MoC@NC cocatalyst-modified ZnIn 2 S 4 with strong 2D/2D hetero-interface interaction for efficient H 2 evolution. CrystEngComm 25:6310–6316. https://doi.org/10.1039/D3CE00907F
Jiang X, Fan D, Yao X, Dong Z, Li X, Ma S, Liu J, Zhang D, Li H, Pu CP (2023) Highly efficient flower-like ZnIn 2 S 4 /CoFe 2 O 4 photocatalyst with p-n type heterojunction for enhanced hydrogen evolution under visible light irradiation. J Colloid Interface Sci 641:26–35. https://doi.org/10.1016/j.jcis.2023.03.055
Wang M, Kafizas A, Sathasivam S, Blunt MO, Moss B, Gonzalez-Carrero S, Carmalt CJ (2023) ZnO/BiOI heterojunction photoanodes with enhanced photoelectrochemical water oxidation activity. Appl Catal B: Environ Energy 331:122657. https://doi.org/10.1016/j.apcatb.2023.122657
Gao H, Sun L, Li M, Zhan W, Wang X, Han X (2021) Fabricating a hollow cuboctahedral structure for N-doped carbon coated p-n heterojunctions towards high-performance photocatalytic organic transformation. Nano Res 15:4638–4645. https://doi.org/10.1007/s12274-021-4016-5
Velusamy P, Liu X, Sathiya M, Alsaiari NS, Alzahrani FM, Tariq Nazir M, Elangovan Elamurugu M, Pandian S, Zhang F (2023) Investigate the suitability of g-C 3 N 4 nanosheets ornamented with BiOI nanoflowers for photocatalytic dye degradation and PEC water splitting. Chemosphere 321:138007. https://doi.org/10.1016/j.chemosphere.2023.138007
Wang Q, Guo L, Gao W, Li S, Hao L, Wang Z, Wang C, Wu Q (2022) Facile synthesis of BiOI/MXene heterostructure as a superior photoelectrochemical sensor for sensitive detection of glucose. Anal Chim Acta 1233:340511. https://doi.org/10.1016/j.aca.2022.340511
Xu W, Tian W, Meng L, Cao F, Li L (2021) Interfacial Chemical Bond-Modulated Z-Scheme Charge Transfer for Efficient Photoelectrochemical Water Splitting. 11:2003500. https://doi.org/10.1002/aenm.202003500
Wang H, Liang Y, Liu L, Hu J, Wu P, Cui W (2017) Enriched photoelectrocatalytic degradation and photoelectric performance of BiOI photoelectrode by coupling rGO. Appl Catal B 208:22–34. https://doi.org/10.1016/j.apcatb.2017.02.055
Naranov E, Sadovnikov A, Arapova O, Kuchinskaya T, Usoltsev O, Bugaev A, Janssens K, De Vos D, Maximov A (2023) The in-situ formation of supported hydrous ruthenium oxide in aqueous phase during HDO of lignin-derived fractions. Appl Catal B: Environ Energy 334:122861. https://doi.org/10.1016/j.apcatb.2023.122861
Cheng D, Wu H, Feng C, Zhang Y, Ding Y, Mei H (2021) Highly sensitive detection of chromium (VI) by photoelectrochemical sensor based on p-n heterojunction of carbon nitride-modified BiOI. J Alloy Compd 882:160690. https://doi.org/10.1016/j.jallcom.2021.160690
Qiao J, Wang Y, Dong S, Liang Q, Shao S (2023) Au Nanoparticles-Decorated BiOI Nanosheet Arrays for Cathodic Photoelectrochemical Dopamine Sensors. ACS Appl Nano Mater 6:5664–5674. https://doi.org/10.1021/acsanm.3c00111
Liu Z, Wang Q, Tan X, Wang Y, Jin R, Gao S (2019) Enhanced photocatalytic performance of TiO 2 NTs decorated with chrysanthemum-like BiOI nanoflowers. Sep Purif Technol 215:565–572. https://doi.org/10.1016/j.seppur.2019.01.046
Du C, Zhang Q, Lin Z, Yan B, Xia C, Yang G (2019) Half-unit-cell ZnIn 2 S 4 monolayer with sulfur vacancies for photocatalytic hydrogen evolution. Appl Catal B 248:193–201. https://doi.org/10.1016/j.apcatb.2019.02.027
Yang W, Liu B, Fang T, Jennifer W-A, Christophe L, Li Z, Zhang X, Jiang X (2016) Layered crystalline ZnIn 2 S 4 nanosheets: CVD synthesis and photo-electrochemical properties. Nanoscale 8:18197–18203. https://doi.org/10.1039/C6NR06969J
Tu X, Lu J, Li M, Su Y, Yin G, He D (2018) Hierarchically ZnIn 2 S 4 nanosheet-constructed microwire arrays: template-free synthesis and excellent photocatalytic performances. Nanoscale 10:4735–4744. https://doi.org/10.1039/C7NR09413B
Li J, Wu C, Li J, Dong B, Zhao L, Wang S (2022) 1D/2D TiO 2 /ZnIn 2 S 4 S-scheme heterojunction photocatalyst for efficient hydrogen evolution. Chin J Catal 43:339–349. https://doi.org/10.1016/S1872-2067(21)63875-5
Hou J, Jiang T, Wang X, Zhang G, Zou J, Cao C (2021) Variable dimensional structure and interface design of g-C 3 N 4 /BiOI composites with oxygen vacancy for improving visible-light photocatalytic properties. J Clean Prod 287:125072. https://doi.org/10.1016/j.jclepro.2020.125072
Luo L, Zhong J, Li J (2022) Photocatalytic property of MWCNTs/BiOI with rich oxygen vacancies. Mater Res Bull 150:111763. https://doi.org/10.1016/j.materresbull.2022.111763
Sowik J, Grzyb T, Trykowski G, Klimczuk T, Nikiforow K, Cavdar O, Zaleska-Medynska A, Malankowska A (2022) Lanthanide-organic-frameworks modified ZnIn 2 S 4 for boosting hydrogen generation under UV–Vis and visible light. Int J Hydrogen Energy 47:16065–16079. https://doi.org/10.1016/j.ijhydene.2022.03.111
Zhu J-H, Feng Y-G, Wang A-J, Mei L-P, Luo X, Feng J-J (2021) A signal-on photoelectrochemical aptasensor for chloramphenicol assay based on 3D self-supporting AgI/Ag/BiOI Z-scheme heterojunction arrays. Biosens Bioelectron 181:113158. https://doi.org/10.1016/j.bios.2021.113158
Wang Y, Liu M, Wu C, Gao J, Li M, Xing Z, Li Z, Zhou W (2022). Hollow nanoboxes Cu 2-x S@ZnIn 2 S 4 core-shell s-scheme heterojunction with broad-spectrum response and enhanced photothermal-photocatalytic performance. 18:2202544. https://doi.org/10.1002/smll.202202544
Yan P, Xu L, Cheng X, Qian J, Li H, Xia J, Zhang Q, Hua M, Li H (2017) Photoelectrochemical monitoring of phenol by metallic Bi self-doping BiOI composites with enhanced photoelectrochemical performance. J Electroanal Chem 804:64–71. https://doi.org/10.1016/j.jelechem.2017.09.003
Wu R, Fan G-C, Jiang L-P, Zhu J-J (2018) Peptide-Based Photoelectrochemical Cytosensor Using a Hollow-TiO 2 /EG/ZnIn 2 S 4 Cosensitized Structure for Ultrasensitive Detection of Early Apoptotic Cells and Drug Evaluation. ACS Appl Mater Interfaces 10:4429–4438. https://doi.org/10.1021/acsami.7b16054
Meng L, Zhang Y, Wang J, Zhou B, Xu Z, Shi J (2023) Construction of MIL-125 derived 2D TiO 2 @C nanocake/Au/3D peony-like BiOI Z-scheme heterojunction for sensitive and facile photoelectrochemical sensing assay of Cr (VI) based on competitive consumption of surface sacrificial agent. Sens Actuators, B Chem 396:134578. https://doi.org/10.1016/j.snb.2023.134578
Kumar D, Sharma S, Khare N (2021) Electric polarization tune enhanced photoelectrochemical performance of visible light active ferroelectric Bi 0.5 Na 0.5 TiO 3 nanostructure photoanode. Renewable Energy 180:186–192. https://doi.org/10.1016/j.renene.2021.08.049
Sharma S, Kumar D, Khare N (2019) Plasmonic Ag nanoparticles decorated Bi 2 S 3 nanorods and nanoflowers: Their comparative assessment for photoelectrochemical water splitting. Int J Hydrogen Energy 44:3538–3552. https://doi.org/10.1016/j.ijhydene.2018.11.238
Dong J, Shi Y, Huang C, Wu Q, Zeng T, Yao W (2018) A New and stable Mo-Mo 2 C modified g-C 3 N 4 photocatalyst for efficient visible light photocatalytic H 2 production. Appl Catal B: Environ Energy 243:27–35. https://doi.org/10.1016/j.apcatb.2018.10.016
Cheng D, Wu H, Feng C, Ding Y, Mei H (2022) Bifunctional photoelectrochemical sensor based on Bi/Bi 2 S 3 /BiVO 4 for detecting hexavalent chromium and hydrogen peroxide. Sens Actuators, B Chem 353:131108. https://doi.org/10.1016/j.snb.2021.131108
Sun Z, Sun S, Jiang X, Ai Y, Xu W, Xie L, Sun H-b, Liang Q (2022) Oligo-layer graphene stabilized fully exposed Fe-sites for ultra-sensitivity electrochemical detection of dopamine. Biosens Bioelectron 211:114367. https://doi.org/10.1016/j.bios.2022.114367
Song Y, Wang C, Sha J, Liu X, Han L, Li L (2023) Photoelectrochemical sensor based on the sensitive interface of photosensitive electrode for the detection of hydrogen peroxide in dried bean curds. J Food Compos Anal 119:105237. https://doi.org/10.1016/j.jfca.2023.105237
Tong M, Zhang N, Tan Z, Chi L, Zhang K, Chen B, Hu F, Guo C (2023) Oxygen vacancy-rich Bi 2 WO 6 nanocrystals for fast and wide-range photoelectrochemical sensing of hydrogen peroxide. Microchem J 190:108618. https://doi.org/10.1016/j.microc.2023.108618
Xiang G, He X, Liu Y, Lian H, Zhang C, Peng J (2022) Quinoxaline-based conjugated microporous polymer photoelectrochemical sensor for the sensitive detection of dopamine. J Electrochem Soc 169:127505. https://doi.org/10.1149/1945-7111/aca563
Wang Z, Lin Z, Shen S, Zhong W, Cao S (2021) Advances in designing heterojunction photocatalytic materials. Chin J Catal 42:710–730. https://doi.org/10.1016/S1872-2067(20)63698-1
Yu J, Wang W, Cheng B (2010) Synthesis and enhanced photocatalytic activity of a hierarchical porous flowerlike p–n junction NiO/TiO 2 photocatalyst. Chem Asian J 5:2499–2506. https://doi.org/10.1002/asia.201000550
Choi DS, Lee H, Tieves F, Lee YW, Son EJ, Zhang W, Shin B, Hollmann F, Park CB (2019) Bias-free in situ H 2 O 2 generation in a photovoltaic-photoelectrochemical tandem cell for biocatalytic oxyfunctionalization. ACS Catal 9:10562–10566. https://doi.org/10.1021/acscatal.9b04454
Zhu M, Chen H, Dai Y, Wu X, Han Z, Zhu Y (2021) Novel n-p-n heterojunction of AgI/BiOI/UiO-66 composites with boosting visible light photocatalytic activities. 35:e6186. https://doi.org/10.1002/aoc.6186
Qian J, Liu Y, Cui H, You F, Yang H, Wang K, Wei J, Long L, Wang C (2024) Incorporation of ZnIn 2 S 4 semiconductors with S-vacancy engineered MoS 2 nanosheets to develop sensitive photoelectrochemical aptasensor for aflatoxin B1 detection. Sensors Actuators B Chem 403:135195. https://doi.org/10.1016/j.snb.2023.135195
Zhang J, Gao Y, Liu P, Yan J, Zhang X, Xing Y, Song W (2021) Charge transfer accelerated by internal electric field of MoS 2 QDs-BiOI p-n heterojunction for high performance cathodic PEC aptasensing. Electrochim Acta 365:137392. https://doi.org/10.1016/j.electacta.2020.137392
Yang Z, Wang Y, Zhang D, Chen C (2018) A sensitizing photoelectrochemical sensing platform strategy based on bio-etching preparation of Bi 2 S 3 /BiOCl p–n heterojunction. Talanta 190:357–362. https://doi.org/10.1016/j.talanta.2018.08.004
Shang X, Weng Q, Wang F, Wang J, Huang S, Chen S, Han Z, Chen J (2021) Non-enzymatic photoelectrochemical sensors based on Schiff base and chitosan co-decorated TiO 2 nanosheets for dopamine detection. J Photochem Photobiol, A 418:113426. https://doi.org/10.1016/j.jphotochem.2021.113426
Zhang L-X, Feng L-P, Li P, Chen X, Jiang J-T, Zhang S, Zhang C-X (2020) Direct Zscheme photocatalyst of hollow CoSx@CdS polyhedron constructed by ZIF-67-templated one-pot solvothermal route: A signal-on photoelectrochemical sensor for mercury (II). Chem Eng J 395:125072. https://doi.org/10.1016/j.cej.2020.125072
Du H, Xue Y, Wang C, Jie G (2022) ZnIn 2 S 4 QDs@TiO 2 nanosphere-BiOI double heterojunction combined with unique tripod DNA walker amplification for photoelectrochemical biosensing of microRNA-21. Sens Actuators, B Chem 373:132704. https://doi.org/10.1016/j.snb.2022.132704
Shao Z, Zhang Y, Yang X, Yu G, Song Y, Zhong M, Xiu X (2024) Insights on Z-scheme interfacial charge transfer of TiO 2 -NRAs/BiOI-NFs/Au-NPs nanoheterostructures and unveiling enhanced photoelectrochemical performances. Arab J Chem 17:105765. https://doi.org/10.1016/j.arabjc.2024.105765
Wang T, Zhao C, Meng L, Li Y, Chu H, Wang F, Tao Y, Liu W, Wang C-C (2023) In-situ-construction of BiOI/UiO-66 heterostructure via nanoplate-on-octahedron: a novel p-n heterojunction photocatalyst for efficient sulfadiazine elimination. Chem Eng J 451:138624. https://doi.org/10.1016/j.cej.2022.138624
Download references
This work was financially supported by Hubei Key Laboratory for Processing and Application of Catalytic Materials (202023704), and the Science and Technology Projects of Suzhou City (SYC2022043).
Author information
Liangliang Qie and Ye Li contributed equally to this work and should be considered co-first authors.
Authors and Affiliations
School of Chemistry and Materials Science, Hubei Engineering University, Xiaogan, 432000, Hubei, P. R. China
Liangliang Qie & Yu Ding
Key Laboratory of Advanced Electrode Materials for Novel Solar Cells for Petroleum and Chemical Industry of China, School of Chemistry and Life Sciences, Suzhou University of Science and Technology, Suzhou City, 215009, Jiangsu Province, P. R. China
Ye Li, Weichang Li, Chunsheng Li & Yan Sun
Hubei Key Laboratory for Precision Synthesis of Small Molecule Pharmaceuticals, College of Chemistry and Chemical Engineering, Hubei University, Wuhan, 430062, Hubei, P. R. China
Nuode New Energy Materials Research Institute, Nuode New Materials Co., Ltd, Shenzhen, 518000, P. R. China
You can also search for this author in PubMed Google Scholar
Contributions
Liangliang Qie did some physical testings and writing. Ye Li did some photo-electrochemical testings and writing. Weichang Li did some literature review. Yu Ding financial support. Chunsheng Li financial support. Yan Sun did some literature review. Huimin Wu supervisor.
Corresponding author
Correspondence to Huimin Wu .
Ethics declarations
Ethics approval.
Not applicable.
Conflict of interest
The authors declare no competing interests.
Additional information
Publisher’s note.
Springer Nature remains neutral with regard to jurisdictional claims in published maps and institutional affiliations.
Supplementary Information
Below is the link to the electronic supplementary material.
Supplementary file1 (DOCX 646 KB)
Rights and permissions.
Springer Nature or its licensor (e.g. a society or other partner) holds exclusive rights to this article under a publishing agreement with the author(s) or other rightsholder(s); author self-archiving of the accepted manuscript version of this article is solely governed by the terms of such publishing agreement and applicable law.
Reprints and permissions
About this article
Qie, L., Li, Y., Li, W. et al. Photo-electrochemical sensor based on BiOI/ZnIn 2 S 4 heterojunction for detecting hydrogen peroxide and dopamine. Microchim Acta 191 , 590 (2024). https://doi.org/10.1007/s00604-024-06659-9
Download citation
Received : 29 May 2024
Accepted : 27 August 2024
Published : 11 September 2024
DOI : https://doi.org/10.1007/s00604-024-06659-9
Share this article
Anyone you share the following link with will be able to read this content:
Sorry, a shareable link is not currently available for this article.
Provided by the Springer Nature SharedIt content-sharing initiative
- BiOI/ZnIn 2 S 4
- Photoelectrochemical sensor
- Hydrogen peroxide sensing
- Dopamine sensing
- Find a journal
- Publish with us
- Track your research

COMMENTS
1. Elephant Toothpaste. One experiment that is sure to captivate the minds of both students and teachers alike is the famous "Elephant Toothpaste" experiment using hydrogen peroxide. Elephant Toothpaste experiment is a must-try for any classroom, sparking excitement and curiosity while reinforcing fundamental chemistry principles. 2.
Hydrogen Peroxide and Yeast. Hydrogen peroxide is relatively unstable, so over time it breaks down into water and oxygen. In this experiment, yeast is added to hydrogen peroxide to speed up its decomposition process, which is normally slow. You can perform the experiment at home in a sink. You'll need an empty large soda bottle, 3 percent ...
Gather Materials: Collect all the necessary materials - a clear jar or cup, 3% hydrogen peroxide, food coloring (optional), dish soap, a favorite fragrance (optional), yeast, warm water, a mixing spoon, and a whisk. Set Up the Jar/Cup: Pour 3% hydrogen peroxide into the clear jar or cup. Add a few drops of dish soap for a colorful effect.
The demonstration. Place about 1 cm 3 of washing up liquid into each of the measuring cylinders. To each one add the amount of catalyst specified above. Then add 25 cm 3 of 50 volume hydrogen peroxide solution to each cylinder. The addition of the catalyst to each cylinder should be done as nearly simultaneously as possible - using two ...
The basis for the genie in the bottle reaction is the decomposition of hydrogen peroxide. But, you need a more concentrated solution that household peroxide. Get the 30% peroxide solution from a beauty supply store, online, or a chemical supply company. Bottle; 30 to 50 milliliter 30% hydrogen peroxide (H 2 O 2)
Instructions. Pour 1/2 cup of hydrogen peroxide into the bottle. Add a big squirt of dish soap into the bottle, and swirl gently to mix. If you want to make your foam a single color, add a few drops of food coloring directly into the hydrogen peroxide, and swirl the bottle gently to mix. If you want to give your foam stripes like some ...
The basis for the elephant toothpaste display is the rapid decomposition of hydrogen peroxide (H 2 O 2). Hydrogen peroxide naturally decomposes into water and oxygen gas according to this chemical reaction: 2H 2 O 2 (l) → 2H 2 O(l) + O 2 (g) In a decomposition reaction, a larger molecule breaks down into two or more smaller molecules. The ...
Figure 1. When blended liver is exposed to hydrogen peroxide, the catalase enzyme in the liver reacts with the hydrogen peroxide to form oxygen gas, which creates the visible bubbles in this photograph, and water. In this science fair project, you will use fresh (or frozen) liver as a source of catalase and investigate how the activity of the ...
In this catalase and hydrogen peroxide experiment, we will discover how enzymes act as catalysts by causing chemical reactions to occur more quickly within living things. Using a potato and hydrogen peroxide, we can observe how enzymes like catalase work to perform decomposition, or the breaking down, of other substances. Catalase works to ...
Step 4: Mix together the yeast and warm water. Add yeast and warm water to a measuring cup. Stir with a fork to combine, or use a whisk. The yeast will clump up, so either keep stirring until it dissolves more into the water, or let it sit for a couple of minutes before stirring again.
Use a funnel to carefully pour 3/4 cup (180 ml) of the hydrogen peroxide liquid into the bottle. Add about 10 drops of your favorite food coloring into the bottle. Add about 1 tablespoon (15ml) of liquid dish soap into the bottle and swish the bottle around a bit to mix it. In a separate small cup, combine the warm water and the yeast together ...
Elephant Toothpaste Science. The main reactor in this experiment is 3% Hydrogen Peroxide a liquid made from hydrogen atoms and oxygen atoms (chemical formula H 2 O 2), which is used in first aid as an antiseptic.Normally it comes in a dark bottle because H 2 O 2 or hydrogen peroxide breaks down in light, turning into oxygen (O 2) and water (H 2 O). Normally this reaction happens very slowly ...
Pour 1 tablespoon of hydrogen peroxide into a cup. Place the thermometer into the cup. Hold the thermometer and the cup so they do not fall over. Read the temperature and write it down as your "Starting Temperature". Measure 1 teaspoon of yeast. While the thermometer is still in the cup, dump all the yeast into the cup.
Using hydrogen peroxide and yeast (which contains catalase), students are guided in a hands-on experiment with a control and sample solutions containing varying amounts of hydrogen peroxide. When hydrogen peroxide breaks down into oxygen and water, you can see the release of oxygen in the formation of bubbles (or foam).
Bottle 6 is for the 6% hydrogen peroxide and bottle 3 is for the 3% hydrogen peroxide. Add ½ cup of the appropriate type of hydrogen peroxide to each empty bottle. Add a squirt of dishwashing soap to each bottle. Add 4 drops of food coloring to each. In 2 small dishes, add to each 1 teaspoon of yeast to 2 tablespoons of warm water.
liquid dishwashing detergent. red or orange food coloring. baking soda. vinegar. Pour warm water into the volcano until it is 1/2 to 3/4 of the way full. Add several drops of food coloring. Add a squirt of detergent. This helps the 'lava' foam up and flow. Add a couple of spoonfuls of baking soda.
Foam is fun! Check out this video to see our Fantastic Foamy Fountain in action. The experiment uses Hydrogen peroxide and dry yeast. Hydrogen peroxide is similar to water but it has an extra oxygen atom. This makes it more dangerous and only adults should handle the hydrogen peroxide. When you add the yeast, it acts as a catalyst (a helper) to ...
Procedure. Measure 1/2 cup of hydrogen peroxide, and carefully pour it into the bottle. Add a big squirt of dish soap into the bottle, and swirl gently to mix. If you want to make your foam a ...
Elephant Toothpaste Experiment Instructions. Step 1 - Combine two tablespoons of warm water with one teaspoon of yeast and mix until the yeast is completely dissolved in the water. Step 2 - Pour 1/2 cup hydrogen peroxide into the empty bottle. You'll see here that we have two bottles. The bottle pictured on the left is being filled with 3 ...
Hydrogen peroxide's chemical formula is H 2 O 2. H 2 O is liquid water, and O 2 is oxygen gas. In this experiment, we observed the chemical decomposition of hydrogen peroxide. Chemical decomposition is the separation of a chemical compound into elements or simpler compounds. In this case, hydrogen peroxide decomposed into water and oxygen.
Science Interpreter Al explains chemical decomposition. Don't try this at home. You will find out what happens to hydrogen peroxide when it comes in contact with light! You will explore how things break down in decomposition reactions, and how we can speed up those reactions using a catalyst.
Add 1 teaspoon of yeast to both each of the graduated cylinders. Shake, spin, and twirl the graduated cylinders to mix up the baking soda and yeast combination. Add a generous splash of hydrogen peroxide to one of the graduated cylinders. In the other graduated cylinder, pour in a generous amount of white vinegar.
Hydrogen peroxide normally decomposes (breaks down into separate elements), and combining it with detergent and yeast (a catalyst) speeds up the process. As the oxygen emanates from the reaction, it creates bubbles. The detergent speeds up the foaming. The reaction is "exothermic," meaning it produces heat as well as steam.
Control experiments show that H 2 O 2 is predominantly produced through the 2e ... G. Hydrogen Peroxide Price Trend, Monitor, ... Science 366, 226-231 (2019). ...
Research efforts worldwide have focused on high test peroxide (HTP, >85 wt% H 2 O 2), recognized as a promising oxidizer candidate for low-toxicity hypergolic propellants due to its excellent performance, safe handling and environmental friendliness.The use of HTP in combination with various fuels, such as hydrocarbons, amines, alcohols, ionic liquids and ethers, as well as with catalytic and ...
Cao et al. found that the mobile signal is hydrogen peroxide produced by NADPH oxidase. In a concentration-dependent manner, the peroxide is sufficient to oxidize a cysteine residue in the transcription factor CCA1 HIKING EXPEDITION (CHE). Sulfenylation of CHE allows the transcription factor to bind to the promoter of the SA-biosynthesis gene.
Hydrogen peroxide (H 2 O 2) is a catalytic byproduct produced by many oxidases, and an excess of H 2 O 2 can lead to damage DNA or proteins [].Meanwhile, H 2 O 2 is extensively utilized as an oxidizing agent in various industries, including food processing, environmental protection, life sciences, and pharmaceuticals [].H 2 O 2 involved in the metabolic process will produce hydroxyl free ...